2.1: Classification and Properties of Matter
- Page ID
- 3551
\( \newcommand{\vecs}[1]{\overset { \scriptstyle \rightharpoonup} {\mathbf{#1}} } \)
\( \newcommand{\vecd}[1]{\overset{-\!-\!\rightharpoonup}{\vphantom{a}\smash {#1}}} \)
\( \newcommand{\id}{\mathrm{id}}\) \( \newcommand{\Span}{\mathrm{span}}\)
( \newcommand{\kernel}{\mathrm{null}\,}\) \( \newcommand{\range}{\mathrm{range}\,}\)
\( \newcommand{\RealPart}{\mathrm{Re}}\) \( \newcommand{\ImaginaryPart}{\mathrm{Im}}\)
\( \newcommand{\Argument}{\mathrm{Arg}}\) \( \newcommand{\norm}[1]{\| #1 \|}\)
\( \newcommand{\inner}[2]{\langle #1, #2 \rangle}\)
\( \newcommand{\Span}{\mathrm{span}}\)
\( \newcommand{\id}{\mathrm{id}}\)
\( \newcommand{\Span}{\mathrm{span}}\)
\( \newcommand{\kernel}{\mathrm{null}\,}\)
\( \newcommand{\range}{\mathrm{range}\,}\)
\( \newcommand{\RealPart}{\mathrm{Re}}\)
\( \newcommand{\ImaginaryPart}{\mathrm{Im}}\)
\( \newcommand{\Argument}{\mathrm{Arg}}\)
\( \newcommand{\norm}[1]{\| #1 \|}\)
\( \newcommand{\inner}[2]{\langle #1, #2 \rangle}\)
\( \newcommand{\Span}{\mathrm{span}}\) \( \newcommand{\AA}{\unicode[.8,0]{x212B}}\)
\( \newcommand{\vectorA}[1]{\vec{#1}} % arrow\)
\( \newcommand{\vectorAt}[1]{\vec{\text{#1}}} % arrow\)
\( \newcommand{\vectorB}[1]{\overset { \scriptstyle \rightharpoonup} {\mathbf{#1}} } \)
\( \newcommand{\vectorC}[1]{\textbf{#1}} \)
\( \newcommand{\vectorD}[1]{\overrightarrow{#1}} \)
\( \newcommand{\vectorDt}[1]{\overrightarrow{\text{#1}}} \)
\( \newcommand{\vectE}[1]{\overset{-\!-\!\rightharpoonup}{\vphantom{a}\smash{\mathbf {#1}}}} \)
\( \newcommand{\vecs}[1]{\overset { \scriptstyle \rightharpoonup} {\mathbf{#1}} } \)
\( \newcommand{\vecd}[1]{\overset{-\!-\!\rightharpoonup}{\vphantom{a}\smash {#1}}} \)
\(\newcommand{\avec}{\mathbf a}\) \(\newcommand{\bvec}{\mathbf b}\) \(\newcommand{\cvec}{\mathbf c}\) \(\newcommand{\dvec}{\mathbf d}\) \(\newcommand{\dtil}{\widetilde{\mathbf d}}\) \(\newcommand{\evec}{\mathbf e}\) \(\newcommand{\fvec}{\mathbf f}\) \(\newcommand{\nvec}{\mathbf n}\) \(\newcommand{\pvec}{\mathbf p}\) \(\newcommand{\qvec}{\mathbf q}\) \(\newcommand{\svec}{\mathbf s}\) \(\newcommand{\tvec}{\mathbf t}\) \(\newcommand{\uvec}{\mathbf u}\) \(\newcommand{\vvec}{\mathbf v}\) \(\newcommand{\wvec}{\mathbf w}\) \(\newcommand{\xvec}{\mathbf x}\) \(\newcommand{\yvec}{\mathbf y}\) \(\newcommand{\zvec}{\mathbf z}\) \(\newcommand{\rvec}{\mathbf r}\) \(\newcommand{\mvec}{\mathbf m}\) \(\newcommand{\zerovec}{\mathbf 0}\) \(\newcommand{\onevec}{\mathbf 1}\) \(\newcommand{\real}{\mathbb R}\) \(\newcommand{\twovec}[2]{\left[\begin{array}{r}#1 \\ #2 \end{array}\right]}\) \(\newcommand{\ctwovec}[2]{\left[\begin{array}{c}#1 \\ #2 \end{array}\right]}\) \(\newcommand{\threevec}[3]{\left[\begin{array}{r}#1 \\ #2 \\ #3 \end{array}\right]}\) \(\newcommand{\cthreevec}[3]{\left[\begin{array}{c}#1 \\ #2 \\ #3 \end{array}\right]}\) \(\newcommand{\fourvec}[4]{\left[\begin{array}{r}#1 \\ #2 \\ #3 \\ #4 \end{array}\right]}\) \(\newcommand{\cfourvec}[4]{\left[\begin{array}{c}#1 \\ #2 \\ #3 \\ #4 \end{array}\right]}\) \(\newcommand{\fivevec}[5]{\left[\begin{array}{r}#1 \\ #2 \\ #3 \\ #4 \\ #5 \\ \end{array}\right]}\) \(\newcommand{\cfivevec}[5]{\left[\begin{array}{c}#1 \\ #2 \\ #3 \\ #4 \\ #5 \\ \end{array}\right]}\) \(\newcommand{\mattwo}[4]{\left[\begin{array}{rr}#1 \amp #2 \\ #3 \amp #4 \\ \end{array}\right]}\) \(\newcommand{\laspan}[1]{\text{Span}\{#1\}}\) \(\newcommand{\bcal}{\cal B}\) \(\newcommand{\ccal}{\cal C}\) \(\newcommand{\scal}{\cal S}\) \(\newcommand{\wcal}{\cal W}\) \(\newcommand{\ecal}{\cal E}\) \(\newcommand{\coords}[2]{\left\{#1\right\}_{#2}}\) \(\newcommand{\gray}[1]{\color{gray}{#1}}\) \(\newcommand{\lgray}[1]{\color{lightgray}{#1}}\) \(\newcommand{\rank}{\operatorname{rank}}\) \(\newcommand{\row}{\text{Row}}\) \(\newcommand{\col}{\text{Col}}\) \(\renewcommand{\row}{\text{Row}}\) \(\newcommand{\nul}{\text{Nul}}\) \(\newcommand{\var}{\text{Var}}\) \(\newcommand{\corr}{\text{corr}}\) \(\newcommand{\len}[1]{\left|#1\right|}\) \(\newcommand{\bbar}{\overline{\bvec}}\) \(\newcommand{\bhat}{\widehat{\bvec}}\) \(\newcommand{\bperp}{\bvec^\perp}\) \(\newcommand{\xhat}{\widehat{\xvec}}\) \(\newcommand{\vhat}{\widehat{\vvec}}\) \(\newcommand{\uhat}{\widehat{\uvec}}\) \(\newcommand{\what}{\widehat{\wvec}}\) \(\newcommand{\Sighat}{\widehat{\Sigma}}\) \(\newcommand{\lt}{<}\) \(\newcommand{\gt}{>}\) \(\newcommand{\amp}{&}\) \(\definecolor{fillinmathshade}{gray}{0.9}\)- Give examples of extensive and intensive properties of a sample of matter. Which kind of property is more useful for describing a particular kind of matter?
- Explain what distinguishes heterogeneous matter from homogeneous matter.
- Describe the following separation processes: distillation, crystallization, liquid-liquid extraction, chromatography.
- To the somewhat limited extent to which it is meaningful, classify a given property as a physical or chemical property of matter.
Matter is “anything that has mass and occupies space”, we were taught in school. True enough, but not very satisfying. A really complete answer is unfortunately beyond the scope of this course, but we will offer a hint of it in a later chapter on atomic structure. For the moment, let’s put off trying to define matter and focus on the chemist’s view: matter is what chemical substances are composed of. But what do we mean by chemical substances? How do we organize our view of matter and its properties? These very practical questions will be the subjects of this lesson.
Properties of Matter
The science of chemistry developed from observations made about the nature and behavior of different kinds of matter, which we refer to collectively as the properties of matter. The properties we refer to in this lesson are all macroscopic properties: those that can be observed in bulk matter. At the microscopic level, matter is of course characterized by its structure: the spatial arrangement of the individual atoms in a molecular unit or an extended solid. By observing a sample of matter and measuring its various properties, we gradually acquire enough information to characterize it; to distinguish it from other kinds of matter. This is the first step in the development of chemical science, in which interest is focused on specific kinds of matter and the transformations between them.
If you think about the various observable properties of matter, it will become apparent that these fall into two classes. Some properties, such as mass and volume, depend on the quantity of matter in the sample we are studying. Clearly, these properties, as important as they may be, cannot by themselves be used to characterize a kind of matter; to say that “water has a mass of 2 kg” is nonsense, although it may be quite true in a particular instance. Properties of this kind are called extensive properties of matter.
Suppose we take further measurements, and find that the same quantity of water whose mass is 2.0 kg also occupies a volume of 2.0 liters. We have measured two extensive properties (mass and volume) of the same sample of matter. This allows us to define a new quantity, the quotient m/V which defines another property of water which we call the density. Unlike the mass and the volume, which by themselves refer only to individual samples of water, the density (mass per unit volume) is a property of all samples of pure water at the same temperature. Density is an example of an intensive property of matter.
This definition of the density illustrates an important general rule: the ratio of two extensive properties is always an intensive property.
Intensive properties are extremely important, because every possible kind of matter possesses a unique set of intensive properties that distinguishes it from every other kind of matter. Some intensive properties can be determined by simple observations: color (absorption spectrum), melting point, density, solubility, acidic or alkaline nature, and density are common examples. Even more fundamental, but less directly observable, is chemical composition.
The more intensive properties we know, the more precisely we can characterize a sample of matter.
Intensive properties are extremely important, because every possible kind of matter possesses a unique set of intensive properties that distinguishes it from every other kind of matter. In other words, intensive properties serve to characterize matter. Many of the intensive properties depend on such variables as the temperature and pressure, but the ways in which these properties change with such variables can themselves be regarded as intensive properties.
Classify each of the following as an extensive or intensive property.
- The volume of beer in a mug
- The percentage of alcohol in the beer
- The number of calories of energy you derive from eating a banana
- The number of calories of energy made available to your body when you consume 10.0 g of sugar
- The mass of iron present in your blood
- The mass of iron present in 5 mL of your blood
- The electrical resistance of a piece of 22-gauge copper wire.
- The electrical resistance of a 1-km length of 22-gauge copper wire
- The pressure of air in a bicycle tire
- Answer a
-
extensive; depends on size of the mug.
- Answer b
-
intensive; same for any same-sized sample.
- Answer c
-
extensive; depends on size and sugar content of the banana.
- Answer d
-
intensive; same for any 10 g portion of sugar.
- Answer e
-
extensive; depends on volume of blood in the body.
- Answer f
-
intensive; the same for any 5 mL sample.
- Answer g
-
extensive; depends on length of the wire.
- Answer h
-
intensive; same for any 1 km length of the same wire.
- Answer i
-
pressure itself is intensive, but is also dependent on the quantity of air in the tire.
The last example shows that not everything is black or white! But we often encounter matter that is not uniform throughout, whose different parts exhibit different sets of intensive properties. This brings up another distinction that we address immediately below.
How to classify matter?
One useful way of organizing our understanding of matter is to think of a hierarchy that extends down from the most general and complex to the simplest and most fundamental. The orange-colored boxes represent the central realm of chemistry, which deals ultimately with specific chemical substances, but as a practical matter, chemical science extends both above and below this region.
Alternatively, when we are thinking about specific samples of matter, it may be more useful to re-cast our classification in two dimensions:
Notice, in the bottom line of boxes above, that "mixtures" and "pure substances" can fall into either the homogeneous or heterogeneous categories.
Homogeneous and heterogeneous: it's a matter of phases
Homogeneous matter (from the Greek homo = same) can be thought of as being uniform and continuous, whereas heterogeneous matter (hetero = different) implies non-uniformity and discontinuity. To take this further, we first need to define "uniformity" in a more precise way, and this takes us to the concept of phases.
A phase is a region of matter that possesses uniform intensive properties throughout its volume. A volume of water, a chunk of ice, a grain of sand, a piece of copper— each of these constitutes a single phase, and by the above definition, is said to be homogeneous. A sample of matter can contain more than a single phase; a cool drink with ice floating in it consists of at least two phases, the liquid and the ice. If it is a carbonated beverage, you can probably see gas bubbles in it that make up a third phase.
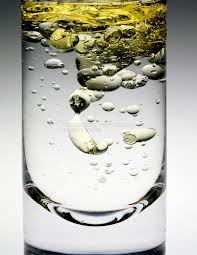
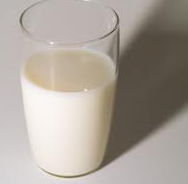
Phase boundaries
Each phase in a multiphase system is separated from its neighbors by a phase boundary, a thin region in which the intensive properties change discontinuously. Have you ever wondered why you can easily see the ice floating in a glass of water although both the water and the ice are transparent? The answer is that when light crosses a phase boundary, its direction of travel is slightly bent, and a portion of the light gets reflected back; it is these reflected and distorted light rays emerging from that reveal the chunks of ice floating in the liquid.
If, instead of visible chunks of material, the second phase is broken into tiny particles, the light rays usually bounce off the surfaces of many of these particles in random directions before they emerge from the medium and are detected by the eye. This phenomenon, known as scattering, gives multiphase systems of this kind a cloudy appearance, rendering them translucent instead of transparent. Two very common examples are ordinary fog, in which water droplets are suspended in the air, and milk, which consists of butterfat globules suspended in an aqueous solution.
Getting back to our classification, we can say that Homogeneous matter consists of a single phase throughout its volume; heterogeneous matter contains two or more phases.
Dichotomies ("either-or" classifications) often tend to break down when closely examined, and the distinction between homogeneous and heterogeneous matter is a good example; this is really a matter of degree, since at the microscopic level all matter is made up of atoms or molecules separated by empty space! For most practical purposes, we consider matter as homogeneous when any discontinuities it contains are too small to affect its visual appearance.
How large must a molecule or an agglomeration of molecules be before it begins to exhibit properties of a being a separate phase? Such particles span the gap between the micro and macro worlds, and have been known as colloids since they began to be studied around 1900. But with the development of nanotechnology in the 1990s, this distinction has become even more fuzzy.
Pure Substances and Mixtures
The air around us, most of the liquids and solids we encounter, and all too much of the water we drink consists not of pure substances, but of mixtures. You probably have a general idea of what a mixture is, and how it differs from a pure substance; what is the scientific criterion for making this distinction?
To a chemist, a pure substance usually refers to a sample of matter that has a distinct set of properties that are common to all other samples of that substance. A good example would be ordinary salt, sodium chloride. No matter what its source (from a mine, evaporated from seawater, or made in the laboratory), all samples of this substance, once they have been purified, possess the same unique set of properties.
A pure substance is one whose intensive properties are the same in any purified sample of that same substance.A mixture, in contrast, is composed of two or more substances, and it can exhibit a wide range of properties depending on the relative amounts of the components present in the mixture. For example, you can dissolve up to 357 g of salt in one litre of water at room temperature, making possible an infinite variety of "salt water" solutions. For each of these concentrations, properties such as the density, boiling and freezing points, and the vapor pressure of the resulting solution will be different.
Is anything really pure?
Those of us who enjoy peanut butter would never willingly purchase a brand advertised as "impure". But a Consumer Reports article published some years ago showed a table listing the number of "mouse droppings" and "insect parts" (presumably from peanut storage silos) they found in samples of all the major brands. Bon appetit!
Finally, we all prefer to drink "pure" water, but we don't usually concern ourselves with the dissolved atmospheric gases and ions such as Ca2+ and HCO3- that are present in most drinking waters. But these harmless "impurities" are always present in those "pure" spring waters.
and para-water, respectively.The bottom line: To a chemist, the term "pure" has meaning only in the context of a particular application or process.
Operational and conceptual classifications
Since chemistry is an experimental science, we need a set of experimental criteria for placing a given sample of matter in one of these categories. There is no single experiment that will always succeed unambiguously deciding this kind of question. However, there is one principle that will always work in theory, if not in practice. This is based on the fact that the various components of a mixture can, in principle, always be separated into pure substances.
Consider a heterogeneous mixture of salt water and sand. The sand can be separated from the salt water by the mechanical process of filtration. Similarly, the butterfat contained in milk may be separated from the water by a process known as churning, in which mechanical agitation forces the butterfat droplets to coalesce into the solid mass we know as butter. These examples illustrate the general principle that heterogeneous matter may be separated into homogeneous matter by mechanical means.
Turning this around, we have an operational definition of heterogeneous matter: If, by some mechanical operation we can separate a sample of matter into two or more other kinds of matter, then our original sample was heterogeneous. To find a similar operational definition for homogeneous mixtures, consider how we might separate the two components of a solution of salt water. The most obvious way would be to evaporate off the water, leaving the salt as a solid residue. Thus a homogeneous mixture can be separated into pure substances by undergoing appropriate partial changes of state— that is, by evaporation, freezing, etc.
Note the term partial in the above sentence; in the last example, we evaporate only the water, not the salt (which would be very difficult to do anyway!) The idea is that one component of the mixture is preferentially affected by the process we are carrying out. This principle will be emphasized in the following examples.
Separating homogeneous mixtures
Some common methods of separating homogeneous mixtures into their components are outlined below.
Distillation
A mixture of two volatile liquids is partly boiled away; the first portions of the condensed vapor will be enriched in the component having the lower boiling point. Note that if all the liquid were boiled away, the distillate would be identical with the original liquid. But if, say, half of the liquid is distilled, the distillate would contain a larger fraction of the more volatile component. If the distillate is then re-distilled, it can be further enriched in the low-boiling liquid. By repeating this process many times (aided by the fractionating column above the boiling vessel), a high degree of separation can be achieved.
Fractional crystallization
A hot saturated solution containing two or more dissolved solids is allowed to cool slowly; the least-soluble material crystallizes out first, and can be separated by filtration. This process is widely employed both in the laboratory and, on a much larger scale, in industry.
Similarly, a molten mixture of several components, when slowly cooled, will first yield crystals of the material having the highest melting point. This process occurs on a huge scale in nature when molten magma from the earth's mantle rises into the lithosphere and cools underground — a process that can take up to a million years. This is how the common rock known as granite is formed. Eventually these rocks rise and become exposed on the earth's surface.
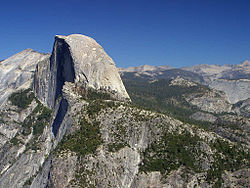
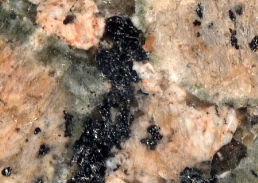
Liquid-liquid Extraction
Two mutually-insoluble liquids, one containing two or more solutes (dissolved substances), are shaken together in a separatory funnel. Each solute will concentrate in the liquid in which it is more soluble. The two solutions are then separated by opening the stopcock at the bottom, allowing the more dense solution to drain out.
Solid-liquid Extraction
In working with natural products such as plant materials, a first step is often to extract soluble substances from the plant parts. This, and similar extractions of the soluble components of complex solids, is carried out in an apparatus known as a Soxhlet extractor.
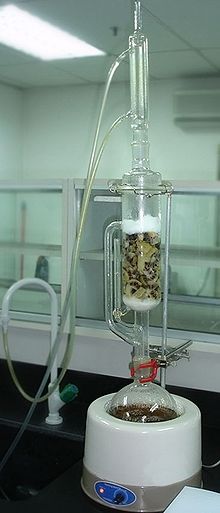
The idea is to continuously percolate an appropriate hot solvent through the material, which is contained in a porous paper "thimble". Hot vapor from the boiling flask bypasses the extraction chamber through the arm at the left (labeled "vapor" in the illustration →) and into the condenser, from which it drips down into the extraction chamber, where a portion of the soluble material mixes with the solvent. When the condensate reaches the top of the chamber, it flows out through the siphon arm, emptying its contents into the boiling flask, which becomes increasingly concentrated in the extracted material.
The advantage of this arrangement is that the percolation-and-extraction process can be repeated indefinitely (usually hours to days) without much attention.
Chromatography
As a liquid or gaseous mixture flows along a column containing an adsorbant material, the more strongly-adsorbed components tend to move more slowly and emerge later than the less-strongly adsorbed components. In this example, an extract made from plant leaves is separated into its principle components: carotene, xanthophyll, and chlorophylls A and B.
Although chromatography originated in the mid-19th Century, it was not widely employed until the 1950's. Since that time, it has encompassed a huge variety of techniques and is no longer limited to colored substances. Chromatography is now one of the most widely-employed methods for the analysis and separation of complex mixtures of liquids and gases.
Physical and Chemical Properties
Since chemistry is partly the study of the transformations that matter can undergo, we can also assign to any substance a set of chemical properties that express the various changes of composition the substance is known to undergo. Chemical properties also include the conditions of temperature, etc., required to bring about the change, and the amount of energy released or absorbed as the change takes place.
The properties that we described above are traditionally known as physical properties, and are to be distinguished from chemical properties that usually refer to changes in composition that a substance can undergo. For example, we can state some of the more distinctive physical and chemical properties of the element sodium:
Physical properties (25 °C)
|
Chemical properties
|
---|---|
|
|
The more closely one looks at the distinction between physical and chemical properties, the more blurred this distinction becomes. For example, the high boiling point of water compared to that of methane, CH4, is a consequence of the electrostatic attractions between O-H bonds in adjacent molecules, in contrast to those between C-H bonds; at this level, we are really getting into chemistry! So although you will likely be expected to "distinguish between" physical and chemical properties on an exam, don't take it too seriously — this turns out to be a rather dubious dichotomy, loved by teachers, but of limited usefulness!