2.2: Atomic Structure, Isotopes, and Atomic Mass
- Page ID
- 428683
\( \newcommand{\vecs}[1]{\overset { \scriptstyle \rightharpoonup} {\mathbf{#1}} } \)
\( \newcommand{\vecd}[1]{\overset{-\!-\!\rightharpoonup}{\vphantom{a}\smash {#1}}} \)
\( \newcommand{\id}{\mathrm{id}}\) \( \newcommand{\Span}{\mathrm{span}}\)
( \newcommand{\kernel}{\mathrm{null}\,}\) \( \newcommand{\range}{\mathrm{range}\,}\)
\( \newcommand{\RealPart}{\mathrm{Re}}\) \( \newcommand{\ImaginaryPart}{\mathrm{Im}}\)
\( \newcommand{\Argument}{\mathrm{Arg}}\) \( \newcommand{\norm}[1]{\| #1 \|}\)
\( \newcommand{\inner}[2]{\langle #1, #2 \rangle}\)
\( \newcommand{\Span}{\mathrm{span}}\)
\( \newcommand{\id}{\mathrm{id}}\)
\( \newcommand{\Span}{\mathrm{span}}\)
\( \newcommand{\kernel}{\mathrm{null}\,}\)
\( \newcommand{\range}{\mathrm{range}\,}\)
\( \newcommand{\RealPart}{\mathrm{Re}}\)
\( \newcommand{\ImaginaryPart}{\mathrm{Im}}\)
\( \newcommand{\Argument}{\mathrm{Arg}}\)
\( \newcommand{\norm}[1]{\| #1 \|}\)
\( \newcommand{\inner}[2]{\langle #1, #2 \rangle}\)
\( \newcommand{\Span}{\mathrm{span}}\) \( \newcommand{\AA}{\unicode[.8,0]{x212B}}\)
\( \newcommand{\vectorA}[1]{\vec{#1}} % arrow\)
\( \newcommand{\vectorAt}[1]{\vec{\text{#1}}} % arrow\)
\( \newcommand{\vectorB}[1]{\overset { \scriptstyle \rightharpoonup} {\mathbf{#1}} } \)
\( \newcommand{\vectorC}[1]{\textbf{#1}} \)
\( \newcommand{\vectorD}[1]{\overrightarrow{#1}} \)
\( \newcommand{\vectorDt}[1]{\overrightarrow{\text{#1}}} \)
\( \newcommand{\vectE}[1]{\overset{-\!-\!\rightharpoonup}{\vphantom{a}\smash{\mathbf {#1}}}} \)
\( \newcommand{\vecs}[1]{\overset { \scriptstyle \rightharpoonup} {\mathbf{#1}} } \)
\( \newcommand{\vecd}[1]{\overset{-\!-\!\rightharpoonup}{\vphantom{a}\smash {#1}}} \)
\(\newcommand{\avec}{\mathbf a}\) \(\newcommand{\bvec}{\mathbf b}\) \(\newcommand{\cvec}{\mathbf c}\) \(\newcommand{\dvec}{\mathbf d}\) \(\newcommand{\dtil}{\widetilde{\mathbf d}}\) \(\newcommand{\evec}{\mathbf e}\) \(\newcommand{\fvec}{\mathbf f}\) \(\newcommand{\nvec}{\mathbf n}\) \(\newcommand{\pvec}{\mathbf p}\) \(\newcommand{\qvec}{\mathbf q}\) \(\newcommand{\svec}{\mathbf s}\) \(\newcommand{\tvec}{\mathbf t}\) \(\newcommand{\uvec}{\mathbf u}\) \(\newcommand{\vvec}{\mathbf v}\) \(\newcommand{\wvec}{\mathbf w}\) \(\newcommand{\xvec}{\mathbf x}\) \(\newcommand{\yvec}{\mathbf y}\) \(\newcommand{\zvec}{\mathbf z}\) \(\newcommand{\rvec}{\mathbf r}\) \(\newcommand{\mvec}{\mathbf m}\) \(\newcommand{\zerovec}{\mathbf 0}\) \(\newcommand{\onevec}{\mathbf 1}\) \(\newcommand{\real}{\mathbb R}\) \(\newcommand{\twovec}[2]{\left[\begin{array}{r}#1 \\ #2 \end{array}\right]}\) \(\newcommand{\ctwovec}[2]{\left[\begin{array}{c}#1 \\ #2 \end{array}\right]}\) \(\newcommand{\threevec}[3]{\left[\begin{array}{r}#1 \\ #2 \\ #3 \end{array}\right]}\) \(\newcommand{\cthreevec}[3]{\left[\begin{array}{c}#1 \\ #2 \\ #3 \end{array}\right]}\) \(\newcommand{\fourvec}[4]{\left[\begin{array}{r}#1 \\ #2 \\ #3 \\ #4 \end{array}\right]}\) \(\newcommand{\cfourvec}[4]{\left[\begin{array}{c}#1 \\ #2 \\ #3 \\ #4 \end{array}\right]}\) \(\newcommand{\fivevec}[5]{\left[\begin{array}{r}#1 \\ #2 \\ #3 \\ #4 \\ #5 \\ \end{array}\right]}\) \(\newcommand{\cfivevec}[5]{\left[\begin{array}{c}#1 \\ #2 \\ #3 \\ #4 \\ #5 \\ \end{array}\right]}\) \(\newcommand{\mattwo}[4]{\left[\begin{array}{rr}#1 \amp #2 \\ #3 \amp #4 \\ \end{array}\right]}\) \(\newcommand{\laspan}[1]{\text{Span}\{#1\}}\) \(\newcommand{\bcal}{\cal B}\) \(\newcommand{\ccal}{\cal C}\) \(\newcommand{\scal}{\cal S}\) \(\newcommand{\wcal}{\cal W}\) \(\newcommand{\ecal}{\cal E}\) \(\newcommand{\coords}[2]{\left\{#1\right\}_{#2}}\) \(\newcommand{\gray}[1]{\color{gray}{#1}}\) \(\newcommand{\lgray}[1]{\color{lightgray}{#1}}\) \(\newcommand{\rank}{\operatorname{rank}}\) \(\newcommand{\row}{\text{Row}}\) \(\newcommand{\col}{\text{Col}}\) \(\renewcommand{\row}{\text{Row}}\) \(\newcommand{\nul}{\text{Nul}}\) \(\newcommand{\var}{\text{Var}}\) \(\newcommand{\corr}{\text{corr}}\) \(\newcommand{\len}[1]{\left|#1\right|}\) \(\newcommand{\bbar}{\overline{\bvec}}\) \(\newcommand{\bhat}{\widehat{\bvec}}\) \(\newcommand{\bperp}{\bvec^\perp}\) \(\newcommand{\xhat}{\widehat{\xvec}}\) \(\newcommand{\vhat}{\widehat{\vvec}}\) \(\newcommand{\uhat}{\widehat{\uvec}}\) \(\newcommand{\what}{\widehat{\wvec}}\) \(\newcommand{\Sighat}{\widehat{\Sigma}}\) \(\newcommand{\lt}{<}\) \(\newcommand{\gt}{>}\) \(\newcommand{\amp}{&}\) \(\definecolor{fillinmathshade}{gray}{0.9}\)- Write and interpret symbols that depict the atomic number, mass number, and charge of an atom or ion
- Define the unified atomic mass unit and average atomic mass
- Calculate average atomic mass and isotopic abundance
- State the periodic law and explain the organization of elements in the periodic table
- Predict the general properties of elements based on their location within the periodic table
- Identify metals, nonmetals, and metalloids by their properties and/or location on the periodic table
Structure of the Atom
The development of modern atomic theory revealed much about the inner structure of atoms. It was learned that an atom contains a very small nucleus composed of positively charged protons and uncharged neutrons, surrounded by a much larger volume of space containing negatively charged electrons. The nucleus contains the majority of an atom’s mass because protons and neutrons are much heavier than electrons, whereas electrons occupy almost all of an atom’s volume. The diameter of an atom is on the order of 10−10 m, whereas the diameter of the nucleus is roughly 10−15 m—about 100,000 times smaller. For a perspective about their relative sizes, consider this: If the nucleus were the size of a blueberry, the atom would be about the size of a football stadium (Figure \(\PageIndex{1}\)).
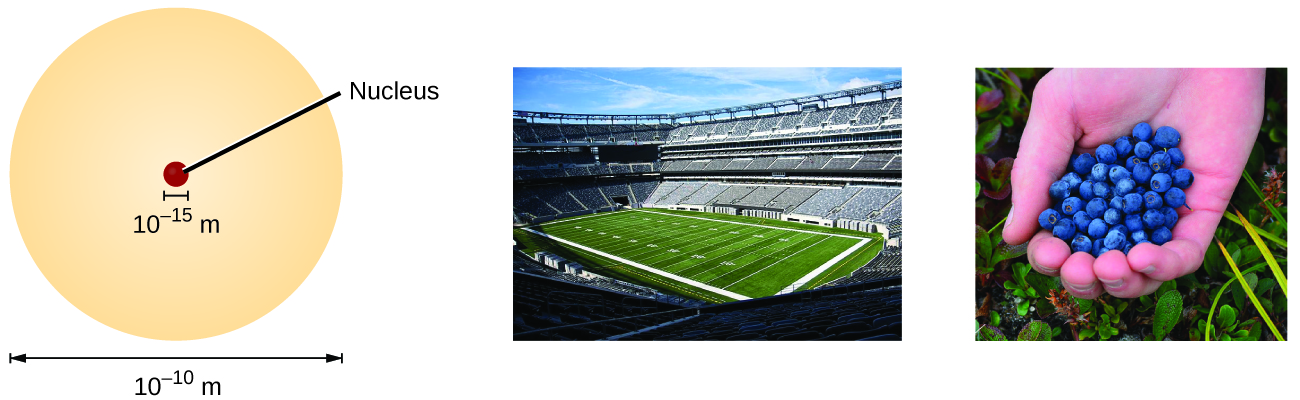
Atoms—and the protons, neutrons, and electrons that compose them—are extremely small. For example, a carbon atom weighs less than 2 \(\times\) 10−23 g, and an electron has a charge of less than 2 \(\times\) 10−19 C (coulomb). When describing the properties of tiny objects such as atoms, we use appropriately small units of measure, such as the atomic mass unit (amu) and the fundamental unit of charge (e). The atomic mass unit (amu) was originally defined based on hydrogen, the lightest element, and oxygen. Because of this ambiguity it was redefined in 1961 as the unified atomic mass unit (u) with regard to the most abundant isotope of carbon, atoms of which are assigned masses of exactly 12 u. Thus, one u is exactly \(1/12\) of the mass of one carbon-12 atom. The Dalton (Da) and the atomic mass unit (amu) are alternative units that are almost identical to the u. The fundamental unit of charge (also called the elementary charge) equals the magnitude of the charge of an electron (e) with e = 1.602 \(\times\) 10−19 C.
A proton has a mass of 1.0073 u and a charge of 1+. A neutron is a slightly heavier particle with a mass 1.0087 u and a charge of zero; as its name suggests, it is neutral. The electron has a charge of 1− and is a much lighter particle with a mass of about 0.00055 u (it would take about 1800 electrons to equal the mass of one proton. The properties of these fundamental particles are summarized in Table \(\PageIndex{1}\). You may notice the sum of an atom’s subatomic particles does not equal the atom’s actual mass: The total mass of six protons, six neutrons, and six electrons is 12.0993 u, slightly larger than the 12.00 u of an actual carbon-12 atom. This “missing” mass is known as the mass defect, it is related to the energy holding the atom together.
Name | Location | Charge (C) | Unit Charge | Mass (u) | Significance |
---|---|---|---|---|---|
electron | outside nucleus | \(−1.602 \times 10^{−19}\) | 1− | 0.00055 | charge = proton - electron |
proton | nucleus | \(1.602 \times 10^{−19}\) | 1+ | 1.00727 | atomic number = proton |
neutron | nucleus | 0 | 0 | 1.00866 | mass = proton + neutron |
The number of protons in the nucleus of an atom is its atomic number (\(Z\)). This is the defining trait of an element: Its value determines the identity of the atom. For example, any atom that contains six protons is the element carbon and has the atomic number 6, regardless of how many neutrons or electrons it may have. A neutral atom must contain the same number of positive and negative charges, so the number of protons equals the number of electrons. Therefore, the atomic number also indicates the number of electrons in an uncharged atom. The total number of protons and neutrons in an atom is called its mass number (A). The number of neutrons is therefore the difference between the mass number and the atomic number: A – Z = number of neutrons.
\[\begin{align*}
\ce{atomic\: number\:(Z)\: &= \:number\: of\: protons\\
mass\: number\:(A)\: &= \:number\: of\: protons + number\: of\: neutrons\\
A-Z\: &= \:number\: of\: neutrons}
\end{align*}\]
Atoms are electrically neutral if they contain the same number of positively charged protons and negatively charged electrons. When the numbers of these subatomic particles are not equal, the atom is electrically charged and is called an ion. The charge of an atom is defined as follows:
Atomic charge = number of protons − number of electrons
As will be discussed in more detail later in this chapter, atoms (and molecules) typically acquire charge by gaining or losing electrons. An atom that gains one or more electrons will exhibit a negative charge and is called an anion. Positively charged atoms called cations are formed when an atom loses one or more electrons. For example, a neutral sodium atom (Z = 11) has 11 electrons. If this atom loses one electron, it will become a cation with a 1+ charge (11 − 10 = 1+). A neutral oxygen atom (Z = 8) has eight electrons, and if it gains two electrons it will become an anion with a 2− charge (8 − 10 = 2−).
Iodine is an essential trace element in our diet; it is needed to produce thyroid hormone. Insufficient iodine in the diet can lead to the development of a goiter, an enlargement of the thyroid gland (Figure \(\PageIndex{2}\)).

The addition of small amounts of iodine to table salt (iodized salt) has essentially eliminated this health concern in the United States, but as much as 40% of the world’s population is still at risk of iodine deficiency. The iodine atoms are added as anions, and each has a 1− charge and a mass number of 127. Determine the numbers of protons, neutrons, and electrons in one of these iodine anions.
Solution
The atomic number of iodine (53) tells us that a neutral iodine atom contains 53 protons in its nucleus and 53 electrons outside its nucleus. Because the sum of the numbers of protons and neutrons equals the mass number, 127, the number of neutrons is 74 (127 − 53 = 74). Since the iodine is added as a 1− anion, the number of electrons is 54 [53 – (1–) = 54].
An ion of platinum has a mass number of 195 and contains 74 electrons. How many protons and neutrons does it contain, and what is its charge?
- Answer
-
78 protons; 117 neutrons; charge is 4+
Chemical Symbols
A chemical symbol is an abbreviation that we use to indicate an element or an atom of an element. For example, the symbol for mercury is Hg (Figure \(\PageIndex{3}\)). We use the same symbol to indicate one atom of mercury (microscopic domain) or to label a container of many atoms of the element mercury (macroscopic domain).
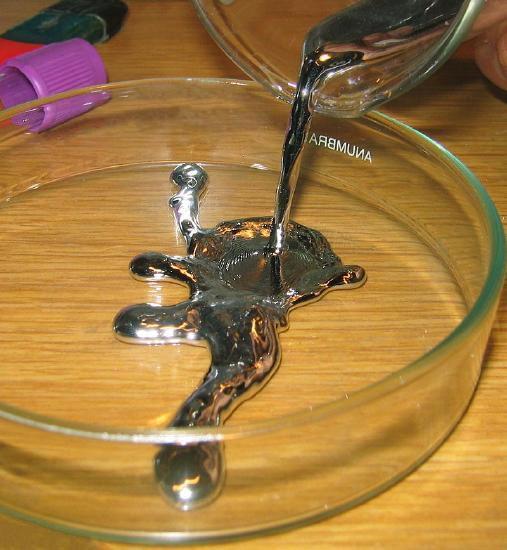
The symbols for several common elements and their atoms are listed in Table \(\PageIndex{2}\). Some symbols are derived from the common name of the element; others are abbreviations of the name in another language. Symbols have one or two letters, for example, H for hydrogen and Cl for chlorine. To avoid confusion with other notations, only the first letter of a symbol is capitalized. For example, Co is the symbol for the element cobalt, but CO is the notation for the compound carbon monoxide, which contains atoms of the elements carbon (C) and oxygen (O). All known elements and their symbols are in the periodic table.
Element | Symbol | Element | Symbol |
---|---|---|---|
aluminum | Al | iron | Fe (from ferrum) |
bromine | Br | lead | Pb (from plumbum) |
calcium | Ca | magnesium | Mg |
carbon | C | mercury | Hg (from hydrargyrum) |
copper | Cu (from cuprum) | silicon | Si |
gold | Au (from aurum) | sodium | Na (from natrium) |
Traditionally, the discoverer (or discoverers) of a new element names the element. However, until the name is recognized by the International Union of Pure and Applied Chemistry (IUPAC), the recommended name of the new element is based on the Latin word(s) for its atomic number. For example, element 106 was called unnilhexium (Unh), element 107 was called unnilseptium (Uns), and element 108 was called unniloctium (Uno) for several years. These elements are now named after scientists or locations; for example, element 106 is now known as seaborgium (Sg) in honor of Glenn Seaborg, a Nobel Prize winner who was active in the discovery of several heavy elements.
Isotopes
The symbol for a specific isotope of any element is written by placing the mass number as a superscript to the left of the element symbol (Figure \(\PageIndex{4}\)). The atomic number is sometimes written as a subscript preceding the symbol, but since this number defines the element’s identity, as does its symbol, it is often omitted. For example, magnesium exists as a mixture of three isotopes, each with an atomic number of 12 and with mass numbers of 24, 25, and 26, respectively. These isotopes can be identified as 24Mg, 25Mg, and 26Mg. These isotope symbols are read as “element, mass number” and can be symbolized consistent with this reading. For instance, 24Mg is read as “magnesium 24,” and can be written as “magnesium-24” or “Mg-24.” 25Mg is read as “magnesium 25,” and can be written as “magnesium-25” or “Mg-25.” All magnesium atoms have 12 protons in their nucleus. They differ only because a 24Mg atom has 12 neutrons in its nucleus, a 25Mg atom has 13 neutrons, and a 26Mg has 14 neutrons.
Figure \(\PageIndex{4}\) also shows how the charge of an atom is represented with the symbol for an element. In this example, the helium has a charge of +2 which is written as a superscript following the element symbol: 4He2+. The magnesium isotope has a mass of 24 and a charge of +2, so it is written as 24Mg2+.
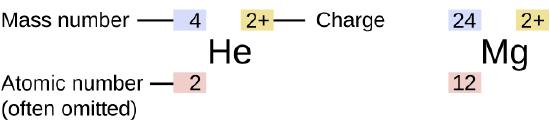
Information about the naturally occurring isotopes of elements with atomic numbers 1 through 5 is given in Table \(\PageIndex{2}\). Note that in addition to standard names and symbols, the isotopes of hydrogen are often referred to using common names and accompanying symbols. Hydrogen-2, symbolized 2H, is also called deuterium and sometimes symbolized D. Hydrogen-3, symbolized 3H, is also called tritium and sometimes symbolized T.
Element | Symbol | Atomic Number | Number of Protons | Number of Neutrons | Mass (u) | % Natural Abundance |
---|---|---|---|---|---|---|
hydrogen | \(\ce{^1_1H}\) (protium) |
1 | 1 | 0 | 1.0078 | 99.989 |
\(\ce{^2_1H}\) (deuterium) |
1 | 1 | 1 | 2.0141 | 0.0115 | |
\(\ce{^3_1H}\) (tritium) |
1 | 1 | 2 | 3.01605 | — (trace) | |
helium | \(\ce{^3_2He}\) | 2 | 2 | 1 | 3.01603 | 0.00013 |
\(\ce{^4_2He}\) | 2 | 2 | 2 | 4.0026 | 100 | |
lithium | \(\ce{^6_3Li}\) | 3 | 3 | 3 | 6.0151 | 7.59 |
\(\ce{^7_3Li}\) | 3 | 3 | 4 | 7.0160 | 92.41 | |
beryllium | \(\ce{^9_4Be}\) | 4 | 4 | 5 | 9.0122 | 100 |
boron | \(\ce{^{10}_5B}\) | 5 | 5 | 5 | 10.0129 | 19.9 |
\(\ce{^{11}_5B}\) | 5 | 5 | 6 | 11.0093 | 80.1 |
Atomic Mass and Isotopes
Because each proton and each neutron contribute approximately one u to the mass of an atom, and each electron contributes far less, the atomic mass of a single atom is approximately equal to its mass number (a whole number). However, the average masses of atoms of most elements are not whole numbers because most elements exist naturally as mixtures of two or more isotopes.
The mass of an element shown in a periodic table or listed in a table of atomic masses is a weighted, average mass of all the isotopes present in a naturally occurring sample of that element. This is equal to the sum of each individual isotope’s mass multiplied by its fractional abundance.
\[\mathrm{average\: mass}=\sum_{i}(\mathrm{fractional\: abundance\times isotopic\: mass})_i\]
For example, the element boron is composed of two isotopes: About 19.9% of all boron atoms are 10B with a mass of 10.0129 u, and the remaining 80.1% are 11B with a mass of 11.0093 u. The average atomic mass for boron is calculated to be:
\[\begin{align*}
\textrm{boron average mass} &=\mathrm{(0.199\times10.0129\: u)+(0.801\times11.0093\: u)}\\
&=\mathrm{1.99\: u+8.82\: u}\\
&=\mathrm{10.81\: u}
\end{align*}\]
It is important to understand that no single boron atom weighs exactly 10.8 u; 10.8 u is the average mass of a large number of naturally occurring boron atoms. The individual boron atoms weigh either approximately 10 u or 11 u.
A meteorite found in central Indiana contains traces of the noble gas neon picked up from the solar wind during the meteorite’s trip through the solar system. Analysis of a sample of the gas showed that it consisted of 91.84% 20Ne (mass 19.9924 u), 0.47% 21Ne (mass 20.9940 u), and 7.69% 22Ne (mass 21.9914 u). What is the average mass of the neon in the solar wind?
Solution
\[\begin{align*}
\mathrm{average\: mass} &=\mathrm{(0.9184\times19.9924\: u)+(0.0047\times20.9940\: u)+(0.0769\times21.9914\: u)}\\
&=\mathrm{(18.36+0.099+1.69)\:u}\\
&=\mathrm{20.15\: u}
\end{align*}\]
The average mass of a neon atom in the solar wind is 20.15 u. The average mass of a terrestrial neon atom is 20.1796 u. This result demonstrates that we may find slight differences in the natural abundance of isotopes, depending on their origin. For some elements the differences in these isotope abundances are used to identify where the element came from. Neon from a meteorite has a different isotope distribution thane neon from the earth's crust. Lead from different mines can have different isotope distributions depending on the geology of the locations.
A sample of magnesium is found to contain 78.70% of 24Mg atoms (mass 23.98 u), 10.13% of 25Mg atoms (mass 24.99 u), and 11.17% of 26Mg atoms (mass 25.98 u). Calculate the average mass of a Mg atom.
- Answer
-
24.31 u
We can also do variations of this type of calculation, as shown in the next example.
Naturally occurring chlorine consists of 35Cl (mass 34.96885 u) and 37Cl (mass 36.96590 u), with an average mass of 35.453 u. What is the percent composition of Cl in terms of these two isotopes?
Solution
The average mass of chlorine is the fraction that is 35Cl times the mass of 35Cl plus the fraction that is 37Cl times the mass of 37Cl.
\[\mathrm{average\: mass=(fraction\: of\: ^{35}Cl\times mass\: of\: ^{35}Cl)+(fraction\: of\: ^{37}Cl\times mass\: of\: ^{37}Cl)}\]
If we let x represent the fraction that is 35Cl, then the fraction that is 37Cl is represented by 1.00 − x.
(The fraction that is 35Cl + the fraction that is 37Cl must add up to 1, so the fraction of 37Cl must equal 1.00 − the fraction of 35Cl.)
Substituting this into the average mass equation, we have:
\[\begin{align*}
\mathrm{35.453\: u} &=(x\times 34.96885\: \ce{u})+[(1.00-x)\times 36.96590\: \ce{u}]\\
35.453 &=34.96885x+36.96590-36.96590x\\
1.99705x &=1.513\\
x&=\dfrac{1.513}{1.99705}=0.7576
\end{align*}\]
So solving yields: x = 0.7576, which means that 1.00 − 0.7576 = 0.2424. Therefore, chlorine consists of 75.76% 35Cl and 24.24% 37Cl.
Naturally occurring copper consists of 63Cu (mass 62.9296 u) and 65Cu (mass 64.9278 u), with an average mass of 63.546 u. What is the percent composition of Cu in terms of these two isotopes?
- Answer
-
69.15% Cu-63 and 30.85% Cu-65
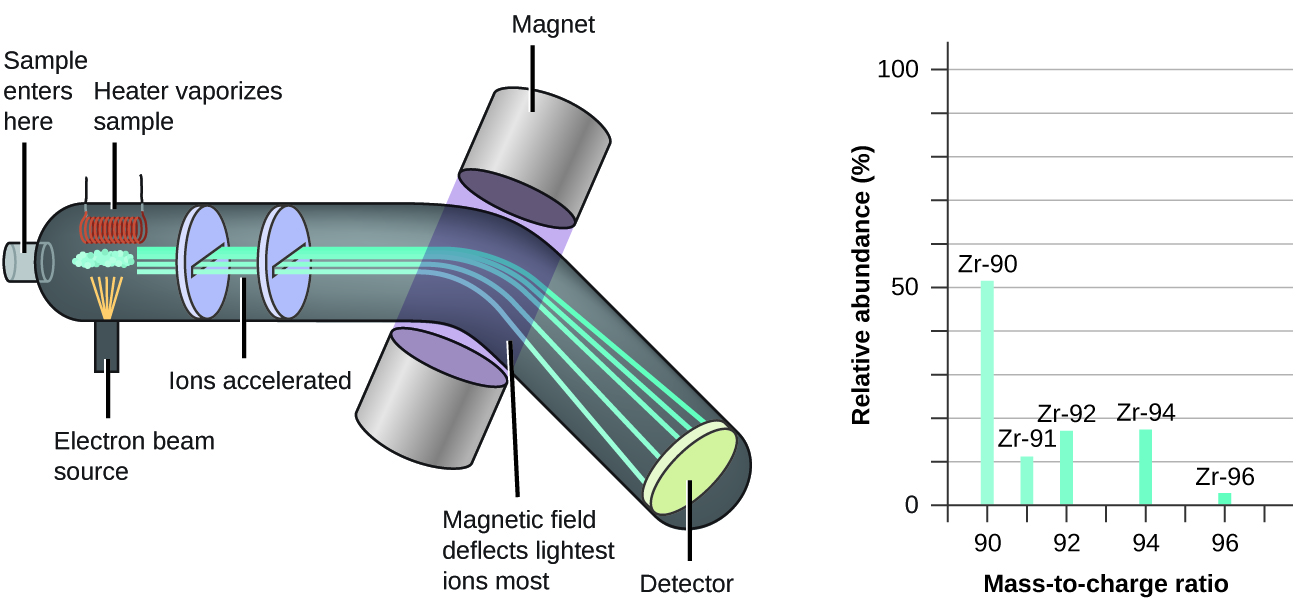
The occurrence and natural abundances of isotopes can be experimentally determined using an instrument called a mass spectrometer. Mass spectrometry (MS) is widely used in chemistry, forensics, medicine, environmental science, and many other fields to analyze and help identify the substances in a sample of material. In a typical mass spectrometer (Figure \(\PageIndex{5}\)), the sample is vaporized and exposed to a high-energy electron beam that causes the sample’s atoms (or molecules) to become electrically charged, typically by losing one or more electrons. These cations then pass through a (variable) electric or magnetic field that deflects each cation’s path to an extent that depends on both its mass and charge (similar to how the path of a large steel ball bearing rolling past a magnet is deflected to a lesser extent that that of a small steel BB). The ions are detected, and a plot of the relative number of ions generated versus their mass-to-charge ratios (a mass spectrum) is made. The height of each vertical feature or peak in a mass spectrum is proportional to the fraction of cations with the specified mass-to-charge ratio. Since its initial use during the development of modern atomic theory, MS has evolved to become a powerful tool for chemical analysis in a wide range of applications.
Video \(\PageIndex{1}\): Watch this video from the Royal Society for Chemistry for a brief description of the rudiments of mass spectrometry.
Finding the Averaged Atomic Mass of an Element - Video
The Periodic Table
As early chemists worked to purify ores and discovered more elements, they realized that various elements could be grouped together by their similar chemical behaviors. One such grouping includes lithium (Li), sodium (Na), and potassium (K): These elements all are shiny, conduct heat and electricity well, and have similar chemical properties. A second grouping includes calcium (Ca), strontium (Sr), and barium (Ba), which also are shiny, good conductors of heat and electricity, and have chemical properties in common. However, the specific properties of these two groupings are notably different from each other. For example: Li, Na, and K are much more reactive than are Ca, Sr, and Ba; Li, Na, and K form compounds with oxygen in a ratio of two of their atoms to one oxygen atom, whereas Ca, Sr, and Ba form compounds with one of their atoms to one oxygen atom. Fluorine (F), chlorine (Cl), bromine (Br), and iodine (I) also exhibit similar properties to each other, but these properties are drastically different from those of any of the elements above.
Dimitri Mendeleev in Russia (1869) and Lothar Meyer in Germany (1870) independently recognized that there was a periodic relationship among the properties of the elements known at that time. Both published tables with the elements arranged according to increasing atomic mass. But Mendeleev went one step further than Meyer: He used his table to predict the existence of elements that would have the properties similar to aluminum and silicon, but were yet unknown. The discoveries of gallium (1875) and germanium (1886) provided great support for Mendeleev’s work. Although Mendeleev and Meyer had a long dispute over priority, Mendeleev’s contributions to the development of the periodic table are now more widely recognized (Figure \(\PageIndex{6}\)).

By the twentieth century, it became apparent that the periodic relationship involved atomic numbers rather than atomic masses. The modern statement of this relationship, the periodic law, is as follows: the properties of the elements are periodic functions of their atomic numbers. A modern periodic table arranges the elements in increasing order of their atomic numbers and groups atoms with similar properties in the same vertical column (Figure \(\PageIndex{2}\)). Each box represents an element and contains its atomic number, symbol, average atomic mass, and (sometimes) name. The elements are arranged in seven horizontal rows, called periods or series, and 18 vertical columns, called groups. Groups are labeled at the top of each column. In the United States, the labels traditionally were numerals with capital letters. However, IUPAC recommends that the numbers 1 through 18 be used, and these labels are more common. For the table to fit on a single page, parts of two of the rows, a total of 14 columns, are usually written below the main body of the table.

Many elements differ dramatically in their chemical and physical properties, but some elements are similar in their behaviors. For example, many elements appear shiny, are malleable (able to be deformed without breaking) and ductile (can be drawn into wires), and conduct heat and electricity well. Other elements are not shiny, malleable, or ductile, and are poor conductors of heat and electricity. We can sort the elements into large classes with common properties: metals (elements that are shiny, malleable, good conductors of heat and electricity—shaded yellow); nonmetals (elements that appear dull, poor conductors of heat and electricity—shaded green); and metalloids (elements that conduct heat and electricity moderately well, and possess some properties of metals and some properties of nonmetals—shaded purple).
The elements can also be classified into the main-group elements (or representative elements) in the columns labeled 1, 2, and 13–18; the transition metals in the columns labeled 3–12; and inner transition metals in the two rows at the bottom of the table (the top-row elements are called lanthanides and the bottom-row elements are actinides; Figure \(\PageIndex{7}\)). The elements can be subdivided further by more specific properties, such as the composition of the compounds they form. For example, the elements in group 1 (the first column) form compounds that consist of one atom of the element and one atom of hydrogen. These elements (except hydrogen) are known as alkali metals, and they all have similar chemical properties. The elements in group 2 (the second column) form compounds consisting of one atom of the element and two atoms of hydrogen: These are called alkaline earth metals, with similar properties among members of that group. Other groups with specific names are the pnictogens (group 15), chalcogens (group 16), halogens (group 17), and the noble gases (group 18, also known as inert gases). The groups can also be referred to by the first element of the group: For example, the chalcogens can be called the oxygen group or oxygen family. Hydrogen is a unique, nonmetallic element with properties similar to both group 1 and group 17 elements. For that reason, hydrogen may be shown at the top of both groups, or by itself.
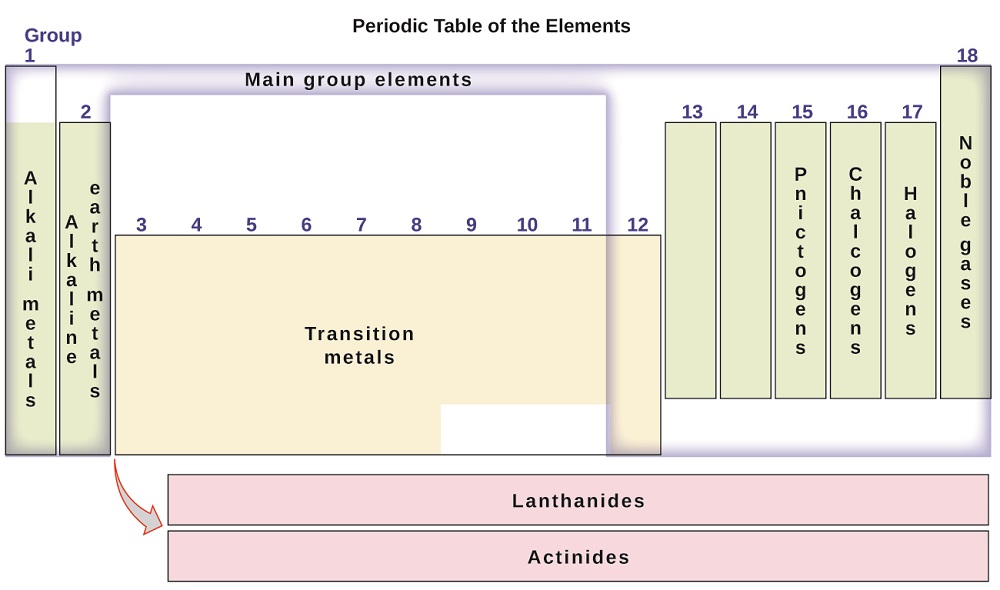
Atoms of each of the following elements are essential for life. Give the group name for the following elements:
- chlorine
- calcium
- sodium
- sulfur
Solution
The family names are as follows:
- halogen
- alkaline earth metal
- alkali metal
- chalcogen
Give the group name for each of the following elements:
- krypton
- selenium
- barium
- lithium
- Answer a
-
noble gas
- Answer b
-
chalcogen
- Answer c
-
alkaline earth metal
- Answer d
-
alkali metal
In studying the periodic table, you might have noticed something about the atomic masses of some of the elements. Element 43 (technetium), element 61 (promethium), and most of the elements with atomic number 84 (polonium) and higher have their atomic mass given in square brackets. This is done for elements that consist entirely of unstable, radioactive isotopes (you will learn more about radioactivity in the nuclear chemistry chapter). An average atomic weight cannot be determined for these elements because their radioisotopes may vary significantly in relative abundance, depending on the source, or may not even exist in nature. The number in square brackets is the atomic mass number (and approximate atomic mass) of the most stable isotope of that element.
Summary
An atom consists of a small, positively charged nucleus surrounded by electrons. The nucleus contains protons and neutrons; its diameter is about 100,000 times smaller than that of the atom. The mass of one atom is usually expressed in unified atomic mass units (u), which is referred to as the atomic mass. A u is defined as exactly \(1/12\) of the mass of a carbon-12 atom and is equal to 1.6605 \(\times\) 10−24 g.
Protons are relatively heavy particles with a charge of 1+ and a mass of 1.0073 u. Neutrons are relatively heavy particles with no charge and a mass of 1.0087 u. Electrons are light particles with a charge of 1− and a mass of 0.00055 u. The number of protons in the nucleus is called the atomic number (Z) and is the property that defines an atom’s elemental identity. The sum of the numbers of protons and neutrons in the nucleus is called the mass number and, expressed in u, is approximately equal to the mass of the atom. An atom is neutral when it contains equal numbers of electrons and protons.
Isotopes of an element are atoms with the same atomic number but different mass numbers; isotopes of an element, therefore, differ from each other only in the number of neutrons within the nucleus. When a naturally occurring element is composed of several isotopes, the atomic mass of the element represents the average of the masses of the isotopes involved. A chemical symbol identifies the atoms in a substance using symbols, which are one-, two-, or three-letter abbreviations for the atoms.
The discovery of the periodic recurrence of similar properties among the elements led to the formulation of the periodic table, in which the elements are arranged in order of increasing atomic number in rows known as periods and columns known as groups. Elements in the same group of the periodic table have similar chemical properties. Elements can be classified as metals, metalloids, and nonmetals, or as a main-group elements, transition metals, and inner transition metals. Groups are numbered 1–18 from left to right. The elements in group 1 are known as the alkali metals; those in group 2 are the alkaline earth metals; those in 15 are the pnictogens; those in 16 are the chalcogens; those in 17 are the halogens; and those in 18 are the noble gases.
Key Equations
- \(\mathrm{average\: mass}=\sum_{i}(\mathrm{fractional\: abundance \times isotopic\: mass})_i\)
Glossary
- actinide
- inner transition metal in the bottom of the bottom two rows of the periodic table
- alkali metal
- element in group 1
- alkaline earth metal
- element in group 2
- anion
- negatively charged atom or molecule (contains more electrons than protons)
- atomic mass
- average mass of atoms of an element, expressed in u
- atomic number (Z)
- number of protons in the nucleus of an atom
- cation
- positively charged atom or molecule (contains fewer electrons than protons)
- chalcogen
- element in group 16
- chemical symbol
- one-, two-, or three-letter abbreviation used to represent an element or its atoms
- Dalton (Da)
- alternative unit equivalent to the atomic mass unit
- fundamental unit of charge
- (also called the elementary charge) equals the magnitude of the charge of an electron (e) with e = 1.602 \(\times\) 10−19 C
- group
- vertical column of the periodic table
- halogen
- element in group 17
- inert gas
- (also, noble gas) element in group 18
- inner transition metal
- (also, lanthanide or actinide) element in the bottom two rows; if in the first row, also called lanthanide, or if in the second row, also called actinide
- ion
- electrically charged atom or molecule (contains unequal numbers of protons and electrons)
- lanthanide
- inner transition metal in the top of the bottom two rows of the periodic table
- main-group element
- (also, representative element) element in columns 1, 2, and 12–18
- mass number (A)
- sum of the numbers of neutrons and protons in the nucleus of an atom
- metal
- element that is shiny, malleable, good conductor of heat and electricity
- metalloid
- element that conducts heat and electricity moderately well, and possesses some properties of metals and some properties of nonmetals
- noble gas
- (also, inert gas) element in group 18
- nonmetal
- element that appears dull, poor conductor of heat and electricity
- period
- (also, series) horizontal row of the periodic table
- periodic law
- properties of the elements are periodic function of their atomic numbers.
- periodic table
- table of the elements that places elements with similar chemical properties close together
- pnictogen
- element in group 15
- representative element
- (also, main-group element) element in columns 1, 2, and 12–18
- transition metal
- element in columns 3–11
- series
- (also, period) horizontal row of the period table
- unified atomic mass unit (u)
- (also, atomic mass unit (amu) or Dalton, Da) unit of mass equal to \(\dfrac{1}{12}\) of the mass of a 12C atom
Contributors and Attributions
Paul Flowers (University of North Carolina - Pembroke), Klaus Theopold (University of Delaware) and Richard Langley (Stephen F. Austin State University) with contributing authors. Textbook content produced by OpenStax College is licensed under a Creative Commons Attribution License 4.0 license. Download for free at http://cnx.org/contents/85abf193-2bd...a7ac8df6@9.110).