Discovering Sub-atomic Particles
- Page ID
- 54144
\( \newcommand{\vecs}[1]{\overset { \scriptstyle \rightharpoonup} {\mathbf{#1}} } \)
\( \newcommand{\vecd}[1]{\overset{-\!-\!\rightharpoonup}{\vphantom{a}\smash {#1}}} \)
\( \newcommand{\id}{\mathrm{id}}\) \( \newcommand{\Span}{\mathrm{span}}\)
( \newcommand{\kernel}{\mathrm{null}\,}\) \( \newcommand{\range}{\mathrm{range}\,}\)
\( \newcommand{\RealPart}{\mathrm{Re}}\) \( \newcommand{\ImaginaryPart}{\mathrm{Im}}\)
\( \newcommand{\Argument}{\mathrm{Arg}}\) \( \newcommand{\norm}[1]{\| #1 \|}\)
\( \newcommand{\inner}[2]{\langle #1, #2 \rangle}\)
\( \newcommand{\Span}{\mathrm{span}}\)
\( \newcommand{\id}{\mathrm{id}}\)
\( \newcommand{\Span}{\mathrm{span}}\)
\( \newcommand{\kernel}{\mathrm{null}\,}\)
\( \newcommand{\range}{\mathrm{range}\,}\)
\( \newcommand{\RealPart}{\mathrm{Re}}\)
\( \newcommand{\ImaginaryPart}{\mathrm{Im}}\)
\( \newcommand{\Argument}{\mathrm{Arg}}\)
\( \newcommand{\norm}[1]{\| #1 \|}\)
\( \newcommand{\inner}[2]{\langle #1, #2 \rangle}\)
\( \newcommand{\Span}{\mathrm{span}}\) \( \newcommand{\AA}{\unicode[.8,0]{x212B}}\)
\( \newcommand{\vectorA}[1]{\vec{#1}} % arrow\)
\( \newcommand{\vectorAt}[1]{\vec{\text{#1}}} % arrow\)
\( \newcommand{\vectorB}[1]{\overset { \scriptstyle \rightharpoonup} {\mathbf{#1}} } \)
\( \newcommand{\vectorC}[1]{\textbf{#1}} \)
\( \newcommand{\vectorD}[1]{\overrightarrow{#1}} \)
\( \newcommand{\vectorDt}[1]{\overrightarrow{\text{#1}}} \)
\( \newcommand{\vectE}[1]{\overset{-\!-\!\rightharpoonup}{\vphantom{a}\smash{\mathbf {#1}}}} \)
\( \newcommand{\vecs}[1]{\overset { \scriptstyle \rightharpoonup} {\mathbf{#1}} } \)
\( \newcommand{\vecd}[1]{\overset{-\!-\!\rightharpoonup}{\vphantom{a}\smash {#1}}} \)
\(\newcommand{\avec}{\mathbf a}\) \(\newcommand{\bvec}{\mathbf b}\) \(\newcommand{\cvec}{\mathbf c}\) \(\newcommand{\dvec}{\mathbf d}\) \(\newcommand{\dtil}{\widetilde{\mathbf d}}\) \(\newcommand{\evec}{\mathbf e}\) \(\newcommand{\fvec}{\mathbf f}\) \(\newcommand{\nvec}{\mathbf n}\) \(\newcommand{\pvec}{\mathbf p}\) \(\newcommand{\qvec}{\mathbf q}\) \(\newcommand{\svec}{\mathbf s}\) \(\newcommand{\tvec}{\mathbf t}\) \(\newcommand{\uvec}{\mathbf u}\) \(\newcommand{\vvec}{\mathbf v}\) \(\newcommand{\wvec}{\mathbf w}\) \(\newcommand{\xvec}{\mathbf x}\) \(\newcommand{\yvec}{\mathbf y}\) \(\newcommand{\zvec}{\mathbf z}\) \(\newcommand{\rvec}{\mathbf r}\) \(\newcommand{\mvec}{\mathbf m}\) \(\newcommand{\zerovec}{\mathbf 0}\) \(\newcommand{\onevec}{\mathbf 1}\) \(\newcommand{\real}{\mathbb R}\) \(\newcommand{\twovec}[2]{\left[\begin{array}{r}#1 \\ #2 \end{array}\right]}\) \(\newcommand{\ctwovec}[2]{\left[\begin{array}{c}#1 \\ #2 \end{array}\right]}\) \(\newcommand{\threevec}[3]{\left[\begin{array}{r}#1 \\ #2 \\ #3 \end{array}\right]}\) \(\newcommand{\cthreevec}[3]{\left[\begin{array}{c}#1 \\ #2 \\ #3 \end{array}\right]}\) \(\newcommand{\fourvec}[4]{\left[\begin{array}{r}#1 \\ #2 \\ #3 \\ #4 \end{array}\right]}\) \(\newcommand{\cfourvec}[4]{\left[\begin{array}{c}#1 \\ #2 \\ #3 \\ #4 \end{array}\right]}\) \(\newcommand{\fivevec}[5]{\left[\begin{array}{r}#1 \\ #2 \\ #3 \\ #4 \\ #5 \\ \end{array}\right]}\) \(\newcommand{\cfivevec}[5]{\left[\begin{array}{c}#1 \\ #2 \\ #3 \\ #4 \\ #5 \\ \end{array}\right]}\) \(\newcommand{\mattwo}[4]{\left[\begin{array}{rr}#1 \amp #2 \\ #3 \amp #4 \\ \end{array}\right]}\) \(\newcommand{\laspan}[1]{\text{Span}\{#1\}}\) \(\newcommand{\bcal}{\cal B}\) \(\newcommand{\ccal}{\cal C}\) \(\newcommand{\scal}{\cal S}\) \(\newcommand{\wcal}{\cal W}\) \(\newcommand{\ecal}{\cal E}\) \(\newcommand{\coords}[2]{\left\{#1\right\}_{#2}}\) \(\newcommand{\gray}[1]{\color{gray}{#1}}\) \(\newcommand{\lgray}[1]{\color{lightgray}{#1}}\) \(\newcommand{\rank}{\operatorname{rank}}\) \(\newcommand{\row}{\text{Row}}\) \(\newcommand{\col}{\text{Col}}\) \(\renewcommand{\row}{\text{Row}}\) \(\newcommand{\nul}{\text{Nul}}\) \(\newcommand{\var}{\text{Var}}\) \(\newcommand{\corr}{\text{corr}}\) \(\newcommand{\len}[1]{\left|#1\right|}\) \(\newcommand{\bbar}{\overline{\bvec}}\) \(\newcommand{\bhat}{\widehat{\bvec}}\) \(\newcommand{\bperp}{\bvec^\perp}\) \(\newcommand{\xhat}{\widehat{\xvec}}\) \(\newcommand{\vhat}{\widehat{\vvec}}\) \(\newcommand{\uhat}{\widehat{\uvec}}\) \(\newcommand{\what}{\widehat{\wvec}}\) \(\newcommand{\Sighat}{\widehat{\Sigma}}\) \(\newcommand{\lt}{<}\) \(\newcommand{\gt}{>}\) \(\newcommand{\amp}{&}\) \(\definecolor{fillinmathshade}{gray}{0.9}\)Skills to Develop
- Describe the three types of radioactivity
- Understand the difference between atoms. ions, and isotopes
- Define the subatomic particles that make up atoms
At the end of the last section (Valence), we mentioned the problem of the masses of iodine and tellurium, which were "out of order" in the periodic table. This was only one of several problems facing chemists at this point. Another was the rare earth elements (the lanthanoides and actinoides, at the bottom of the periodic table). The rare earth elements were extremely similar to each other, so it was very hard to separate them, determine the masses, and fit them into the periodic table.
Another problem was ions. Ions were charged chemical entities that moved through solutions carrying electric current when electricity was applied. Chemists knew they existed, but could not explain how they formed. Cations were positively charged, while anions were negatively charged. Hydrogen was known to form positive ions with a particular charge. But where did the charges come from? Berzelius had thought that the atoms themselves were charged, but this didn't make sense after Avogadro's hypothesis was accepted, since in that case the two O atoms in O2 would repel each other, not form a molecule. Of course, this was mostly a problem to physical chemists, because physicists mostly still didn't believe in atoms.
Experiments by Davy, Faraday and others showed that solids usually conduct electricity without changing themselves; liquids are decomposed by electrical current, and gases conduct badly and only at high voltage, but become better conductors at low pressure. Glass tubes of low pressure gas glow; examples include neon lights in store windows, fluorescent light bulbs, and yellow sodium lamps sometimes used at street lights. When glass tubes full of very low pressure gas were made by expert glassblower Geissler after 1850, a glowing spot would appear somewhere on the glass when the tube was connected to a high voltage. This spot could be moved by a magnet, suggesting that it was negatively charged. A beam or ray was coming out of the cathode (the electrode to which cations move), and this beam caused the glass to glow. (This is exactly how old TVs work, from before there were flat screens.)
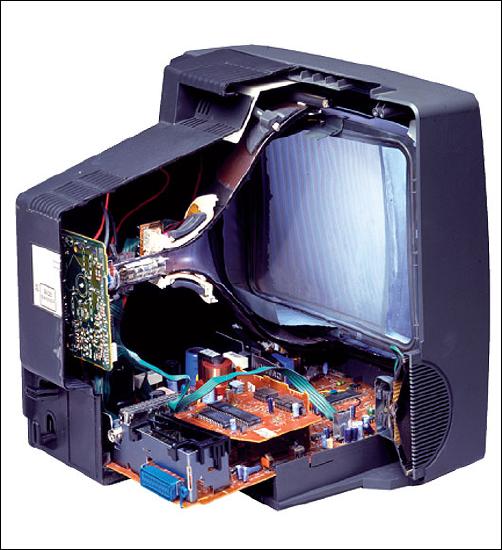
But what was the beam that came out of the cathode? Some thought it was negatively-charged particles, while others (especially physicists) thought it was a wave. Thomson convinced himself that the beam is a negatively charged particle, which can be moved using electric or magnetic fields. He estimated that the particle has a charge/mass ratio 1000x greater than the hydrogen ion, but with the opposite charge. The ray that comes out of the cathode is the same whatever the material, and he found that you can generate the same particle by heating metal filaments very hot or exposing a metal surface to UV light (the light that gives you sunburns).
What are cathode rays? Electrons! When Thomson suggested in 1897 that either they had a much higher charge or a much smaller mass than hydrogen ions, many people were skeptical. However, careful measurements by Millikan (starting 1908, continuing for almost 10 years!) of falling droplets of mist in an electric or magnetic field revealed that electrons have the same charge as hydrogen ions, but are almost ~1/2000 less massive, so in fact Thomson guessed electrons were twice as big as they really are.
In summary, electrons have an equal but opposite charge to hydrogen ions, but hydrogen ions weigh 2000x more than electrons. Electrons are part of every kind of atom.
Other than electrons, what are atoms made of? X-rays were discovered in 1895 when Roentgen noticed a ray that passed through the glass of his cathode ray tube and developed photographic film placed in its path, even if it was wrapped in dark paper. Inspired by this discovery, Becquerel studied the fluorescence (this means glowing after being exposed to light) of minerals and whether this could develop film through dark paper. He used sunlight to make the minerals fluoresce, but discovered that some minerals fluoresce even without sunlight. Minerals that contain the elements uranium and thorium have this property, which is called radioactivity. Three types of radioactivity were discovered, α (positive charge), β (electrons, same as cathode rays) and γ (light, similar to x-rays). This radiation is a property of the element, and is not changed by chemical combinations. It was also discovered that radioactive elements decay into products that closely resemble known elements, except that the masses are different. These decay products have exactly the same chemical properties as the known elements, and once mixed together, they can't be separated by chemical means. But the masses were different. The evidence against Dalton's "identical and indivisible atoms of each element" was getting strong.
Rutherford studied the α-radiation, and found that it had a positive charge, and an e/m (charge/mass) ratio 1/2 that of a hydrogen ion. Later he proved that it is a charged form of the element helium (He).
Finally, using an early version of the mass spectrometer, which measures e/m by accelerating charged particles in an electric or magnetic field, Aston determined that neon (Ne) atoms have masses of 20 or 22, not 20.2, then the accepted value. Here's another case where knowing the precision of your experiment is important: if he couldn't tell the difference between 20.0 and 20.2, this wouldn't have been useful! The two versions of Ne are called isotopes. Isotopes are atoms of the same element with have the same chemical properties, but have different masses.
What is the structure of an atom? At this point, it was clear that Dalton had not been entirely correct, because atoms of one element could exist in different forms, or isotopes. It was also becoming clear that atoms could be divided into smaller parts, like electrons. But how were the electrons and the positive parts (like the part of He that is α-radiation) arranged, and what was the positive particle? It seemed like there was a lot of empty space in atoms, because cathode rays could pass through aluminum windows. Many theories were considered, but ultimately two of Rutherford's students, Geiger and Marsden, found an answer. They observed that α-rays directed at a thin gold (Au) foil usually passed through the foil (though not usually quite straight), but sometimes the foil knocked them back in the direction they came from. These α-particles must have bumped into (or been repelled by a large positive charge in) something very heavy, to have so large a change in direction. In 1911, Rutherford proposed that atoms consist of a small dense particle, the nucleus, which contains most of the atom's mass and all of its positive charge, with electrons moving through a large empty space around the nucleus. But they were a little confused about the mass and charge of the nucleus.

In 1913, a very young chemist named Henry Moseley measured the fluorescence wavelength produced when x-rays shine on pure elemental samples. He discovered that if you graph the square root of the fluorescence frequency vs the element's location in the periodic table (such as H, 1; C, 6; Hg, 80) you get a straight line. The location in the periodic table is called atomic number. This confirmed the ordering of Te/I in the periodic table and a few other similar problems. He was also able to determine where undiscovered elements were missing in the table, and clarify identification of rare earth elements, which was very difficult because they were so similar. He was 26 years old, and he died a year later in WWI. Moseley's work showed that the ordering of the periodic table is based on the atomic number, which is the number of positive charges in the nucleus and also the number of electrons. The unit of positive charge is a hydrogen nucleus, or proton.
The mass of the nucleus continued to be confusing even after the amount of positive charge had been determined by Moseley's atomic numbers. The atomic weights were about double what they should be if the nucleus held only protons. A proposal to explain this was that there were some electrons in the nucleus, canceling out the charge of half the protons. Later radiochemistry experiments showed the existence of the neutron, which has about the mass of a proton and no charge. This was understood to be the extra mass in nuclei. Now, though, we know that neutrons can decay into protons and electrons (and some other less familiar particles), so the earlier idea wasn't far off.
Summary
Atoms are composed of protons, neutrons, and electrons. Protons and neutrons make most of the atom's mass and all of its positive charge in the nucleus. Protons are subatomic particles that contain a positive charge. Essentially, they are hydrogen nuclei. Neutrons are subatomic particles that have the same mass as a proton but not charge. Electrons are subatomic particles with a negative charge that are found outside of the atom's nucleus. An element's atomic number provides its location on the periodic table as well as describe how many protons are in an atoms of that particular element. Ions are atoms that are either positively or negatively charged due to the number of protons or electrons in an atom not being equal to each other. Isotopes are atoms that have a different number of neutrons in the nucleus than normal. There are three main types of radioactivity: α , β, and γ. α (Alpha) radiation involves the emission of a helium nucleus, which is two protons and two neutrons. β (Beta) radiation involves the emission of an electron from the atom's nucleus. γ (Gamma) radiation involves the emission of high-energy electromagnetic radiation.
Contributors and Attributions
Emily V Eames (City College of San Francisco)