4.2: Single Bonds and Molecular Shape
- Page ID
- 355280
\( \newcommand{\vecs}[1]{\overset { \scriptstyle \rightharpoonup} {\mathbf{#1}} } \)
\( \newcommand{\vecd}[1]{\overset{-\!-\!\rightharpoonup}{\vphantom{a}\smash {#1}}} \)
\( \newcommand{\id}{\mathrm{id}}\) \( \newcommand{\Span}{\mathrm{span}}\)
( \newcommand{\kernel}{\mathrm{null}\,}\) \( \newcommand{\range}{\mathrm{range}\,}\)
\( \newcommand{\RealPart}{\mathrm{Re}}\) \( \newcommand{\ImaginaryPart}{\mathrm{Im}}\)
\( \newcommand{\Argument}{\mathrm{Arg}}\) \( \newcommand{\norm}[1]{\| #1 \|}\)
\( \newcommand{\inner}[2]{\langle #1, #2 \rangle}\)
\( \newcommand{\Span}{\mathrm{span}}\)
\( \newcommand{\id}{\mathrm{id}}\)
\( \newcommand{\Span}{\mathrm{span}}\)
\( \newcommand{\kernel}{\mathrm{null}\,}\)
\( \newcommand{\range}{\mathrm{range}\,}\)
\( \newcommand{\RealPart}{\mathrm{Re}}\)
\( \newcommand{\ImaginaryPart}{\mathrm{Im}}\)
\( \newcommand{\Argument}{\mathrm{Arg}}\)
\( \newcommand{\norm}[1]{\| #1 \|}\)
\( \newcommand{\inner}[2]{\langle #1, #2 \rangle}\)
\( \newcommand{\Span}{\mathrm{span}}\) \( \newcommand{\AA}{\unicode[.8,0]{x212B}}\)
\( \newcommand{\vectorA}[1]{\vec{#1}} % arrow\)
\( \newcommand{\vectorAt}[1]{\vec{\text{#1}}} % arrow\)
\( \newcommand{\vectorB}[1]{\overset { \scriptstyle \rightharpoonup} {\mathbf{#1}} } \)
\( \newcommand{\vectorC}[1]{\textbf{#1}} \)
\( \newcommand{\vectorD}[1]{\overrightarrow{#1}} \)
\( \newcommand{\vectorDt}[1]{\overrightarrow{\text{#1}}} \)
\( \newcommand{\vectE}[1]{\overset{-\!-\!\rightharpoonup}{\vphantom{a}\smash{\mathbf {#1}}}} \)
\( \newcommand{\vecs}[1]{\overset { \scriptstyle \rightharpoonup} {\mathbf{#1}} } \)
\( \newcommand{\vecd}[1]{\overset{-\!-\!\rightharpoonup}{\vphantom{a}\smash {#1}}} \)
\(\newcommand{\avec}{\mathbf a}\) \(\newcommand{\bvec}{\mathbf b}\) \(\newcommand{\cvec}{\mathbf c}\) \(\newcommand{\dvec}{\mathbf d}\) \(\newcommand{\dtil}{\widetilde{\mathbf d}}\) \(\newcommand{\evec}{\mathbf e}\) \(\newcommand{\fvec}{\mathbf f}\) \(\newcommand{\nvec}{\mathbf n}\) \(\newcommand{\pvec}{\mathbf p}\) \(\newcommand{\qvec}{\mathbf q}\) \(\newcommand{\svec}{\mathbf s}\) \(\newcommand{\tvec}{\mathbf t}\) \(\newcommand{\uvec}{\mathbf u}\) \(\newcommand{\vvec}{\mathbf v}\) \(\newcommand{\wvec}{\mathbf w}\) \(\newcommand{\xvec}{\mathbf x}\) \(\newcommand{\yvec}{\mathbf y}\) \(\newcommand{\zvec}{\mathbf z}\) \(\newcommand{\rvec}{\mathbf r}\) \(\newcommand{\mvec}{\mathbf m}\) \(\newcommand{\zerovec}{\mathbf 0}\) \(\newcommand{\onevec}{\mathbf 1}\) \(\newcommand{\real}{\mathbb R}\) \(\newcommand{\twovec}[2]{\left[\begin{array}{r}#1 \\ #2 \end{array}\right]}\) \(\newcommand{\ctwovec}[2]{\left[\begin{array}{c}#1 \\ #2 \end{array}\right]}\) \(\newcommand{\threevec}[3]{\left[\begin{array}{r}#1 \\ #2 \\ #3 \end{array}\right]}\) \(\newcommand{\cthreevec}[3]{\left[\begin{array}{c}#1 \\ #2 \\ #3 \end{array}\right]}\) \(\newcommand{\fourvec}[4]{\left[\begin{array}{r}#1 \\ #2 \\ #3 \\ #4 \end{array}\right]}\) \(\newcommand{\cfourvec}[4]{\left[\begin{array}{c}#1 \\ #2 \\ #3 \\ #4 \end{array}\right]}\) \(\newcommand{\fivevec}[5]{\left[\begin{array}{r}#1 \\ #2 \\ #3 \\ #4 \\ #5 \\ \end{array}\right]}\) \(\newcommand{\cfivevec}[5]{\left[\begin{array}{c}#1 \\ #2 \\ #3 \\ #4 \\ #5 \\ \end{array}\right]}\) \(\newcommand{\mattwo}[4]{\left[\begin{array}{rr}#1 \amp #2 \\ #3 \amp #4 \\ \end{array}\right]}\) \(\newcommand{\laspan}[1]{\text{Span}\{#1\}}\) \(\newcommand{\bcal}{\cal B}\) \(\newcommand{\ccal}{\cal C}\) \(\newcommand{\scal}{\cal S}\) \(\newcommand{\wcal}{\cal W}\) \(\newcommand{\ecal}{\cal E}\) \(\newcommand{\coords}[2]{\left\{#1\right\}_{#2}}\) \(\newcommand{\gray}[1]{\color{gray}{#1}}\) \(\newcommand{\lgray}[1]{\color{lightgray}{#1}}\) \(\newcommand{\rank}{\operatorname{rank}}\) \(\newcommand{\row}{\text{Row}}\) \(\newcommand{\col}{\text{Col}}\) \(\renewcommand{\row}{\text{Row}}\) \(\newcommand{\nul}{\text{Nul}}\) \(\newcommand{\var}{\text{Var}}\) \(\newcommand{\corr}{\text{corr}}\) \(\newcommand{\len}[1]{\left|#1\right|}\) \(\newcommand{\bbar}{\overline{\bvec}}\) \(\newcommand{\bhat}{\widehat{\bvec}}\) \(\newcommand{\bperp}{\bvec^\perp}\) \(\newcommand{\xhat}{\widehat{\xvec}}\) \(\newcommand{\vhat}{\widehat{\vvec}}\) \(\newcommand{\uhat}{\widehat{\uvec}}\) \(\newcommand{\what}{\widehat{\wvec}}\) \(\newcommand{\Sighat}{\widehat{\Sigma}}\) \(\newcommand{\lt}{<}\) \(\newcommand{\gt}{>}\) \(\newcommand{\amp}{&}\) \(\definecolor{fillinmathshade}{gray}{0.9}\)\(\mathrm{C–C}\) and \(\mathrm{C–H}\) bonds are described by molecular orbitals; calculations indicate that most of the electron density associated with these orbitals lies between the two nuclei. The \(\mathrm{C–H}\) bonds have a length of \(109 \times 10^{-12} \mathrm{~m}\) (\(109 \mathrm{~pm}\)) while the \(\mathrm{C–C}\) bond is approximately 50% longer, \(154 \times 10^{-12} \mathrm{~m}\) (\(154 \mathrm{~pm}\)). This is because the \(\mathrm{C–C}\) bonding orbital is made from \(\mathrm{sp}^{3}\) hybrid orbitals, which are larger than the \(1\mathrm{s}\) orbital that hydrogen uses to form bonds. These so-called σ (sigma) bonds have an interesting property; the atoms that they link can spin relative to each other without breaking the bond between them. For a \(\mathrm{C–H}\) bond, if the \(\mathrm{H}\) spins it would be impossible to tell, since the \(\mathrm{H}\) atom is radially symmetric around the \(\mathrm{C–H}\) bond axis. But if the carbons in the \(\mathrm{C–C}\) bond of ethane spin relative to each other, then it is possible to observe different arrangements by looking down the \(\mathrm{C–C}\) bond axis. For example:
and
are both representations of ethane (the \(\mathrm{C–C}\) bond is not seen in this depiction because you are looking straight down the \(\mathrm{C–C}\) bond). They appear different because the arrangement of the atoms is different in space, but in fact at room temperature these two arrangements can easily interconvert by rotating around the \(\mathrm{C–C}\) bond.
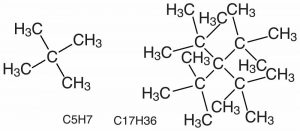
This raises another point to consider, namely that starting (and stopping) bond rotations requires energy. Similarly, there can be vibrations along the length of a bond, which again involves the absorption or release of energy. We will consider this further later on.[6] In the case of the rotating bond it turns out that as the bulk of the groups attached to the carbons increases the energy required for the rotation around the \(\mathrm{C–C}\) bond also increases. Big, bulky groups can bump into each other, occupying each other’s space causing electron-electron repulsions and raising the energy of any shape where the groups are too close. This tends to lock the molecule into specific orientations that can influence the compound’s physical properties. An example of how structure interferes with the formation of a molecule is a molecule containing 17 carbon atoms and 36 hydrogen atoms (\(\rightarrow\)); although it is possible to draw this molecule it has never been synthesized because the atoms crowd each other, and intrude on each other’s space. It is possible, however, to synthesize molecules with the same number of carbon atoms but fewer hydrogen atoms.[7] Can you produce a plausible explanation for why?
Collapsing Real Structures Down to 2-Dimensional Representations
Now, an obvious problem with complex three-dimensional molecules, even those made up only of hydrogen and carbon, is how to convey their structure when they must be depicted in two dimensions, like when you are writing on paper. Research indicates that students (that is, most people) have a tough time with this task, which is why we will describe various approaches here.
Before we begin, we need to have some rules. Let us use the set of possible molecules that contain 5 carbon atoms and 12 hydrogen atoms; these are generically known as pentanes. You can begin with a piece of paper and a pencil; how many different molecules can you draw with the composition of \(\mathrm{C}_{5}\mathrm{H}_{12}\)? Clearly \(\mathrm{C}_{5}\mathrm{H}_{12}\) does not uniquely define the structure of the molecule; it is better to use their distinct names: pentane, isopentane, and neopentane (\(\rightarrow\)). Each of the different molecules you have drawn has the same molecular formula but a different shape and, it turns out, different properties. For example, pentane has a boiling point of \(308 \mathrm{~K}\), whereas the boiling points of isopentane and neopentane are \(301 \mathrm{~K}\) and \(283 \mathrm{~K}\), respectively. Their shape, rather than their elemental composition, influences the strength of the attractions between the individual molecules, which in turn influences their boiling points. We call these kinds of related compounds structural isomers, which means they have the same composition (for example \(\mathrm{C}_{5}\mathrm{H}_{12}\)) but their constituent atoms are connected differently to give different structures and shapes.
It is common to use a number of different types of representations to picture molecules. One way is through what are known as text formulas (or linear formulae). In this scheme, pentane is written \(\mathrm{CH}_{3}\mathrm{–CH}_{2}\(\mathrm{–CH}_{2}\mathrm{–CH}_{2}\(\mathrm{–CH}_{3}\), which can also be written as \(\mathrm{CH}_{3}-\left[\mathrm{CH}_{2}\right]_{3}-\mathrm{CH}_{3}\). This captures some of the structural subtleties of pentane, but not all. For example, it does not illustrate the fact that the molecule is not strictly linear. Nevertheless, we can already anticipate complications. How would we write isopentane? The most obvious way would be \(\left(\mathrm{CH}_{3}\right)_{2} \mathrm{CHCH}_{2} \mathrm{CH}_{3}\). Neopentane is written as \(\left(\mathrm{CH}_{3}\right)_{4} \mathrm{C}\). Does that make sense? Try deciphering them. We will return to this point later on in this chapter.
If we followed the logic of this approach we could draw a more complete representation of pentane, isopentane, and neopentane as Lewis structures, but again, we are missing the three-dimensionality. You might even be led to think that the molecules are actually flat when they are much more like balls. Although it is possible to make the representation a little more realistic by trying to indicate three-dimensionality using the wedge and dash symbols, these structures become very complicated very fast. It is not really practical to draw out full 3D structures for larger, complex molecules. One important skill you will need to master is the ways that short-hand structures (such as Lewis structures) can provide information about the 3D structure of a molecule that allows us to predict chemical and physical properties.
There is one more representation you will often see used that leaves out even more information. In the line structure the only things that are shown are the bonds between carbons! So for example for the pentanes (\(\mathrm{C}_{5}\mathrm{H}_{12}\)) we can draw structures such as those shown in the figure that omit all the symbols for atoms and all the \(\mathrm{C–H}\) bonds. These structures should be used with caution because it is very easy to forget atoms or bonds when they are not in the representation. But what these line structures do show clearly is how the carbon atoms are connected, which can be very helpful at times.
Questions to Answer
- How many different compounds can you draw for \(\mathrm{C}_{6}\mathrm{H}_{14}\)? Draw out the full Lewis structure, the condensed formula, and the line formula.
- What are the advantages and disadvantages of each type of structure?
Questions for Later:
- When you think about rotating around a \(\mathrm{C–C}\) bond (say in ethane), there are more and less stable orientations. Which orientation do you think is the most stable and why?
- Now imagine a butane molecule (\(\mathrm{C}_{4}\mathrm{H}_{10}\)) looking along the \(\mathrm{C}_{2-3}\) bond. You would see one methyl group and two hydrogen atoms bonded to the two carbon atoms. How would that influence rotation around the \(\mathrm{C–C}\) bond we have been considering?