Section 4.2.1: Orbital Overlap
- Last updated
- Save as PDF
- Page ID
- 440784
\( \newcommand{\vecs}[1]{\overset { \scriptstyle \rightharpoonup} {\mathbf{#1}} } \)
\( \newcommand{\vecd}[1]{\overset{-\!-\!\rightharpoonup}{\vphantom{a}\smash {#1}}} \)
\( \newcommand{\id}{\mathrm{id}}\) \( \newcommand{\Span}{\mathrm{span}}\)
( \newcommand{\kernel}{\mathrm{null}\,}\) \( \newcommand{\range}{\mathrm{range}\,}\)
\( \newcommand{\RealPart}{\mathrm{Re}}\) \( \newcommand{\ImaginaryPart}{\mathrm{Im}}\)
\( \newcommand{\Argument}{\mathrm{Arg}}\) \( \newcommand{\norm}[1]{\| #1 \|}\)
\( \newcommand{\inner}[2]{\langle #1, #2 \rangle}\)
\( \newcommand{\Span}{\mathrm{span}}\)
\( \newcommand{\id}{\mathrm{id}}\)
\( \newcommand{\Span}{\mathrm{span}}\)
\( \newcommand{\kernel}{\mathrm{null}\,}\)
\( \newcommand{\range}{\mathrm{range}\,}\)
\( \newcommand{\RealPart}{\mathrm{Re}}\)
\( \newcommand{\ImaginaryPart}{\mathrm{Im}}\)
\( \newcommand{\Argument}{\mathrm{Arg}}\)
\( \newcommand{\norm}[1]{\| #1 \|}\)
\( \newcommand{\inner}[2]{\langle #1, #2 \rangle}\)
\( \newcommand{\Span}{\mathrm{span}}\) \( \newcommand{\AA}{\unicode[.8,0]{x212B}}\)
\( \newcommand{\vectorA}[1]{\vec{#1}} % arrow\)
\( \newcommand{\vectorAt}[1]{\vec{\text{#1}}} % arrow\)
\( \newcommand{\vectorB}[1]{\overset { \scriptstyle \rightharpoonup} {\mathbf{#1}} } \)
\( \newcommand{\vectorC}[1]{\textbf{#1}} \)
\( \newcommand{\vectorD}[1]{\overrightarrow{#1}} \)
\( \newcommand{\vectorDt}[1]{\overrightarrow{\text{#1}}} \)
\( \newcommand{\vectE}[1]{\overset{-\!-\!\rightharpoonup}{\vphantom{a}\smash{\mathbf {#1}}}} \)
\( \newcommand{\vecs}[1]{\overset { \scriptstyle \rightharpoonup} {\mathbf{#1}} } \)
\( \newcommand{\vecd}[1]{\overset{-\!-\!\rightharpoonup}{\vphantom{a}\smash {#1}}} \)
Molecular orbital theory is based on the overlap of atomic orbitals, linear combinations of atomic orbitals are taken to form atomic orbitals. At a research level this involves computationally solving the overlap integral between the two or more contributing atomic orbital wavefunctions. In this course we will qualitatively assess orbital overlap primarily through visual inspection.
Sigma (σ) Molecular Orbitals
s orbitals
Similar to valence bond theory, the number of atomic orbitals combines is equal to the number of molecular orbitals formed. Therefore there are two types of molecular orbitals that can form from the overlap of two atomic s orbitals. The two types are illustrated in Figure \(\PageIndex{1}\). The in-phase combination produces a lower energy bonding σs molecular orbital in which most of the electron density is directly between the nuclei. The out-of-phase addition (which can also be thought of as subtracting the wave functions) produces a higher energy antibonding \(σ^∗_s\) molecular orbital in which there is a node between the nuclei. The asterisk signifies that the orbital is an antibonding orbital. Electrons in a σs orbital are attracted by both nuclei at the same time and are more stable (of lower energy) than they would be in the isolated atoms. Adding electrons to these orbitals creates a force that holds the two nuclei together, so we call these orbitals bonding orbitals. Electrons in the \(σ^∗_s\) orbitals are located well away from the region between the two nuclei. The attractive force between the nuclei and these electrons pulls the two nuclei apart. Hence, these orbitals are called antibonding orbitals.

p orbitals
Unlike s orbitals, p orbitals have two lobes with opposite phases, indicated by shading the orbital lobes different colors. When orbital lobes of the same phase overlap, constructive wave interference increases the electron density between the two nuclei. When regions of opposite phase overlap, the destructive wave interference decreases electron density and creates nodes between the two nucei. When p orbitals overlap end to end, they create σ and σ* orbitals. If the two p orbitals are located along the bond axis (assumed to be the z-axis unless otherwise stated), they overlap end to end and form σp (bonding) and \(σ^∗_{p}\) (antibonding) as illustrated in (Figure \(\PageIndex{2}\). Just as with s-orbital overlap, the asterisk indicates the orbital with a node between the nuclei, which is a higher-energy, antibonding orbital.

d orbitals
Recall the shapes and orientations of the five d orbitals from chapter 2. Three of the d orbitals (\(d_{xy}\), \(d_{xz}\), and \(d_{yz}\)) have electron density between the axes and nodes along the axes. Two of the d orbitals (\(d_{z^2}\) and \(d_{x^2-y^2}\)) have electron density along the axes. Only the latter two d orbitals can possibly form sigma bonds. These will be similar to sigma bonds formed from p orbitals, when two lobes of the same phase overlap the electron density between the two nuclei increases and sigma bond is formed as shown in Figure \(\PageIndex{3}\)
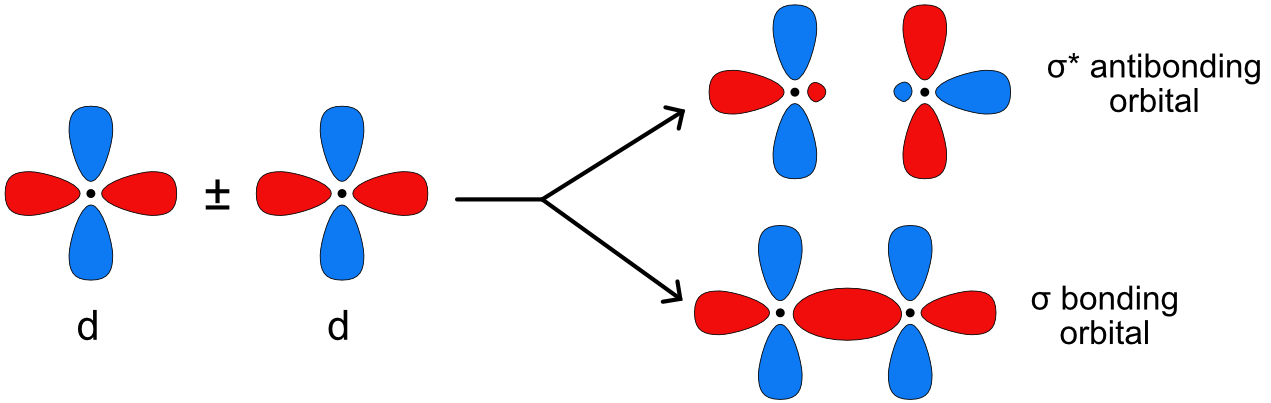
Pi (π) Molecular Orbitals
If two p orbitals are orientated perpendicular to the bond axis they give rise to a pi (\(π\)) bonding molecular orbital and a (\(π^*\)) antibonding molecular orbital, as shown in Figure \(\PageIndex{4}\). The in phase overlap forms a pi bonding orbital, which has increased electron density between the nuclei above and below the internuclear bond axis, with a single node along the bond axis. For the out-of-phase combination, an antibonding orbital is formed with two nodal planes created, one along the internuclear axis and a perpendicular one between the nuclei.

The d orbitals with electron density between the axes (\(d_{xy}\), \(d_{xz}\), and \(d_{yz}\)) can also form pi bonds in the same way that p orbitals can, but an s orbital doesn't have the correct geometry to form pi bonds.
Delta (δ) Molecular Orbitals
In main group chemistry only sigma and pi bonds are possible. When two metal atoms are bonded together, a third type of bond, a delta bond, is possible. Two d orbitals orientated face-to-face along the internuclear bond axis ((\(d_{xy}\) or \(d_{x^2-y^2}\) if the bond is on the z axis) will overlap to form delta bonding and antibonding orbitals as shown in Figure \(\PageIndex{5}\). The in-phase bonding combination has four areas of electron density above and below the two nuclei, with a vertical and a horizontal node along the internuclear bond axis. In the out-of-phase combination there is a third node bisecting the internuclear axis. With delta bonding it it possible to have quadruple or even quintuple bonds between two metal centers, although in practice quintuple bonds are exceedingly rare and have only been observed in a few chromium and molybdenum dimers.

Summary
Bonding orbitals are ones that increase the amount of electron density between the two nuclei relative to the atomic orbitals. Antibonding orbitals are ones that decrease the amount of electron density between the two nuclei relative to the atomic orbitals. Bonding orbitals will have fewer nodes and be lower in energy than antibonding orbitals.A sigma (σ) orbital is one that has no nodes along the internuclear bond axis. Any two orbitals (s, p, or d) that are oriented along the bonding axis can form a sigma bond. A pi (π) orbital is one that has one node containing the internuclear bond axis. A pi bond can be formed from p or d orbitals orientated perpendicular to the bond axis. A delta (δ) orbital is one that has two nodes containing the internuclear bond axis. Only d (or f) orbitals can form delta bonds so they are only possible between two metal atoms.
Multiple Bonds
- Single bonds are formed from 1 sigma bond
- Double bonds are formed from 1 sigma bond and 1 pi bond
- Triple bonds are formed from 1 sigma bond and 2 pi bonds
- Quadruple bonds (only found between two metal atoms) are formed from 1 sigma bond, 2 pi bonds and 1 delta bond
- Quintuple bonds (only found between two metal atoms) are formed from 1 sigma bond, 2 pi bonds and 2 delta bonds
Factors That Influence Covalent Interaction
Now let us refine our understanding of molecular orbitals and molecular orbitals diagrams. Not all atomic orbitals can be combined to form molecular orbitals, and the degree of overlap or covalent interaction between two atomic orbitals can vary lot. What are the criteria according to which we can decide if covalent interaction between two atomic orbitals is possible, and if so how much? There are three criteria to consider; the symmetry criterion, the overlap criterion, and the energy criterion. The symmetry criterion says that If there is a combination of atomic orbitals with bonding and the antibonding interactions that do not cancel out then there is a bonding interaction. The overlap criterion states that the better the atomic orbitals (of appropriate symmetry!) overlap the stronger the covalent interaction. The energy criterion states that the closer the orbitals are in energy the more covalent interaction between them. We will discuss all three criteria in greater detail below.
Criteria for Covalent Interactions
- Symmetry Criterion: If there is a combination of atomic orbitals with bonding and the antibonding interactions that do not cancel out then there is a bonding interaction.
- Overlap Criterion: The better the atomic orbitals overlap the stronger the covalent interaction.
- Energy Criterion: The closer the atomic orbitals in energy the stronger the covalent interaction.
The Overlap Criterion
Let us now look at each criterion in more detail. Let us start with the one we can probably most easily understand, the overlap criterion. The greater the overlap the greater the covalent interaction. The overlap can be estimated according to three rules.
Rule 1: Distance
The first rule says that the overlap is the greater the smaller the distance between the two orbitals. This means that a small distance between the orbital leads to a strongly bonding and a strongly anti-bonding orbital, respectively while a large distance leads to a weakly bonding and a weakly anti-bonding orbital. When the distance is small then there is a large energy difference between the bonding and the anti-bonding molecular orbital, when the distance is large then the energy difference is small (Figure \(\PageIndex{6}\) ).
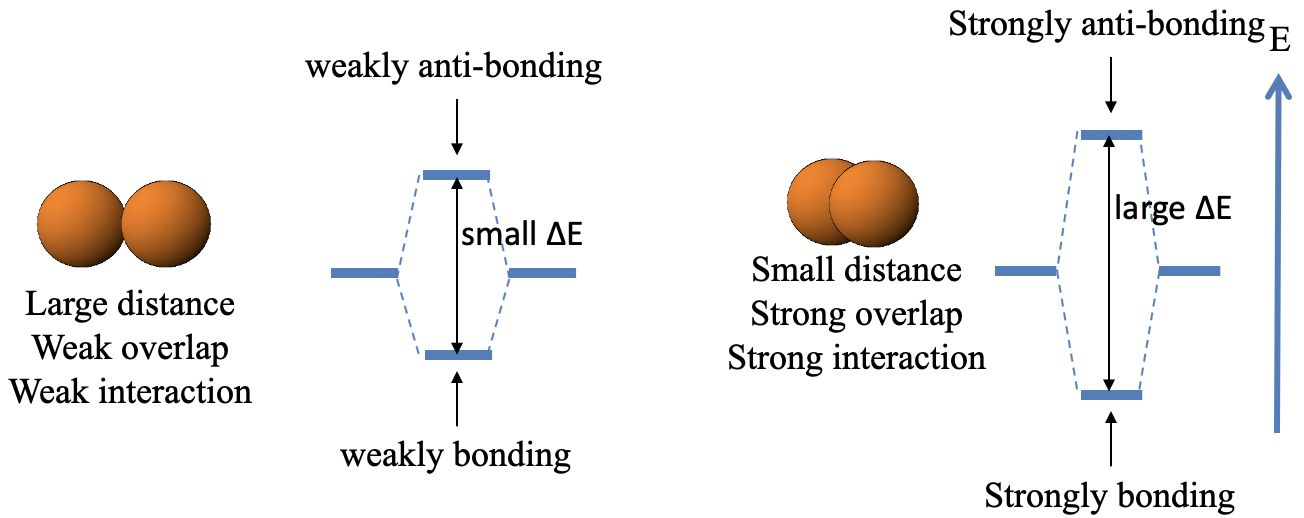
Rule 2: Size
Rule 2 states that a large diffuse orbital tends to overlap better (interact more strongly) with another orbital when this orbital is also a large diffuse orbital. A small, less diffuse orbital tends to interact more strongly with another small orbital. If we combine a large orbital with a small orbital however, then this typically does not lead to good overlap and thus weak interaction. We can qualitatively understand this by looking at the image below (Figure \(\PageIndex{7}\)).

Only a small volume fraction of the large orbital can overlap with the small orbital due to the small size of the small orbital. Due that small overlap the bonding orbital is only weakly bonding, and the anti-bonding is only weakly anti-bonding. The energy difference between the bonding and the anti-bonding MO is small. In the other two cases, the bonding orbitals tend to be strongly bonding, and the anti-bonding ones strongly anti-bonding. The energy differences between the MOs tend to be large.
Rule 3: Orientation
Rule 3 says that that orbitals that overlap in σ-fashion tend to interact more strongly than orbitals that overlap in π-fashion, which interact more strongly than overlap in δ-fashion. One can see easily from the image below that two p orbitals that have the same distance d from each other overlap much more when they overlap in σ-fashion compared to π-fashion (Figure \(\PageIndex{8}\)).
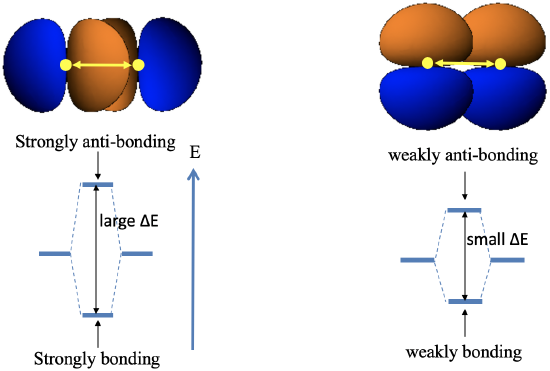
This is because in the first case they point toward each other, and the orbital overlap is on the bond axis, while in the latter case they are oriented parallel to each other, and the orbital overlap is above and below the bond axis. This implies that σ-overlap leads to more strongly bonding and anti-bonding orbitals with a larger energy gap between them compared to π-overlap and π-overlap leads to more strongly bonding and anti-bonding orbitals compared to δ-overlap.
The Energy Criterion
The energy criterion states that the covalent interaction between atomic orbitals is larger the smaller the energy difference is between the atomic orbitals. We can understand this qualitatively when considering that orbitals are waves, and waves of similar energy interfere more significantly with each other than waves with different energies. Just imagine two waves with very different wavelengths associated with very different energies. Would they interfere effectively? No, they wouldn’t. Rather, two waves with very similar wavelengths would interfere better. Because greater energy difference means less interaction, molecular orbitals that result from the interaction of two atomic orbitals with large energy difference are much more similar in shape, size, and location compared to molecular orbitals that result from atomic orbitals with similar energy.
The greatest covalent interaction is expected when the energy between the two orbitals is exactly the same. This is only possible when two, same orbitals A of the two same atoms overlap. In this case we form a perfect covalent bond with electrons exactly equally shared between the orbitals. The maximum of the amplitude of the bonding molecular orbital is exactly in the middle between the two atoms. In the molecular orbital diagram the energy difference between the bonding MO and the atomic orbitals is about the same as the energy difference between the anti-bonding MO and the atomic orbitals. Electrons the bonding (or antibonding) MO are equally shared between the atoms (Figure \(\PageIndex{9}\))

Now let us make the energy of the two atomic orbitals somewhat different. Because they are different we denote the atomic orbitals A and B now, whereby we choose the energy of orbital A to be somewhat higher than that of orbital B. Overlap between the atomic orbital still produces covalent interaction yielding a bonding and an anti-bonding molecular orbital. However, the energy difference of the molecular orbitals to the two atomic orbitals is no longer the same. The antibonding MO is now closer to the atomic orbital with the higher energy, and the bonding MO is now closer in energy to the atomic orbital with the lower energy. This has another consequence. The bonding molecular orbital is now localized primarily at atom A, and the anti-bonding orbital is located primarily at atom B. An electron in the bonding MO is now primarily localized at atom B. This means the bond is a polar covalent bond which is polarized toward atom B (Figure \(\PageIndex{10}\)).

Now let us make the energy difference between the two atomic orbitals of the atoms A and B very large (Figure \(\PageIndex{11}\)). In this case, the bonding MO is energetically very close to the atomic orbital of atom B, and is localized almost exclusively at atom B. Actually, the bonding MO closely resembles the atomic orbital of atom B in shape, size and localization. In other words, the atomic orbital of B has hardly changed due to the very weak covalent interaction resulting from the large energy difference between the atomic orbitals. Vice versa, the antibonding orbital is energetically very close to the atomic orbital of atom A, and is localized almost completely at atom A. The antibonding MO is very close to the atomic orbital in shape, size, and location. Due to the weak covalent interaction, there is almost no change to the atomic orbital of A.

Another conclusion that we can draw is that bonding electrons are located closer to the atom with the atomic orbital of lower energy, and antibonding electrons are located closer to the atoms with the atomic orbital of higher energy. Orbital energy is correlated with electronegativity. For orbitals of the same type and the same elements, orbitals with higher electronegativity have lower energy. For example, a 2s orbital of fluorine has a lower energy than a 2s orbital of oxygen because the electronegativity of fluorine is higher. Bonding electrons are therefore located primarily at the more electronegative atom, while anti-bonding electrons are located primarily at the less electronegative atom. When there are enough anti-bonding orbitals occupied it is possible that the overall polarity in the molecule is such that the dipole moment points toward the more electro-positive atom. An example is carbon monoxide which is slightly polarized toward the carbon atom. We will discuss the MO diagram of the carbon monoxide in detail later.
The Symmetry Criterion
Lastly, let us look at the symmetry criterion. The symmetry criterion tells us if a covalent interaction between orbitals is possible based on the relative orientation of the orbitals. Only if the bonding and antibonding interactions do not cancel out, a bonding interaction is possible, and we can construct molecular orbitals from atomic orbitals. Bonding and antibonding interactions cancel out when positive and negative interferences due to orbital overlap are exactly equal. This can be determined by inspection of orbital overlap.
For example, let us look at the orbital overlap between the 1s orbitals of hydrogen and the 2pz orbital of oxygen in the water molecule (Figure \(\PageIndex{12}\)).
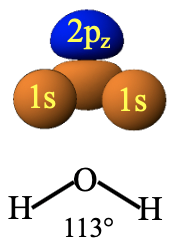
In the water molecule the orbitals are oriented to each other in a specific way because of the bent structure of the water molecule. Due to the bent structure of the water molecule the 1s orbitals overlap differently with the two lobes of the 2pz molecule. The lobe that points downward overlaps more strongly than the lobe that points upward and the two lobes have different phases. Now we choose the phases of 1s orbitals so that bonding is maximized. We can see that the overlap of the 1s with the 2pz orbital produces more constructive than destructive interferences. This is equivalent to saying that bonding and anti-bonding interactions do not cancel out. Therefore, the symmetry is “right”, we can construct molecular orbitals from this combination of atomic orbitals.
Can the 2px orbital of oxygen also combined with the 1s orbitals of the hydrogen atom to form molecular orbitals? The 2px orbital is oriented differently relative to the 1s orbitals in the H2O molecule (Figure \(\PageIndex{13}\)).
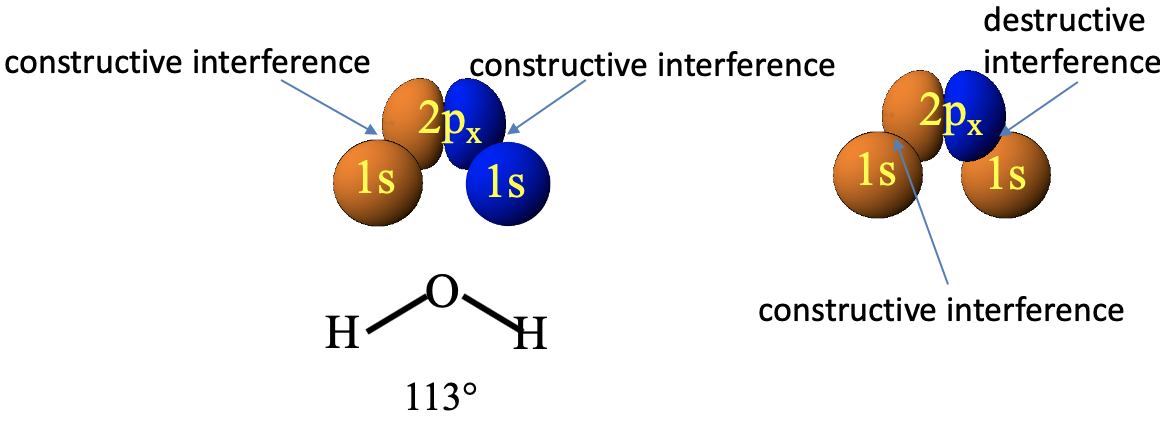
In this case, we must choose the phases of the two 1s orbitals to be different so that bonding interactions are possible. The bonding and the antibonding interactions only do not cancel out if the left 1s orbital has the same phanse as the left lobe of the 2px orbital and the right 1s orbital has the same phase as the right lobe of the 2px orbital. If, for instance, we chose both 1s orbitals to be the same phase then the bonding interactions between the left lobe and the left 1s orbital would be canceled out by the equally strong anti-bonding interactions between the right lobe and the right 1s orbital. Overall, we see however, that if we select the phase of the 1s orbitals appropriately then the symmetry is “right” and we can create molecular orbitals from the atomic orbitals.
What about the interactions between the 1s orbitals and the 2py orbital (Figure \(\PageIndex{14}\))?
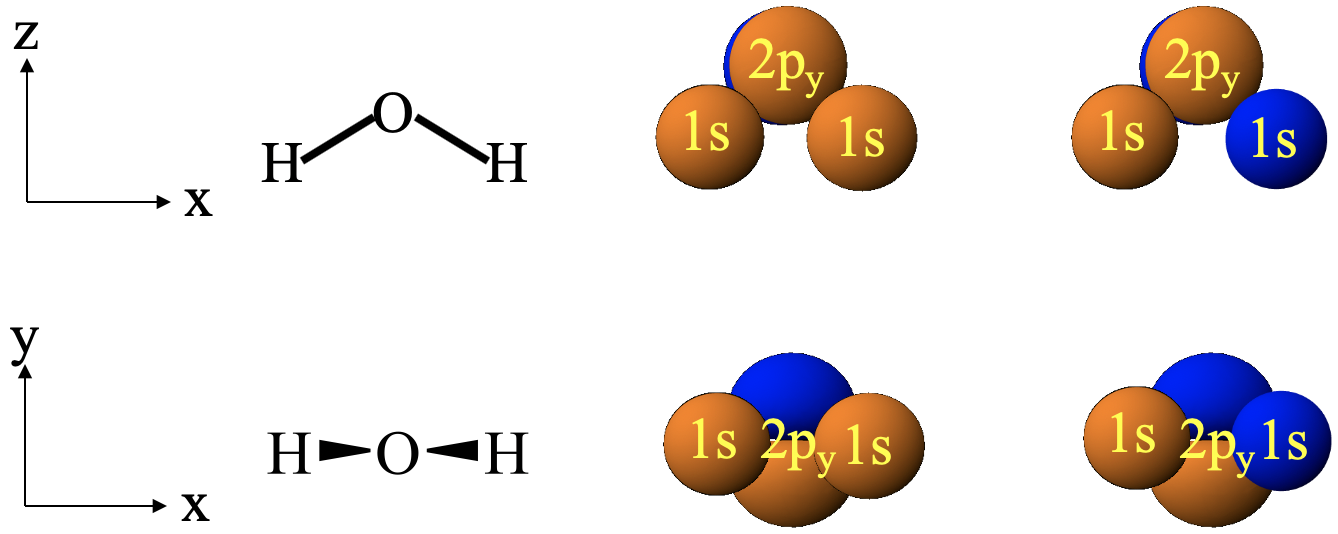
In this case, the two 1s orbitals are in the plane of the page and the 2py orbital stands perpendicular to it. That makes the 1s orbitals overlap equally with both lobes of the 2py orbital. Because the two lobes of a 2py orbital must have different phases, the constructive and the destructive interferences will cancel out, no matter how we chose the phases of our 1s orbitals. That means there is no possibility to create orbital overlap in which bonding an anti-bonding interactions do not cancel out. Therefore, we cannot produce molecular orbitals from a combination of 1s and 2py orbitals. The 2py must remain non-bonding. You may be able to see the cancelation of the bonding and antibonding orbital overlap better if you choose your coordination system differently. Let us have the y-axis point up, and the x-axis point right (Figure \(\PageIndex{9}\), bottom). Now we look at the H2O molecule from the bird perspective, and the 2py orbital is oriented vertically. The 1s orbitals are still on the x-axis. You can see the overlap between the 1s orbitals and the 2py orbital more clearly now. No matter how we choose the phase of our orbitals, the bonding and the anti-bonding interactions cancel out.
We have seen thus far that is is possible to decide about “right” and “wrong” symmetries by inspection, but we have noticed that this is not trivial. Generally, the more complex a molecule gets the more difficult it is to decide about “right” and “wrong” symmetry. In this course we will limit our discussion of molecular orbital theory to cases where we can determine symmetry by visual inspection only. In Advanced Inorganic we will use group theory as a tool to help us decide about “right” and “wrong” symmetry for orbital overlap in more complex molecules.
Example \(\PageIndex{1}\): Molecular Orbitals
Predict what type (if any) of molecular orbital would result from the overlap of each pair of orbitals shown. The orbitals are all similar in energy. Assume the z axis is the bonding axis in all cases.
- s + pz
- px + pz
- dxz + dxz
- (\(d_{xy}\) + (\(d_{xy}\)
Solution
- These both have electron density along the bond axis, so they would form a sigma bonding molecular orbital
- This would be a non-bonding interaction. The px orbital has a node at z axis where it would overlap with the pz orbital. This means there is no net overlap and on bond of any kind is formed.
- These d orbitals are perpendicular to the bond axis, and would therefore form a pi bond.
- These d orbitals are oriented face-to-face along the bond axis, so they would form a delta bonding orbital.
Exercise \(\PageIndex{1}\)
Label the molecular orbital shown as \(σ\),\(π\), or \(δ\), bonding or antibonding, and indicate where any nodes occur.
- Answer
-
The orbital is located along the internuclear axis, so it is a \(σ\) orbital. There is a node bisecting the internuclear axis, so it is an antibonding orbital.
Contributors and Attributions
- Adapted from
Dr. Kai Landskron (Lehigh University). If you like this textbook, please consider to make a donation to support the author's research at Lehigh University: Click Here to Donate.
Paul Flowers (University of North Carolina - Pembroke), Klaus Theopold (University of Delaware) and Richard Langley (Stephen F. Austin State University) with contributing authors. Textbook content produced by OpenStax College is licensed under a Creative Commons Attribution License 4.0 license. Download for free at http://cnx.org/contents/85abf193-2bd...a7ac8df6@9.110).
- Modified by Catherine McCusker (East Tennessee State University)