3.3: Configurations
- Page ID
- 408937
\( \newcommand{\vecs}[1]{\overset { \scriptstyle \rightharpoonup} {\mathbf{#1}} } \)
\( \newcommand{\vecd}[1]{\overset{-\!-\!\rightharpoonup}{\vphantom{a}\smash {#1}}} \)
\( \newcommand{\id}{\mathrm{id}}\) \( \newcommand{\Span}{\mathrm{span}}\)
( \newcommand{\kernel}{\mathrm{null}\,}\) \( \newcommand{\range}{\mathrm{range}\,}\)
\( \newcommand{\RealPart}{\mathrm{Re}}\) \( \newcommand{\ImaginaryPart}{\mathrm{Im}}\)
\( \newcommand{\Argument}{\mathrm{Arg}}\) \( \newcommand{\norm}[1]{\| #1 \|}\)
\( \newcommand{\inner}[2]{\langle #1, #2 \rangle}\)
\( \newcommand{\Span}{\mathrm{span}}\)
\( \newcommand{\id}{\mathrm{id}}\)
\( \newcommand{\Span}{\mathrm{span}}\)
\( \newcommand{\kernel}{\mathrm{null}\,}\)
\( \newcommand{\range}{\mathrm{range}\,}\)
\( \newcommand{\RealPart}{\mathrm{Re}}\)
\( \newcommand{\ImaginaryPart}{\mathrm{Im}}\)
\( \newcommand{\Argument}{\mathrm{Arg}}\)
\( \newcommand{\norm}[1]{\| #1 \|}\)
\( \newcommand{\inner}[2]{\langle #1, #2 \rangle}\)
\( \newcommand{\Span}{\mathrm{span}}\) \( \newcommand{\AA}{\unicode[.8,0]{x212B}}\)
\( \newcommand{\vectorA}[1]{\vec{#1}} % arrow\)
\( \newcommand{\vectorAt}[1]{\vec{\text{#1}}} % arrow\)
\( \newcommand{\vectorB}[1]{\overset { \scriptstyle \rightharpoonup} {\mathbf{#1}} } \)
\( \newcommand{\vectorC}[1]{\textbf{#1}} \)
\( \newcommand{\vectorD}[1]{\overrightarrow{#1}} \)
\( \newcommand{\vectorDt}[1]{\overrightarrow{\text{#1}}} \)
\( \newcommand{\vectE}[1]{\overset{-\!-\!\rightharpoonup}{\vphantom{a}\smash{\mathbf {#1}}}} \)
\( \newcommand{\vecs}[1]{\overset { \scriptstyle \rightharpoonup} {\mathbf{#1}} } \)
\( \newcommand{\vecd}[1]{\overset{-\!-\!\rightharpoonup}{\vphantom{a}\smash {#1}}} \)
\(\newcommand{\avec}{\mathbf a}\) \(\newcommand{\bvec}{\mathbf b}\) \(\newcommand{\cvec}{\mathbf c}\) \(\newcommand{\dvec}{\mathbf d}\) \(\newcommand{\dtil}{\widetilde{\mathbf d}}\) \(\newcommand{\evec}{\mathbf e}\) \(\newcommand{\fvec}{\mathbf f}\) \(\newcommand{\nvec}{\mathbf n}\) \(\newcommand{\pvec}{\mathbf p}\) \(\newcommand{\qvec}{\mathbf q}\) \(\newcommand{\svec}{\mathbf s}\) \(\newcommand{\tvec}{\mathbf t}\) \(\newcommand{\uvec}{\mathbf u}\) \(\newcommand{\vvec}{\mathbf v}\) \(\newcommand{\wvec}{\mathbf w}\) \(\newcommand{\xvec}{\mathbf x}\) \(\newcommand{\yvec}{\mathbf y}\) \(\newcommand{\zvec}{\mathbf z}\) \(\newcommand{\rvec}{\mathbf r}\) \(\newcommand{\mvec}{\mathbf m}\) \(\newcommand{\zerovec}{\mathbf 0}\) \(\newcommand{\onevec}{\mathbf 1}\) \(\newcommand{\real}{\mathbb R}\) \(\newcommand{\twovec}[2]{\left[\begin{array}{r}#1 \\ #2 \end{array}\right]}\) \(\newcommand{\ctwovec}[2]{\left[\begin{array}{c}#1 \\ #2 \end{array}\right]}\) \(\newcommand{\threevec}[3]{\left[\begin{array}{r}#1 \\ #2 \\ #3 \end{array}\right]}\) \(\newcommand{\cthreevec}[3]{\left[\begin{array}{c}#1 \\ #2 \\ #3 \end{array}\right]}\) \(\newcommand{\fourvec}[4]{\left[\begin{array}{r}#1 \\ #2 \\ #3 \\ #4 \end{array}\right]}\) \(\newcommand{\cfourvec}[4]{\left[\begin{array}{c}#1 \\ #2 \\ #3 \\ #4 \end{array}\right]}\) \(\newcommand{\fivevec}[5]{\left[\begin{array}{r}#1 \\ #2 \\ #3 \\ #4 \\ #5 \\ \end{array}\right]}\) \(\newcommand{\cfivevec}[5]{\left[\begin{array}{c}#1 \\ #2 \\ #3 \\ #4 \\ #5 \\ \end{array}\right]}\) \(\newcommand{\mattwo}[4]{\left[\begin{array}{rr}#1 \amp #2 \\ #3 \amp #4 \\ \end{array}\right]}\) \(\newcommand{\laspan}[1]{\text{Span}\{#1\}}\) \(\newcommand{\bcal}{\cal B}\) \(\newcommand{\ccal}{\cal C}\) \(\newcommand{\scal}{\cal S}\) \(\newcommand{\wcal}{\cal W}\) \(\newcommand{\ecal}{\cal E}\) \(\newcommand{\coords}[2]{\left\{#1\right\}_{#2}}\) \(\newcommand{\gray}[1]{\color{gray}{#1}}\) \(\newcommand{\lgray}[1]{\color{lightgray}{#1}}\) \(\newcommand{\rank}{\operatorname{rank}}\) \(\newcommand{\row}{\text{Row}}\) \(\newcommand{\col}{\text{Col}}\) \(\renewcommand{\row}{\text{Row}}\) \(\newcommand{\nul}{\text{Nul}}\) \(\newcommand{\var}{\text{Var}}\) \(\newcommand{\corr}{\text{corr}}\) \(\newcommand{\len}[1]{\left|#1\right|}\) \(\newcommand{\bbar}{\overline{\bvec}}\) \(\newcommand{\bhat}{\widehat{\bvec}}\) \(\newcommand{\bperp}{\bvec^\perp}\) \(\newcommand{\xhat}{\widehat{\xvec}}\) \(\newcommand{\vhat}{\widehat{\vvec}}\) \(\newcommand{\uhat}{\widehat{\uvec}}\) \(\newcommand{\what}{\widehat{\wvec}}\) \(\newcommand{\Sighat}{\widehat{\Sigma}}\) \(\newcommand{\lt}{<}\) \(\newcommand{\gt}{>}\) \(\newcommand{\amp}{&}\) \(\definecolor{fillinmathshade}{gray}{0.9}\)- understand the meaning of absolute configuration, chiral centers, and draw perspective drawings and Fisher projections to represent chiral compounds.
- Apply rules to assigning D/L or R/S stereodescriptors to the chiral compounds containing one or two chiral centers.
- Understand optical activity as a physical characteristic of chiral compounds and the factors that affect it.
- Recognize stereochemical relationships between organic compounds, including enantiomers, diastereomers, and meso.
What is a configuration?
A molecule's permanent geometry that results from its bonds' spatial arrangement is called configuration. Stereoisomers have different configurations for the same set of atoms and the same group of bonds. For example, cis-but-2-ene has a different configuration than its stereoisomer trans-2-butene, shown in Figure 3.1.1.
Representing a configurations
Representing configuration around a single atom will be described first. An sp-hybridized atom has a linear geometry, as in the case of hydrogen cyanide \(\ce{H-C≡N}\) or carbon dioxide \(\ce{O=C=O}\) molecules. An sp2-hybridized atom is a trigonal planer, i.e., there are three \(\sigma\)-bonds at 60o to each other in the same plane and one \(\pi\)-bond with one of the \(\sigma\)-bond, as in the case of formaldehyde \(\ce{H2C=O}\) represented as . An sp3-hybridized atom is tetrahedral, i.e., four \(\sigma\)-bonds as two V's of 109o internal angle joined perpendicular to each other at vortexes, e.g., methane \(\ce{CH4}\) modeled as
. Tetrahedral geometry is a 3D geometry usually represented by perspective drawings or Fisher projections, described in the next sections.
Perspective drawing
Since the tetrahedral geometry is two V's perpendicular to each other, one V is placed in the plane of the screen (or page) and represented by solid lines. The other V is represented by a solid wedge representing the bonding coming out of the screen towards the viewer and a hashed wedge representing the bond going beyond the screen away from the viewer, as illustrated below for the case of two configurations of 2-chlorobutane. The view angle is from the direction of the top-left corner. The V in the plane of the screen appears larger and the other appears smaller. Usually, the solid wedge is near the viewer relative to the hashed wedge.
If the configuration of two or more connected \(\ce{C's}\) needs to be shown, the V's in the plane of the screen is usually drawn pointing downwards or upwards, making a zig-zag line as illustrated below for the case of two configurations of 2-chlorobutane.
In the case of skeletal formulas, \(\ce{C's}\) and \(\ce{H's}\) are not shown. However, the configuration is still readable correctly from the bonds shown, as illustrated below for the case of two configurations of 2-chlorobutane.
Fisher projections
Fisher projections represent the configuration as a projection of the molecule in 2D with four bonds in the shape of a cross, as explained in Figure \(\PageIndex{1}\).

Examples of Fisher projections of the two configurations of 2-chlorobutane are shown below, along with the perspective drawings.
When more than one consecutive \(\ce{C's}\) needs to be shown by a Fisher projection, the \(\ce{C}\) chain, i.e., the skeleton, is shown vertically. For this purpose, \(\ce{C-C}\) single bond is rotated by 180o resulting in a semi-cyclic conformation. Then the \(\ce{C's}\) of interest are viewed from the edge-on so that the horizontal bonds are pointing towards the viewer and drawn vertically from top to bottom, as illustrated Figure \(\PageIndex{2}\).


Chiral center
A compound with sp-, sp2-hybridized central atom, or a sp3-hybridized central atom with two or more same groups have a superimposable mirror image, as illustrated in Figure \(\PageIndex{3}\). Since the compound and its mirror image are identical in these cases, these compounds are achiral, i.e., not chiral.
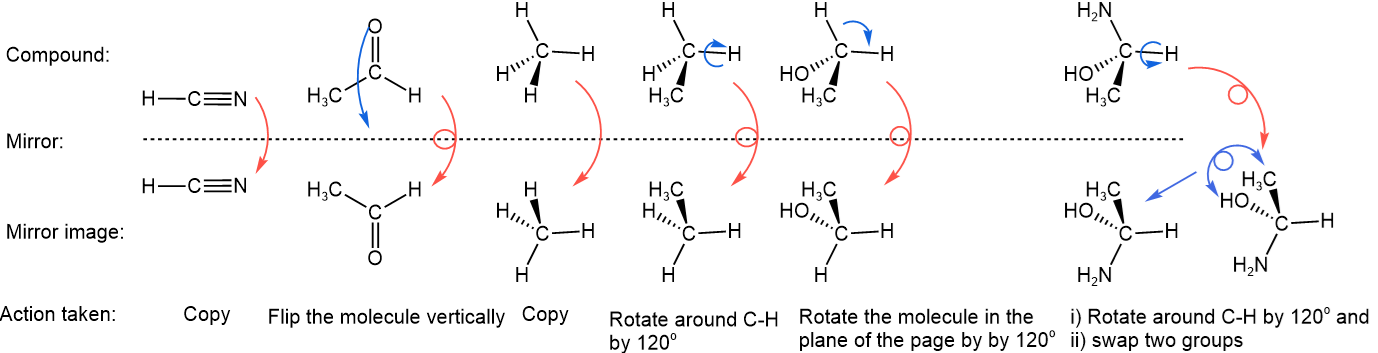
A compound with four different groups attached to an sp3-hybridized central atom has two distinct configurations, which are related as non-superimposable mirror images of each other, i.e., chiral. Since the two configurations represent different compounds that are stereoisomers, making and breaking of bonds, i.e., a chemical change, is needed to inter-convert them, as illustrated in Figure \(\PageIndex{3}\).
An atom, often a \(\ce{C}\) with four different groups attached to it, is called a chiral center.
A chiral center is often a cause of the chirality of the molecule. A stereochemical descriptor, e.g., D or L and R or S, is used to distinguish the two configurations of a chiral center.
An absolute configuration is the spatial arrangement of the atoms of a chiral molecular entity specified by a stereochemical descriptor.
A stereocenter is an atom, axis, or plane with at least three different groups attached to it, so interchanging any two groups creates a new stereoisomer. The chiral center is a sub-class stereocenter, as illustrated in Figure \(\PageIndex{3}\). The \(\ce{C's}\) of the \(\ce{C=C}\)-bond of cis-but-2-ene and trans-but-2-ene shown in Figure 3.1.1, have three different groups and they are sterocenters. For example, swapping \(\ce{-CH3}\) and \(\ce{-H}\) on any of the two \(\ce{C's}\) converts cis-but-2-ene to its stereoisomer trans-but-2-ene and vice versa.
Stereochemical descriptors
Two sets of stereochemical descriptors, i.e., D or L and R or S, are in common use and described next.
D/L Stereochemical descriptors
The D and L stereochemical descriptors were developed for monosaccharides, i.e., sugars. The monosaccharides have an aldehyde or a ketone group placed on the top or near the top in Fisher projections. Every other \(\ce{C}\) usually has an alcohol (\(\ce{-OH}\)) group on them, and they are chiral except the terminal \(\ce{C's}\), which are achiral.
Looking at the bottom-most chiral \(\ce{C}\) in the Fisher projection of a monosaccharide, if the \(\ce{-OH}\) group is towards the right of the Fisher projection, it is designated D and if it is towards the left, it is designated L, as illustrated in Figure \(\PageIndex{4}\).
A hyphen separates the name of the monosaccharide from the D or L stereodescrptor. The D or L defines the absolute configuration of the bottom-most chiral \(\ce{C}\) and the name that follows it defines the absolute configuration of all other chiral \(\ce{C's}\) in a monosaccharide.
Amino acids have a chiral (\ce{C}\) with an amine (\(\ce{-NH2}\)) and a carboxylic acid (\(\ce{-COOH}\)) attached to it. The \(\ce{-COOH}\) group is placed on the top and the \(\ce{-NH2}\) group is either on the left or right side of the Fisher projection.
If the \(\ce{-NH2}\) group is towards the right of the Fisher projection of an amino acid it is designated D. If it is towards the left, it is designated L, as illustrated in Figure \(\PageIndex{4}\).
- Natural monosaccharides (sugars) have D-configurations, and natural amino acids have L-configurations with few exceptions.
- Although the common names of monosaccharides and amino acids with a D or L descriptor are not IUPAC names, these are commonly used in biochemistry.
R/S Stereochemical descriptors
The R and S stereochemical descriptors are part of the IUPAC nomenclature. For this purpose, the four groups attached to the chiral center are assigned priority 1, 2, 3, and 4, where 1 is the highest priority and 4 is the lowest. Then R or S is assigned based on the groups' relative orientations, considering the priority order.
Sequence rules
The following is the sequence rules, also called Cahn-Ingold-Prelog (CIP) sequence rules for assigning priority.
- A group attached to a stereocenter through an atom of a higher atomic number (Z) has higher priority. For example, the priority of the four groups in
is: \(\overleftarrow{\ce{-OH > -NH2 > -CH3 > -H}}\).
- If there is a tie, look at atoms two bonds away from the stereocenter. List them according to their atomic number (Z) and compare them, one by one, starting from the highest atomic number. Stop at the first point of difference and assign higher priority to the higher atomic number at the first point of difference. For example, in
there is a tie between \(\ce{-CH3}\) and \(\ce{-CH2-CH3}\) due to both having \(\ce{C}\) at one bond away from chiral center. Comparison of atoms two bonds away: \(\overset{\large{H, H, H}}{\small{C, H, H}}\) assigns higher priority to \(\ce{-CH2-CH3}\) over \(\ce{-CH3}\), i.e., the priority order is: \(\overleftarrow{\ce{-OH > -CH2-CH3 > -CH3 > -H}}\).
- If the tie does not break at the distance of two bonds, move to atoms at the distance of three bonds from the chiral center and repeat the process until the first point of difference arrives.
Atom bonded by a double bond is treated as connected twice, and by a triple bond is treated as connected three times. For example, \(\ce{-CH=CH2}\) and \(\ce{-C#CH}\) are the same for the atom directly attached to the chiral center. For comparing atoms two bonds away, the groups are treated as explained in the illustration below, with the first point of difference highlighted in red.
The rule is to stop at the first point of difference, even if the order may change later, as illustrated below with the first point of difference highlighted in red.
Assigning R or S to the chiral center
After all four groups on the chiral center have been assigned priority numbers 1, 2, 3, and 4, two situations arise:
- Case 1: The lowest priority, i.e., #4, is pointing away from the viewer, i.e., it is on a hashed line in the perspective drawing or on a vertical line in Fisher projection. In this case, R (rectus, Latin for right) is assigned if an arrow drawn from #1 to #2 to #3 is clockwise or directed to the right, and S (sinister, Latin for left) is assigned if it is directed to the left or counterclockwise, as illustrated in Figure \(\PageIndex{5}\).
- Case 2: The lowest priority, i.e., #4 is not pointing away from the viewer. In this case,
- swap #4 with the group pointing away from the viewers,
- since a swap of any two groups changes the configuration, reverse the assignments, i.e., assign R if an arrow drawn from #1 to #2 to #3 is clockwise or directed to the right and S if it is directed to the left or counterclockwise, as illustrated in Figure \(\PageIndex{5}\).
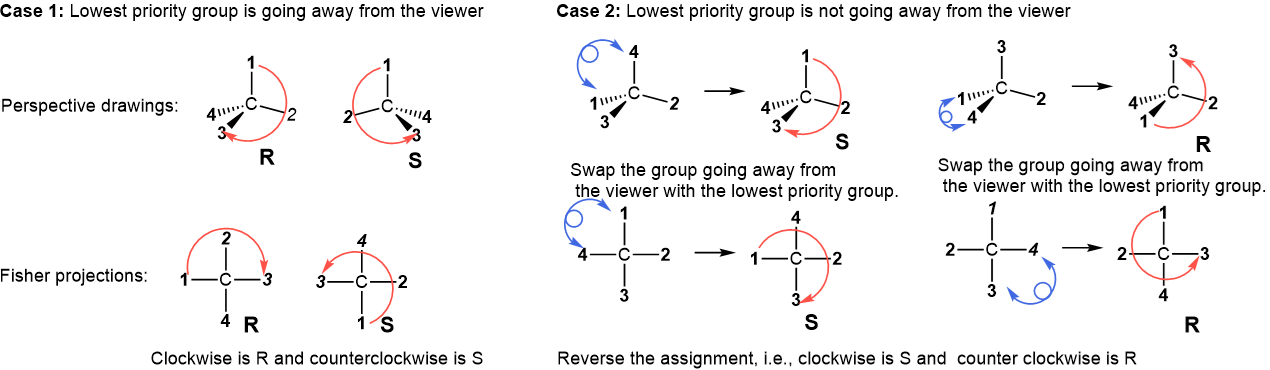
The stereochemical descriptor R or S describes the absolute configuration of the chiral center. They are placed within small brackets at the beginning of the IUPAC name, separated by a hyphen, as shown in the examples below. If there is more than one chiral center in a compound, the locant number precedes each chiral center's R or S descriptor, as explained in the examples below.
Assign R/S to the compound shown on the right.
Solution
- Assign priority based on the atomic number of atoms attached to the chiral center: \(\ce{-Br}\) > \(\ce{-NH2}\) > \(\ce{-CH3}\) > \(\ce{-H}\).
Swap #4 with the group away from the viewer (hashed wedge). No action needed as \(\ce{-H}\) is already at the hashed wedge.
- Draw an arrow from #1 to #2 to #3. The arrow is counterclockwise, i.e., the stereodescriptor is S.
Answer: S
Assign R/S to the compound shown on the right.
Solution
- Assign priority based on the atomic number of atoms attached to the chiral center: \(\ce{-OH}\) is #1, there is a tie between \(\ce{-CH3}\) and \(\ce{-COOH}\), and \(\ce{-H}\) is #4.
- Tiebreaker is \(\ce{O}\) two bonds away \(\overset{\large{O, O, O}}{\small{H, H, H}}\), assigning #2 to \(\ce{-COOH}\) and #3 to \(\ce{-CH3}\).
-
Swap #4 with the group away from the viewer (hashed wedge), i.e., \(\ce{-OH}\) with \(\ce{-H}\) in this case.
- Draw an arrow from #1 to #2 to #3. The arrow is counterclockwise. Since two groups were swapped, reverse the assignment i.e., counterclockwise is R.
Answer: R
When the lowest priority group #4 is on the vertical line in the Fisher projection, as in or
, there is no need to swap to bring it on the vertical line. Draw the arrow from #1 to #2 to #3 and reverse the assignments, i.e., assign S if the arrow is clockwise and R if it is counterclockwise. This trick also works when the lowest priority group #4 is on the solid wedge in the perspective drawing, as in
. This trick makes the R/S assignment easy, especially for complex organic compounds where redrawing the structure after the swap is not easy.
Do not try this trick when the lowest priority group #4 is in the plane of the page in the perspective drawing, as in or
. These two structures have opposite configurations, but the arrow drawn from #1 to #2 to #3 is in the same direction for both. It will lead to an incorrect assignment in one of the two.
Assign R/S to the compound shown on the right.
Solution
- Assign priority based on the atomic number of atoms attached to the chiral center: \(\ce{-Cl}\) is #1, there is a tie between \(\ce{-CH3}\) and \(\ce{-CH2CH3}\), and \(\ce{-H}\) is #4.
- Tiebreaker is \(\ce{C}\) two bonds away \(\overset{\large{C, H, H}}{\small{H, H, H}}\), assigning #2 to \(\ce{-CH2CH3}\) and #3 to \(\ce{-CH3}\).
-
Swap #4 with the group away from the viewer (hashed wedge). Trick: No need for Fisher projection, as the assignment can be reversed in the last step.
- Draw an arrow from #1 to #2 to #3. The arrow is clockwise. Since the lowest priority group is on vertical line, reverse the assignment i.e., clockwise is S.
Answer: S
Assign R/S to the compound shown on the right.
Solution
- Assign priority based on the atomic number of atoms attached to the chiral center: \(\ce{-NH2}\) is #1, there is a tie between \(\ce{-C(CH3)2SH}\) and \(\ce{-COOH}\), and \(\ce{-H}\) is #4.
- Tiebreaker is \(\ce{S}\) two bonds away \(\overset{\large{S, C, C}}{\small{O, O, O}}\), assigning #2 to \(\ce{-C(CH3)2SH}\) and #3 to \(\ce{-COOH}\).
-
Swap #4 with the group away from the viewer (hashed wedge). Trick: No need for perspective drawing with the lowest priority group on a solid wedge, as the assignment can be reversed in the last step.
- Draw an arrow from #1 to #2 to #3. The arrow is clockwise. Since the lowest priority group is on the solid wedge, reverse the assignment i.e., clockwise is S.
Answer: S (This compound is (S)-penicillamine)
Assign R/S to the compound shown on the right.
Solution
- Assign priority based on the atomic number of atoms attached to the chiral center: \(\ce{-NH2}\) is #1, there is a tie between \(\ce{-C(CH3)2SH}\) and \(\ce{-COOH}\), and \(\ce{-H}\) is #4.
- Tiebreaker is \(\ce{S}\) two bonds away \(\overset{\large{S, C, C}}{\small{O, O, O}}\), assigning #2 to \(\ce{-C(CH3)2SH}\) and #3 to \(\ce{-COOH}\).
Swap #4 with the group away from the viewer (hashed wedge). Not needed as the lowest priority group is already away from the viewer on hashed wedge.
- Draw an arrow from #1 to #2 to #3. The arrow is clockwise. Clockwise is R.
Answer: R (This compound is (R)-penicillamine)
Compounds with one chiral center
Compounds containing one chiral center are chiral. They have a stereoisomer called enantiomer, which is their non-superimposable mirror image. Some examples of compounds containing one chiral center, their enantiomers with their absolute configuration assigned by D/L system and R/S system, and their tetetrahederal geometry illustrated are shown in Figure \(\PageIndex{6}\).
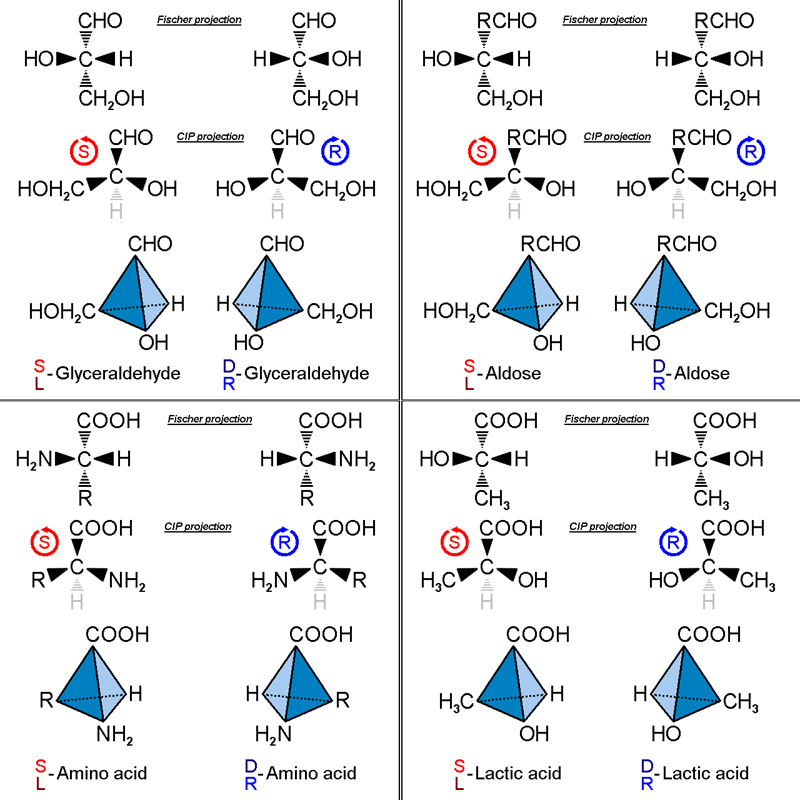
Enantiomers have the same physical and chemical properties, except when they react with or interact with other chiral objects or molecules.
For example, hands and gloves are chiral objects. The right-hand glove fits on the right hand, and the left-hand fits on the left. Similarly, enantiomer molecules react equally and when they are produced in a chemical reaction they are produced equally, except when one of the reagents is chiral. For example, enzymes are chiral reagents and usually react with one of the two enantiomers in the reactants and/or produce one of the two enantiomers.
Optical activity
The light has an electric field oscillating perpendicular to the direction of propagation of the light. Ordinary light has an electric field oscillating in all places perpendicular to the direction of propagation. When it passes through a polarizer, it transmits only the waves oscillating in one plane, i.e., plane polarized light, as illustrated in Figure \(\PageIndex{7}\). The plane polarized light is composed of left-handed and right-handed circularly polarized light, which are non-superimposable mirror images of each other, i.e., chiral, as shown in Figure \(\PageIndex{7}\).


When the plane-polarized light passes through a solution of chiral molecules, one enantiomer absorbs more right-handed and the other more left-handed component. Therefore, if the solution is of a pure enantiomer, the plane of the plane-polarized light rotates clockwise or counterclockwise. The enantiomer that rotates the plane polarized clockwise is called dextrorotatory (abbreviated as d or (+)), and the other that rotates it counterclockwise is called levorotatory (abbreviated as l or (-)).
- The ability of chiral compounds to rotate the plane of plane-polarized light is called optical activity.
- Chiral compounds are optically active, and achiral compounds are optically inactive.
- An instrument used to measure the ability of a compound to rotate the plane of a polarized light is called a polarimeter, illustrated in Figure \(\PageIndex{8}\).
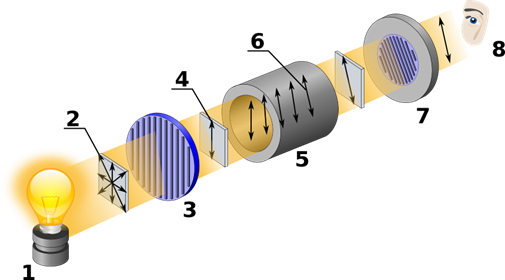
The rotation of the plane of plane-polarized light by an enantiomer is called observed rotation, measured in degrees. The observed rotation depends on the concentration of the sample, the path length of the light through the sample, the wavelength of the light used, and temperature.
When the concentration is fixed to 1 g/mL (or density of the pure sample in g/mL) and path length to 1 dm, the observed rotation is specific rotation [\(\alpha\)].
Usually, the wavelength (\(\lambda\)) is 589 nm, i.e., sodium D light and temperature (T) is room temperature and shown as subscript and superscrpt: \([\alpha]^{T}_{\lambda}\), as in the following formula.
\[[\alpha]^{T}_{\lambda} = \frac{\text{Observed rotation (in degree)}}{\text{Path length (dm)}\times\text{Concentration (g/mL)}} \nonumber \]
The specific rotation is a characteristic physical property of chiral compounds. If one enantiomer is dextrorotatory, the other is leavorotatory to the same degree, as shown in the following examples.
S and R define the absolute configuration of the chiral center, and dextrorotatory (+) or levorotatory (-) is an experimental property of the compound. A compound with S configuration may be dextrorotatory (+) or levorotatory (-), the same for R configuration. For example, (S)-lactic acid is dextrorotatory (+), and its salt (S)-sodium lactate is levorotatory (-), as shown below.
Mixing a chiral compound with its enantiomer diminishes its optical activity. A 50:50 mixture of enantiomers is optically inactive called a racemic mixture.
Compounds with more than one chiral centers
The maximum number of stereoisomers possible for chiral compounds is given by the formula 2n, where n is the number of chiral centers. For example, glyceraldehyde shown in Figure 3.1.1 has one chiral center and (21= 2) two stereoisomers: D-glyceraldehyde and L-glyceraldehyde. Erythrose, i.e., another monosaccharide, has two chiral centers and (22= 4) four stereoisomers, as shown in Figure \(\PageIndex{9}\).
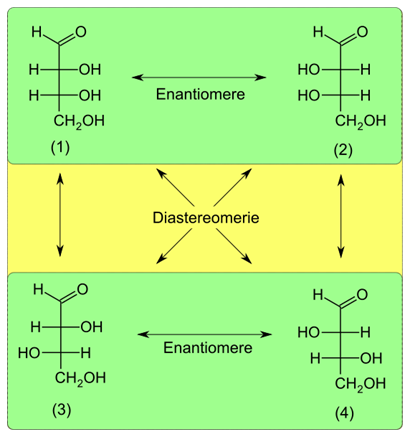
D-erythrose and L-erythrose are enantiomers, and D-threose and L-threose are also enantiomers, but erythrose is not an enantiomer of threose.
Stereoisomers that are not enantiomers are diastereomers of each other. For example, D-erythrose is a diastereomer of D-threose and L-threose, as illustrated in Figure \(\PageIndex{9}\).
The formula 2n tells the maximum possible number of stereoisomers of compounds having n number of chiral centers. The actual number may be less than the maximum. For example, tartaric acid has two chiral centers and three stereoisomers: (+) tartaric acid and (-) tartaric acid, which are enantiomers and achiral stereoisomers that is diastereomers of the first two, as illustrated in Figure \(\PageIndex{10}\). The third isomer is achiral because its mirror image is identical, simply rotated by a 180o.
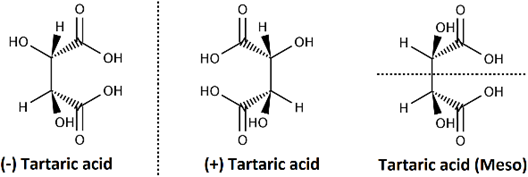
A meso compound is an achiral compound with two or more chiral centers. A meso compound has a plane of symmetry that divides into two mirror-image halves, as illustrated in Figure \(\PageIndex{10}\).
Enantiomers have the same physical and chemical properties, except when interacting with chiral objects or reagents. Diastereomers have different physical and chemical properties, as can be observed in Table 1.
Stereoisomer | (+)-Tartaric acid | (-)-Tartaric acid | Meso tartaric acid |
---|---|---|---|
Specific rotation | +12.7 | -12.7 | 0 |
Melting point (oC) | 171-174 | 171-174 | 146-148 |
Density at 20oC (g/mL) | 1.7598 | 1.7598 | 1.660 |
Solubility in water at 20oC (g/100 mL) | 139 | 139 | 125 |
\(\ce{pK_{1}}\) at 25oC | 2.98 | 2.98 | 3.23 |
\(\ce{pK_{2}}\) at 25oC | 4.34 | 4.34 | 4.82 |
Chirality in life
A large number of compounds in living things are chiral. For example, amino acids and carbohydrates are chiral. Macro-molecules like proteins and nucleic acids are also chiral. Chirality is also observed on a macro-scale, e.g., our hands, foot, and ears are chiral. Similarly, horns and spots in axis deer are chiral, as shown in Figure \(\PageIndex{11}\).
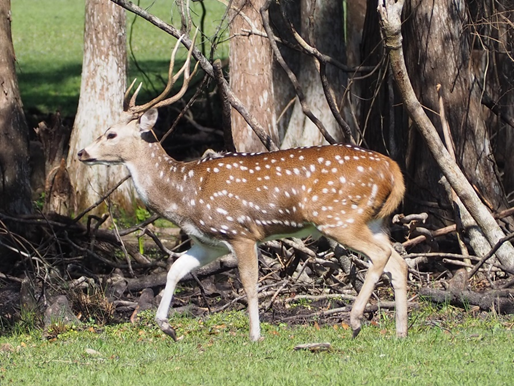
Stereospecific reactions in living things
Most chemical reactions in living things are stereospecific, i.e., one stereoisomer selectively reacts, and one is selectively produced. For example, the human digestion enzyme chymotrypsin has 268 chiral centers and 2268 possible stereoisomers, but only one isomer is produced. This is because amino acids that constitute chymotrypsin and other enzymes have L-configuration. The enzyme-catalyzed reactions are often stereospecific because their binding sites are chiral, and only one enantiomer reactant fits in them nicely for the reaction, as illustrated in Figure \(\PageIndex{12}\). Similarly, one specific surface of enzyme-bound substrates is exposed for the reaction that results in the selective production of one enantiomer.
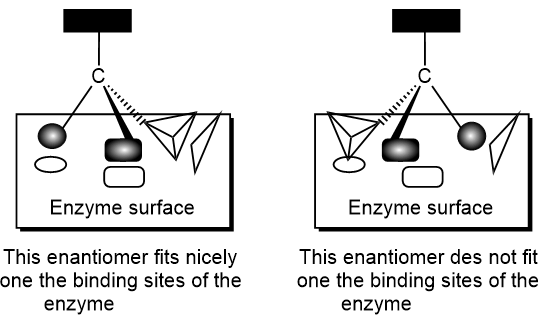
Chirality manifests in taste, flavor, odor, and drug action as their receptors have chiral binding sites, as illustrated in Figure \(\PageIndex{12}\). Examples include:
- L-aspartame tastes sweet and is used as an artificial sweetener but D-aspartame is tasteless,
- R-(-)-carvone smells like spearmint, and S-(+)-carvone smells like caraway,
- R-(+)-limonene smells like orange and lemon, and S-(-)-limonene smells like a spruce tree,
- (S)-penicillamine has antiarthritic activity, and (R)-penicillamine is toxic, and
- S-ibuprofen has anti-inflammatory action, and R-ibuprofen is inactive.

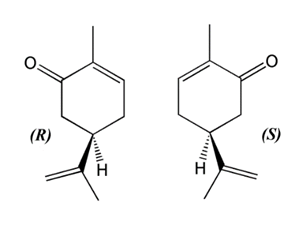
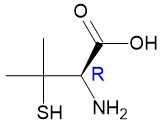


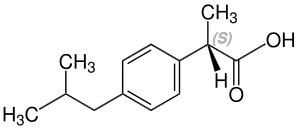