Chromatographic Columns
- Page ID
- 299
\( \newcommand{\vecs}[1]{\overset { \scriptstyle \rightharpoonup} {\mathbf{#1}} } \)
\( \newcommand{\vecd}[1]{\overset{-\!-\!\rightharpoonup}{\vphantom{a}\smash {#1}}} \)
\( \newcommand{\id}{\mathrm{id}}\) \( \newcommand{\Span}{\mathrm{span}}\)
( \newcommand{\kernel}{\mathrm{null}\,}\) \( \newcommand{\range}{\mathrm{range}\,}\)
\( \newcommand{\RealPart}{\mathrm{Re}}\) \( \newcommand{\ImaginaryPart}{\mathrm{Im}}\)
\( \newcommand{\Argument}{\mathrm{Arg}}\) \( \newcommand{\norm}[1]{\| #1 \|}\)
\( \newcommand{\inner}[2]{\langle #1, #2 \rangle}\)
\( \newcommand{\Span}{\mathrm{span}}\)
\( \newcommand{\id}{\mathrm{id}}\)
\( \newcommand{\Span}{\mathrm{span}}\)
\( \newcommand{\kernel}{\mathrm{null}\,}\)
\( \newcommand{\range}{\mathrm{range}\,}\)
\( \newcommand{\RealPart}{\mathrm{Re}}\)
\( \newcommand{\ImaginaryPart}{\mathrm{Im}}\)
\( \newcommand{\Argument}{\mathrm{Arg}}\)
\( \newcommand{\norm}[1]{\| #1 \|}\)
\( \newcommand{\inner}[2]{\langle #1, #2 \rangle}\)
\( \newcommand{\Span}{\mathrm{span}}\) \( \newcommand{\AA}{\unicode[.8,0]{x212B}}\)
\( \newcommand{\vectorA}[1]{\vec{#1}} % arrow\)
\( \newcommand{\vectorAt}[1]{\vec{\text{#1}}} % arrow\)
\( \newcommand{\vectorB}[1]{\overset { \scriptstyle \rightharpoonup} {\mathbf{#1}} } \)
\( \newcommand{\vectorC}[1]{\textbf{#1}} \)
\( \newcommand{\vectorD}[1]{\overrightarrow{#1}} \)
\( \newcommand{\vectorDt}[1]{\overrightarrow{\text{#1}}} \)
\( \newcommand{\vectE}[1]{\overset{-\!-\!\rightharpoonup}{\vphantom{a}\smash{\mathbf {#1}}}} \)
\( \newcommand{\vecs}[1]{\overset { \scriptstyle \rightharpoonup} {\mathbf{#1}} } \)
\( \newcommand{\vecd}[1]{\overset{-\!-\!\rightharpoonup}{\vphantom{a}\smash {#1}}} \)
\(\newcommand{\avec}{\mathbf a}\) \(\newcommand{\bvec}{\mathbf b}\) \(\newcommand{\cvec}{\mathbf c}\) \(\newcommand{\dvec}{\mathbf d}\) \(\newcommand{\dtil}{\widetilde{\mathbf d}}\) \(\newcommand{\evec}{\mathbf e}\) \(\newcommand{\fvec}{\mathbf f}\) \(\newcommand{\nvec}{\mathbf n}\) \(\newcommand{\pvec}{\mathbf p}\) \(\newcommand{\qvec}{\mathbf q}\) \(\newcommand{\svec}{\mathbf s}\) \(\newcommand{\tvec}{\mathbf t}\) \(\newcommand{\uvec}{\mathbf u}\) \(\newcommand{\vvec}{\mathbf v}\) \(\newcommand{\wvec}{\mathbf w}\) \(\newcommand{\xvec}{\mathbf x}\) \(\newcommand{\yvec}{\mathbf y}\) \(\newcommand{\zvec}{\mathbf z}\) \(\newcommand{\rvec}{\mathbf r}\) \(\newcommand{\mvec}{\mathbf m}\) \(\newcommand{\zerovec}{\mathbf 0}\) \(\newcommand{\onevec}{\mathbf 1}\) \(\newcommand{\real}{\mathbb R}\) \(\newcommand{\twovec}[2]{\left[\begin{array}{r}#1 \\ #2 \end{array}\right]}\) \(\newcommand{\ctwovec}[2]{\left[\begin{array}{c}#1 \\ #2 \end{array}\right]}\) \(\newcommand{\threevec}[3]{\left[\begin{array}{r}#1 \\ #2 \\ #3 \end{array}\right]}\) \(\newcommand{\cthreevec}[3]{\left[\begin{array}{c}#1 \\ #2 \\ #3 \end{array}\right]}\) \(\newcommand{\fourvec}[4]{\left[\begin{array}{r}#1 \\ #2 \\ #3 \\ #4 \end{array}\right]}\) \(\newcommand{\cfourvec}[4]{\left[\begin{array}{c}#1 \\ #2 \\ #3 \\ #4 \end{array}\right]}\) \(\newcommand{\fivevec}[5]{\left[\begin{array}{r}#1 \\ #2 \\ #3 \\ #4 \\ #5 \\ \end{array}\right]}\) \(\newcommand{\cfivevec}[5]{\left[\begin{array}{c}#1 \\ #2 \\ #3 \\ #4 \\ #5 \\ \end{array}\right]}\) \(\newcommand{\mattwo}[4]{\left[\begin{array}{rr}#1 \amp #2 \\ #3 \amp #4 \\ \end{array}\right]}\) \(\newcommand{\laspan}[1]{\text{Span}\{#1\}}\) \(\newcommand{\bcal}{\cal B}\) \(\newcommand{\ccal}{\cal C}\) \(\newcommand{\scal}{\cal S}\) \(\newcommand{\wcal}{\cal W}\) \(\newcommand{\ecal}{\cal E}\) \(\newcommand{\coords}[2]{\left\{#1\right\}_{#2}}\) \(\newcommand{\gray}[1]{\color{gray}{#1}}\) \(\newcommand{\lgray}[1]{\color{lightgray}{#1}}\) \(\newcommand{\rank}{\operatorname{rank}}\) \(\newcommand{\row}{\text{Row}}\) \(\newcommand{\col}{\text{Col}}\) \(\renewcommand{\row}{\text{Row}}\) \(\newcommand{\nul}{\text{Nul}}\) \(\newcommand{\var}{\text{Var}}\) \(\newcommand{\corr}{\text{corr}}\) \(\newcommand{\len}[1]{\left|#1\right|}\) \(\newcommand{\bbar}{\overline{\bvec}}\) \(\newcommand{\bhat}{\widehat{\bvec}}\) \(\newcommand{\bperp}{\bvec^\perp}\) \(\newcommand{\xhat}{\widehat{\xvec}}\) \(\newcommand{\vhat}{\widehat{\vvec}}\) \(\newcommand{\uhat}{\widehat{\uvec}}\) \(\newcommand{\what}{\widehat{\wvec}}\) \(\newcommand{\Sighat}{\widehat{\Sigma}}\) \(\newcommand{\lt}{<}\) \(\newcommand{\gt}{>}\) \(\newcommand{\amp}{&}\) \(\definecolor{fillinmathshade}{gray}{0.9}\)Chromatography is an analytical technique that separates components in a mixture. Chromatographic columns are part of the instrumentation that is used in chromatography. Five chromatographic methods that use columns are gas chromatography (GC), liquid chromatography (LC), Ion exchange chromatography (IEC), size exclusion chromatography (SEC), and chiral chromatography. The basic principals of chromatography can be applied to all five methods.
Gas Chromatographic Columns
In gas chromatography the mobile phase is a gas. Gas chromatographic columns are usually between 1 and 100 meters long. Gas liquid chromatography(GLC): The liquid stationary phase is bonded or adsorbed onto the surface of an open tubular (capillary) column, or onto a packed solid support inside the column. Matching the polarities of the analyte and stationary phase is not an exact science. The two should have similar polarities. The thickness of the stationary phase ranges between 0.1 and 8 µm. The thicker the layer the more volatile the analyte can be.
High Performance Liquid Chromatographic Columns
High performance liquid chromatography (HPLC) is a type of liquid chromatography that uses a liquid moblie phase. The same basic principals from gas chromatography are applied to liquid chromatography. There are three basic types of liquid chromatographic columns: liquid-liquid, liquid-solid, and ion-exchange. Liquid-liquid chromatographic columns have the liquid stationary phase bonded or absorbed to the surface of the column, or packed material. liquid-liquid chromatographic columns are not as popular because they have limited stability and they are inconvenient. Partitioning occurs between the two different liquids of the mobile and stationary phases. In liquid-solid chromatographic columns the stationary phase is a solid and the analyte absorbs onto the stationary phase which separates the components of the mixture. In ion-exchange chromatographic columns the stationary phase is an ion-exchange resin and partitioning occurs with ion exchanges that occur between the analyte and stationary phase.
Usually HPLC has a guard column ahead of the analytical column to protect and extend the life of the analytical column. The guard column removes particulate matter, contaminants, and molecules that bind irreversibly to the column. The guard column has a stationary phase similar to the analytical column.
The most common HPLC columns are made from stainless steel, but they can be also made out of thick glass, polymers such as polyetherethelketone, a combination of stainless steel and glass, or a combination of stainless steel and polymers. Typical HPLC analytical columns are between 3 and 25 cm long and have a diameter of 1 to 5 mm. The columns are usually straight unlike GC columns. Particles that pack the columns have a typical diameter between 3 to 5 µm. Liquid chromatographic columns will increase in efficiency when the diameter of the packed particles inside the column decreases.
Packing Material
HPLC columns are usually packed with pellicular, or porous particles. Pellicular particles are made from polymer, or glass beads. Pellicular particles are surrounded by a thin uniform layer of silica, polystyrene-divinyl-benzene synthetic resin, alumina, or other type of ion-exchange resin. The diameter of the pellicular beads is between 30 and 40 µm. Porous particles are more commonly used and have diameters between 3 to 10 µm. Porous particles are made up silica, polystyrene-divinyl-benzene synthetic resin, alumina, or other type of ion-exchange resin. Silica is the most common type of porous particle packing material.
Partition HPLC uses liquid bonded phase columns, where the liquid stationary phase is chemically bonded to the packing material. The packing material is usually hydrolyzed silica which reacts with the bond-phase coating. Common bond phase coatings are siloxanes. The relative structure of the siloxane is shown in Figure \(\PageIndex{1}\).
R group attached to siloxane | Chromatography method application |
---|---|
Alkyl | Reverse phase |
Fluoroalkyl | Reverse phase |
Cyano | Normal and reverse phase |
Amide | Reverse phase |
Amino | Normal and reverse phase |
dimethylamine | Weak anion exchanger |
Quaternary Amine | Strong anion exchanger |
Sulfonic Acid | Strong cation exchanger |
Carboxylic Acid | Weak cation exchanger |
Diol | reverse phase |
Phenyl | Reverse phase |
Carbamate | Reverse Phase |
Reverse and Normal Phase HPLC
A polar stationary phase and a non-polar mobile phase are used for normal phase HPLC. In normal phase, the most common R groups attached to the siloxane are: diol, amino, cyano, inorganic oxides, and dimethylamino. Normal phase is also a form of liquid-solid chromatography. The most non-polar compounds will elute first when doing normal phase HPLC.

Reverse phase HPLC uses a polar mobile phase and a non-polar stationary phase. Reverse phase HPLC is the most common liquid chromatography method used. The R groups usually attached to the siloxane for reverse phase HPLC are: C8, C18,or any hydrocarbon. Reverse phase can also use water as the mobile phase, which is advantageous because water is cheap, nontoxic, and invisible in the UV region. The most polar compounds will elute first when performing reverse phase HPLC. Check the animation on the principle of reversed-phase chromatography to understand its principle.
Ion Exchange Chromatographic Columns
Ion exchange columns are used to separate ions and molecules that can be easily ionized. Separation of the ions depends on the ion's affinity for the stationary phase, which creates an ion exchange system. The electrostatic interactions between the analytes, moble phase, and the stationary phase, contribute to the separation of ions in the sample. Only positively or negatively charged complexes can interact with their respective cation or anion exchangers. Common packing materials for ion exchange columns are amines, sulfonic acid, diatomaceous earth, styrene-divinylbenzene, and cross-linked polystyrene resins. Some of the first ion exchangers used were inorganic and made from aluminosilicates (zeolites). Although aluminosilicates are not widely used as ion exchange resins used.
Size Exclusion Chromatographic Columns
Size Exclusion Chromatographic columns separate molecules based upon their size, not molecular weight. A common packing material for these columns is molecular sieves. Zeolites are a common molecular sieve that is used. The molecular sieves have pores that small molecules can go into, but large molecules cannot. This allows the larger molecules to pass through the column faster than the smaller ones. Other packing materials for size exclusion chromatographic columns are polysaccharides and other polymers, and silica. The pore size for size exclusion separations varies between 4 and 200 nm.
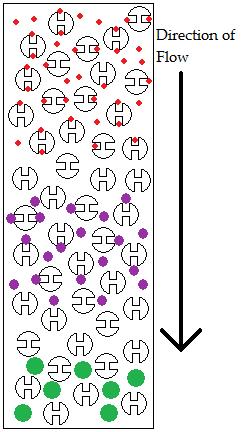
Chiral Columns
Chiral columns are used to separate enantiomers. Separation of chiral molecules is based upon steriochemistry. These columns have a stationary phase that selectively interacts with one enantiomer over the other. These types of columns are very useful for separating racemic mixtures. Some Stationary Phases Used to Separate Enantiomer are show in Table \(\PageIndex{2}\).
Stationary Phase | Method(s) Used |
---|---|
Metal Chelates | GC, LC |
Amino Acid Derivatives | GC, LC |
Proteins | LC |
Helical Polymers | LC |
Cyclodextrin Derivatives | GC, LC |
Column Efficiency
Peak or band broadening causes the column to be less efficient. The ideal situation would to have sharp peaks that are resolved. The longer a substance stays in the column it will cause the peaks to widen. Lengthening the column is a way to improve the separation of different species in the column. A column usually needs to remain at a constant temperature to remain efficient. Plate height and number of theoretical plates determines the efficiency of the column. Improving the efficiency would be to increase the number of plates and decrease the plate height.
The number of plates can be determined from the equation:
\[N=L/H \nonumber \]
where L is the length of the column and H is the height of each plate. N can also be determined from the equation:
\[N=16\left( \dfrac{t_R}{W}\right)^2 \nonumber \]
or
\[N=5.54\left(\dfrac{t_R}{W_{1/2}}\right)^2 \nonumber \]
where \(t_R\) is the retention time, \(W\) is the width of the peak and \(W_{1/2}\) is half the width of the peak.
Height equivalent to a theoretical plate (HETP) is determined from the equation:
\[H=L/N \nonumber \]
or HETP can also be determined by the van Beemter equation:
\[H=A+\dfrac{B}{u}+Cu \nonumber \]
where H equals HETP, A is the term for eddy diffusion, B is the term for longitudinal diffusion, C is the coefficient for mass-transfer between the stationary and mobile phases, and u is the linear velocity. The equation for HETP is often used to describe the efficiency of the column. An efficient column would have a minimum HETP value. Gas chromatographic columns have plate heights that are at least one order of magnitude greater than liquid chromatographic column plates. However GC columns are longer, which causes them to be more efficient. LC columns have a maximum length of 25 cm whereas GC columns can be 100 meters long.
References
- Skoog, D., Holler, J., Crouch, S. Principles of Instrumental Analysis, 6th Ed.; Thomson Brooks/Cole: Belmont, 2007.
- Poole, C.F. The Essence of Chromatography; Elsevier: San Francisco, 2003.
- Miller, J.J. Chromatography: Concepts and Contrasts, 2nd Ed.; John Wiley & Sons, Inc.: Hoboken, NJ, 2005.
- Ravindranath, B., Principles and Practice of Chromatography; John Wiley & Sons: New York, 1989.
- Johnson, E.L., Stevenson, R., Basic Liquid Chromatography; Varian Associates: Palo Alto, CA, 1978.
- Brown, P.R., Hartwick, R.A., High Performance Liquid Chromatography. In Chemical Analysis; Winefordner, J.D., Ed. John Wiley & Sons: New York, 1989; Vol. 98; p 277-295