Learning Objective
Draw, interpret, and convert between Lewis (Kekule), Condensed, and Bond-line Structures
Note: The review of general chemistry in sections 1.3 - 1.6 is integrated into the above Learning Objective for organic chemistry in sections 1.7 and 1.8.
Shorthand notations to represent organic molecules rely on our knowledge of common neutral bonding patterns. Knowing these patterns, we can fill in the missing structural information. Some of these shorthand ways of drawing molecules give us insight into the bond angles and relative positions of atoms in the molecule, while some notations eliminate the carbon and hydrogen atoms and only indicate the heteroatoms (the atoms that are NOT carbon or hydrogen).
There are three primary methods to communicate chemical structure of organic molecules:
Kekule: Lewis structures using lines to represent covalent bonds and showing all atoms and lone pair electrons
Bond-line (Skeletl-line): shows bonds between carbon atoms and heteroatoms) (with lone pair electrons when requested)
Condensed: all atoms are written to communicate structure without drawing any chemical bonds based on the carbon backbone
Introduction
Observe the following drawings of the structure of Retinol, the most common form of vitamin A. The first drawing follows the straight-line (a.k.a. Kekulé) structure which is helpful when you want to look at every single atom; however, showing all of the hydrogen atoms makes it difficult to compare the overall structure with other similar molecules and makes it difficult to focus in on the double bonds and OH group.
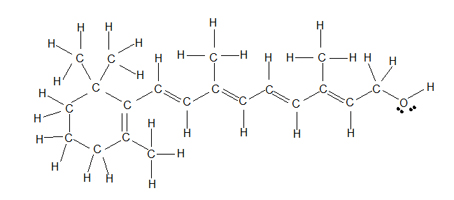
Retinol: Kekulé straight-line drawing
The following is a bond-line (a.k.a. zig-zag) formula for retinol. With this simiplified representation, one can easily see the carbon-carbon bonds, double bonds, OH group, and CH3 groups sticking off of the the main ring and chain. Also, it is much quicker to draw this than the one above. You will learn to appreciate this type of formula writing after drawing a countless number of organic molecules.
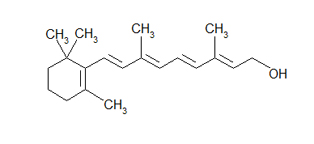
Retinol: Bond-line or zig-zag formula
Importance of Structure
Learning and practicing the basics of Organic Chemistry will help you immensely in the long run as you learn new concepts and reactions. Some people say that Organic Chemistry is like another language, and in some aspects, it is. At first it may seem difficult or overwhelming, but the more you practice looking at and drawing organic molecules, the more familiar you will become with the structures and formulas. Another good idea is to get a model kit and physically make the molecules that you have trouble picturing in your head.
Through general chemistry, you may have already experienced looking at molecular structure. The different ways to draw organic molecules include Kekulé (straight-line), Condensed Formulas, and Bond-Line Formulas (zig-zag). It will be more helpful if you become comfortable going from one style of drawing to another, and look at drawings and understanding what they mean, than knowing which kind of drawing is named what.
An example of a drawing that incorporates all three ways to draw organic molecules would be the following additional drawing of Retinol. The majority of the drawing is Bond-line (zig-zag) formula, but the -CH3 are written as condensed formulas, and the -OH group is written in Kekulé form.
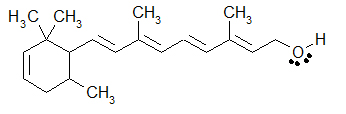
A widely used way of showing the 3D structure of molecules is the use of dashes, wedges, and straight lines. This drawing method is essential because the placement of different atoms could yield different molecules even if the molecular formulas were exactly the same. Below are two drawings of a 4-carbon molecule with two chlorines and two bromines attached.
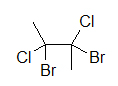
4-carbon molecule with 2 chlorines and 2 bromines 4-carbon molecule with 2 chlorines and 2 bromines
Both drawings look like they represent the same molecule; however, if we add dashes and wedged we will see that two different molecules could be depicted:
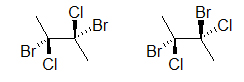
The two molecules above are different, prove this to yourself by building a model. An easier way to compare the two molecules is to rotate one of the bonds (here, it is the bond on the right):
.jpg?revision=1)
Notice how the molecule on the right has both bromines on the same side and chlorines on the same side, whereas the first molecule is different. Read about Dashed-Wedged Line structures, bottom of page, to understand what has been introduced above. You will learn more about the importance of atomic connectivity in molecules as you continue on to learn about Stereochemistry.
Drawing the Structure of Organic Molecules
Although larger molecules may look complicated, they can be easily understood by breaking them down and looking at their smaller components.
All atoms want to have their valence shell full, a "closed shell." Hydrogen wants to have 2 e- whereas carbon, oxygen, and nitrogen want to have 8 e-. When looking at the different representations of molecules, keep in mind the Octet Rule. Also remember that hydrogen can bond one time, oxygen can bond up to two times, nitrogen can bond up to three times, and carbon can bond up to four times.
.jpg?revision=1)
Kekulé (a.k.a. Lewis Structures)
Kekulé structures are similar to Lewis Structures, but instead of covalent bonds being represented by electron dots, the two shared electrons are shown by a line.
(A)
(B)
(C)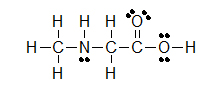
Lone pairs remain as two electron dots, or are sometimes left out even though they are still there. Notice how the three lone pairs of electrons were not draw in around chlorine in example B.
A condensed formula is made up of the elemental symbols. The order of the atoms suggests the connectivity. Condensed formulas can be read from either direction and H3C is the same as CH3, although the latter is more common because Look at the examples below and match them with their identical molecule under Kekulé structures and bond-line formulas.
(A) CH3CH2OH (B) ClCH2CH2CH(OCH3)CH3 (C) H3CNHCH2COOH
Let's look closely at example B. As you go through a condensed formula, you want to focus on the carbons and other elements that aren't hydrogen. The hydrogen's are important, but are usually there to complete octets. Also, notice the -OCH3 is in written in parentheses which tell you that it not part of the main chain of carbons. As you read through a a condensed formula, if you reach an atom that doesn't have a complete octet by the time you reach the next hydrogen, then it's possible that there are double or triple bonds. In example C, the carbon is double bonded to oxygen and single bonded to another oxygen. Notice how COOH means C(=O)-O-H instead of CH3-C-O-O-H because carbon does not have a complete octet and oxygens.
The name gives away how this formula works. This formula is full of bonds and lines, and because of the typical (more stable) bonds that atoms tend to make in molecules, they often end up looking like zig-zag lines. If you work with a molecular model kit you will find it difficult to make stick straight molecules (unless they contain sp triple bonds) whereas zig-zag molecules and bonds are much more feasible.
(A)
(B)
(C)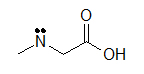
These molecules correspond to the exact same molecules depicted for Kekulé structures and condensed formulas. Notice how the carbons are no longer drawn in and are replaced by the ends and bends of a lines. In addition, the hydrogens have been omitted, but could be easily drawn in (see practice problems). Although we do not usually draw in the H's that are bonded to carbon, we do draw them in if they are connected to other atoms besides carbon (example is the OH group above in example A) . This is done because it is not always clear if the non-carbon atom is surrounded by lone pairs or hydrogens. Also in example A, notice how the OH is drawn with a bond to the second carbon, but it does not mean that there is a third carbon at the end of that bond/ line.
Dashed-Wedged Line Structure
As you may have guessed, the Dashed-Wedged Line structure is all about lines, dashes, and wedges. At first it may seem confusing, but with practice, understanding dash-wedged line structures will become like second nature. The following are examples of each, and how they can be used together.

Above are 4-carbon chains with attached OH groups or Cl and Br atoms. Remember that each line represents a bond and that the carbons and hydrogens have been omitted. When you look at or draw these structures, the straight lines illustrate atoms and bonds that are in the same plane, the plane of the paper (in this case, computer screen). Dashed lines show atoms and bonds that go into the page, behind the plane, away from you. In the above example, the OH group is going into the plane, while at the same time a hydrogen comes out (wedged).

Blue bead= OH group; White bead=H
Wedged lines illustrate bonds and atoms that come out of the page, in front of the plane, toward you. In the 2D diagram above, the OH group is coming out of the plane of the paper, while a hydrogen goes in (dashed).

Blue bead= OH group; White bead=H
As stated before, straight lines illustrate atoms and bonds that are in the same plane as the paper, but in the 2D example, the straight line bond for OH means that it it unsure or irrelevant whether OH is going away or toward you. It is also assumed that hydrogen is also connected to the same carbon that OH is on.
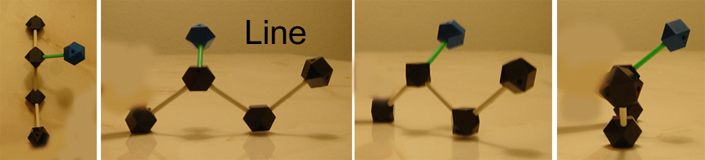
Blue bead= OH group; H is not shown
Try using your model kit to see that the OH group cannot lie in the same plane at the carbon chain (don't forget your hydrogens!). In the final 2Dexample, both dashed and wedged lines are used because the attached atoms are not hydrogens (although dashed and wedged lines can be used for hydrogens).The chlorine is coming out the page while bromine is going into the page.

Blue bead=Cl; Red bead=Br
Throughout the course, it will be helpful to convert compounds into different structural formulas (Kekule (Lewis Structures), Bond-line, and Condensed) depending on the type of question that is asked. Standardized exams frequently include a high percentage of condensed formulas because it is easier and cheaper to type letters and numbers than to import figures. Initially, it can be tricky writing a bond-line structure directly from a condensed formula. First write the Kekule structure from the condensed formula and then draw the bond-line structure from the Kekule. Practice will quickly allow you to convert directly between condensed and bond-line structures.
The condensed formula for propanal is CH3CH2CHO. Can you visualize the bond-line structure of propanal? If yes, excellent, If no, the following practice will help.
The Kekule structure for propanal is shown below.
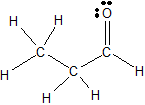
The bond-line structure for propanal is shown below.

All three structures represent the same compound, propanal.

Exercises
- How many carbons are in the following drawing? How many hydrogens?
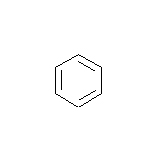
- How many carbons are in the following drawing? How many hydrogens?

- How many carbons are in the following drawing? How many hydrogens?
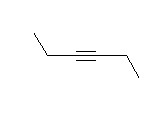
- Look at the following molecule of vitamin A and draw in the hidden hydrogens and electron pairs.
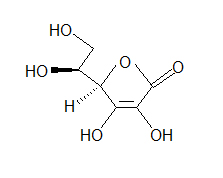
(hint: Do all of the carbons have 4 bonds? Do all the oxygens have a full octet?)
- How many bonds can hydrogen make?
- How many bonds can chlorine make?
- Dashed lines means the atomic bond goes ___________(away/toward) you.
- Draw ClCH2CH2CH(OCH3)CH3 in Kekuléand zig-zag form.
- Extra practice problems can be found ______?
Solutions
- Remember the octet rule and how many times carbons and hydrogens are able to bond to other atoms.
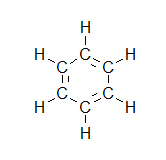
-
.jpg?revision=1)
-
.jpg?revision=1)
- Electron pairs drawn in blue and hydrogens draw in red.
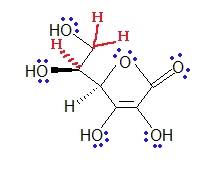
- Hygrogen can make one bond.
- Chlorine can make one bond.
- Away
- See (B) under Kekulé and Bond-line (zig-zag) formulas.
- Extra practice problems can be found: in your textbook, homework, lecture notes, online, reference books, and more. Try making up some of your own molecules, they may exist!
References
- Vollhardt, K. Peter C., and Neil E. Schore. Organic Chemistry: Structure and Function. 5th ed. New York: W. H. Freeman Company, 2007. 38-40.
- Klein, David R. Organic Chemistry I As a Second Language. 2nd ed. Hoboken, NJ: John Wiley & Sons, Inc, 2007. 1-14.
Contributors and Attributions
- Choo, Ezen (2009, UCD '11)
The building block of structural organic chemistry is the tetravalent carbon atom. With few exceptions, carbon compounds can be formulated with four covalent bonds to each carbon, regardless of whether the combination is with carbon or some other element. The two-electron bond, which is illustrated by the carbon-hydrogen bonds in methane or ethane and the carbon-carbon bond in ethane, is called a single bond. In these and many related substances, each carbon is attached to four other atoms:
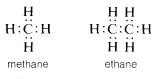
There exist, however, compounds such as ethene (ethylene), \(C_2H_4\), in which two electrons from each of the carbon atoms are mutually shared, thereby producing two two-electron bonds, an arrangement which is called a double bond. Each carbon in ethene is attached to only three other atoms:
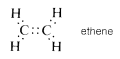
Similarly, in ethyne (acetylene), \(C_2H_2\), three electrons from each carbon atom are mutually shared, producing three two-electron bonds, called a triple bond, in which each carbon is attached to only two other atoms:
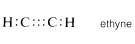
Of course, in all cases each carbon has a full octet of electrons. Carbon also forms double and triple bonds with several other elements that can exhibit a covalence of two or three. The carbon-oxygen (or carbonyl) double bond appears in carbon dioxide and many important organic compounds such as methanal (formaldehyde) and ethanoic acid (acetic acid). Similarly, a carbon-nitrogen triple bond appears in methanenitrile (hydrogen cyanide) and ethanenitrile (acetonitrile).
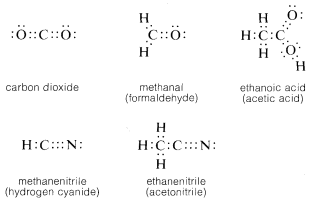
By convention, a single straight line connecting the atomic symbols is used to represent a single (two-electron) bond, two such lines to represent a double (four-electron) bond, and three lines a triple (six-electron) bond. Representations of compounds by these symbols are called structural formulas; some examples are
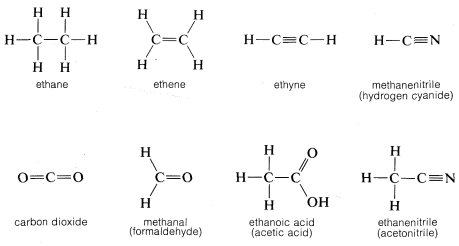
A point worth noting is that structural formulas usually do not indicate the nonbonding electron pairs. This is perhaps unfortunate because they play as much a part in the chemistry of organic molecules as do the bonding electrons and their omission may lead the unwary reader to overlook them. However, when it is important to represent them, this can be done best with pairs of dots, although a few authors use lines:

To save space and time in the representation of organic structures, it is common practice to use "condensed formulas" in which the bonds are not shown explicitly. In using condensed formulas, normal atomic valences are understood throughout. Examples of condensed formulas are
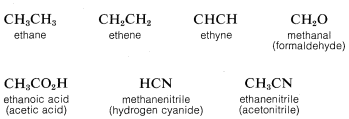
Another type of abbreviation that often is used, particularly for ring compounds, dispenses with the symbols for carbon and hydrogen atoms and leaves only the lines in a structural formula. For instance, cyclopentane, \(C_5H_{10}\), often is represented as a regular pentagon in which it is understood that each apex represents a carbon atom with the requisite number of hydrogens to satisfy the tetravalence of carbon:
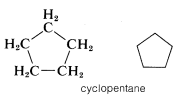
Likewise, cyclopropane, \(C_3H_6\); cyclobutane, \(C_4H_8\); and cyclohexane, \(C_6H_{12}\), are drawn as regular polygons:
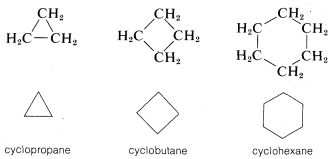
Although this type of line drawing is employed most commonly for cyclic structures, its use for open chain (acyclic) structures is becoming increasingly widespread. There is no special merit to this abbreviation for simple structures such as butane, \(C_4H_{10}\); 1-butene, \(C_4H_8\); or 1,3-butadiene, \(C_4H_6\), but it is of value in representing more complex molecules such as \(\beta\)-carotene, \(C_{40}H_{56}\):
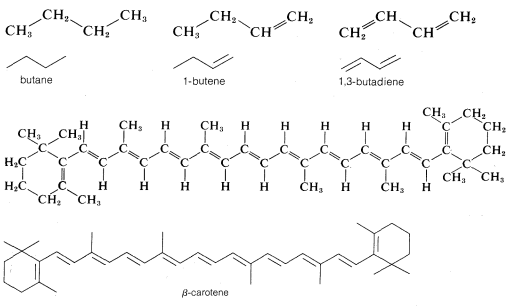
Line structures also can be modified to represent the three-dimensional shapes of molecules, and the way that this is done will be discussed in detail in Chapter 5. At the onset of you study of organic chemistry, you should write out the formulas rather completely until you are thoroughly familiar with what these abbreviations stand for.
Contributors and Attributions
John D. Robert and Marjorie C. Caserio (1977) Basic Principles of Organic Chemistry, second edition. W. A. Benjamin, Inc. , Menlo Park, CA. ISBN 0-8053-8329-8. This content is copyrighted under the following conditions, "You are granted permission for individual, educational, research and non-commercial reproduction, distribution, display and performance of this work in any format."