14.4: Nuclear Decay Processes
- Page ID
- 478413
\( \newcommand{\vecs}[1]{\overset { \scriptstyle \rightharpoonup} {\mathbf{#1}} } \)
\( \newcommand{\vecd}[1]{\overset{-\!-\!\rightharpoonup}{\vphantom{a}\smash {#1}}} \)
\( \newcommand{\id}{\mathrm{id}}\) \( \newcommand{\Span}{\mathrm{span}}\)
( \newcommand{\kernel}{\mathrm{null}\,}\) \( \newcommand{\range}{\mathrm{range}\,}\)
\( \newcommand{\RealPart}{\mathrm{Re}}\) \( \newcommand{\ImaginaryPart}{\mathrm{Im}}\)
\( \newcommand{\Argument}{\mathrm{Arg}}\) \( \newcommand{\norm}[1]{\| #1 \|}\)
\( \newcommand{\inner}[2]{\langle #1, #2 \rangle}\)
\( \newcommand{\Span}{\mathrm{span}}\)
\( \newcommand{\id}{\mathrm{id}}\)
\( \newcommand{\Span}{\mathrm{span}}\)
\( \newcommand{\kernel}{\mathrm{null}\,}\)
\( \newcommand{\range}{\mathrm{range}\,}\)
\( \newcommand{\RealPart}{\mathrm{Re}}\)
\( \newcommand{\ImaginaryPart}{\mathrm{Im}}\)
\( \newcommand{\Argument}{\mathrm{Arg}}\)
\( \newcommand{\norm}[1]{\| #1 \|}\)
\( \newcommand{\inner}[2]{\langle #1, #2 \rangle}\)
\( \newcommand{\Span}{\mathrm{span}}\) \( \newcommand{\AA}{\unicode[.8,0]{x212B}}\)
\( \newcommand{\vectorA}[1]{\vec{#1}} % arrow\)
\( \newcommand{\vectorAt}[1]{\vec{\text{#1}}} % arrow\)
\( \newcommand{\vectorB}[1]{\overset { \scriptstyle \rightharpoonup} {\mathbf{#1}} } \)
\( \newcommand{\vectorC}[1]{\textbf{#1}} \)
\( \newcommand{\vectorD}[1]{\overrightarrow{#1}} \)
\( \newcommand{\vectorDt}[1]{\overrightarrow{\text{#1}}} \)
\( \newcommand{\vectE}[1]{\overset{-\!-\!\rightharpoonup}{\vphantom{a}\smash{\mathbf {#1}}}} \)
\( \newcommand{\vecs}[1]{\overset { \scriptstyle \rightharpoonup} {\mathbf{#1}} } \)
\( \newcommand{\vecd}[1]{\overset{-\!-\!\rightharpoonup}{\vphantom{a}\smash {#1}}} \)
\(\newcommand{\avec}{\mathbf a}\) \(\newcommand{\bvec}{\mathbf b}\) \(\newcommand{\cvec}{\mathbf c}\) \(\newcommand{\dvec}{\mathbf d}\) \(\newcommand{\dtil}{\widetilde{\mathbf d}}\) \(\newcommand{\evec}{\mathbf e}\) \(\newcommand{\fvec}{\mathbf f}\) \(\newcommand{\nvec}{\mathbf n}\) \(\newcommand{\pvec}{\mathbf p}\) \(\newcommand{\qvec}{\mathbf q}\) \(\newcommand{\svec}{\mathbf s}\) \(\newcommand{\tvec}{\mathbf t}\) \(\newcommand{\uvec}{\mathbf u}\) \(\newcommand{\vvec}{\mathbf v}\) \(\newcommand{\wvec}{\mathbf w}\) \(\newcommand{\xvec}{\mathbf x}\) \(\newcommand{\yvec}{\mathbf y}\) \(\newcommand{\zvec}{\mathbf z}\) \(\newcommand{\rvec}{\mathbf r}\) \(\newcommand{\mvec}{\mathbf m}\) \(\newcommand{\zerovec}{\mathbf 0}\) \(\newcommand{\onevec}{\mathbf 1}\) \(\newcommand{\real}{\mathbb R}\) \(\newcommand{\twovec}[2]{\left[\begin{array}{r}#1 \\ #2 \end{array}\right]}\) \(\newcommand{\ctwovec}[2]{\left[\begin{array}{c}#1 \\ #2 \end{array}\right]}\) \(\newcommand{\threevec}[3]{\left[\begin{array}{r}#1 \\ #2 \\ #3 \end{array}\right]}\) \(\newcommand{\cthreevec}[3]{\left[\begin{array}{c}#1 \\ #2 \\ #3 \end{array}\right]}\) \(\newcommand{\fourvec}[4]{\left[\begin{array}{r}#1 \\ #2 \\ #3 \\ #4 \end{array}\right]}\) \(\newcommand{\cfourvec}[4]{\left[\begin{array}{c}#1 \\ #2 \\ #3 \\ #4 \end{array}\right]}\) \(\newcommand{\fivevec}[5]{\left[\begin{array}{r}#1 \\ #2 \\ #3 \\ #4 \\ #5 \\ \end{array}\right]}\) \(\newcommand{\cfivevec}[5]{\left[\begin{array}{c}#1 \\ #2 \\ #3 \\ #4 \\ #5 \\ \end{array}\right]}\) \(\newcommand{\mattwo}[4]{\left[\begin{array}{rr}#1 \amp #2 \\ #3 \amp #4 \\ \end{array}\right]}\) \(\newcommand{\laspan}[1]{\text{Span}\{#1\}}\) \(\newcommand{\bcal}{\cal B}\) \(\newcommand{\ccal}{\cal C}\) \(\newcommand{\scal}{\cal S}\) \(\newcommand{\wcal}{\cal W}\) \(\newcommand{\ecal}{\cal E}\) \(\newcommand{\coords}[2]{\left\{#1\right\}_{#2}}\) \(\newcommand{\gray}[1]{\color{gray}{#1}}\) \(\newcommand{\lgray}[1]{\color{lightgray}{#1}}\) \(\newcommand{\rank}{\operatorname{rank}}\) \(\newcommand{\row}{\text{Row}}\) \(\newcommand{\col}{\text{Col}}\) \(\renewcommand{\row}{\text{Row}}\) \(\newcommand{\nul}{\text{Nul}}\) \(\newcommand{\var}{\text{Var}}\) \(\newcommand{\corr}{\text{corr}}\) \(\newcommand{\len}[1]{\left|#1\right|}\) \(\newcommand{\bbar}{\overline{\bvec}}\) \(\newcommand{\bhat}{\widehat{\bvec}}\) \(\newcommand{\bperp}{\bvec^\perp}\) \(\newcommand{\xhat}{\widehat{\xvec}}\) \(\newcommand{\vhat}{\widehat{\vvec}}\) \(\newcommand{\uhat}{\widehat{\uvec}}\) \(\newcommand{\what}{\widehat{\wvec}}\) \(\newcommand{\Sighat}{\widehat{\Sigma}}\) \(\newcommand{\lt}{<}\) \(\newcommand{\gt}{>}\) \(\newcommand{\amp}{&}\) \(\definecolor{fillinmathshade}{gray}{0.9}\)- Describe the composition and properties of the different types of radiation.
Radioactive decay involves the emission of a particle and/or energy as one atom changes into another. In most instances, the atom changes its identity to become a new element. There are four different types of emissions that occur. We will explore each of them in this section, as well as some of their properties.
Alpha Emission
Alpha \(\left( \alpha \right)\) decay involves the release of helium ions from the nucleus of an atom. This ion consists of two protons and two neutrons and has a \(2+\) charge. Release of an \(\alpha\)-particle produces a new atom that has an atomic number two less than the original atom and an atomic weight that is four less. A typical alpha decay reaction is the conversion of uranium-238 to thorium:
\[\ce{^{238}_{92}U} \rightarrow \ce{^{234}_{90}Th} + \ce{^4_2 \alpha}\nonumber \]
We see a decrease of two in the atomic number (uranium to thorium) and a decrease of four in the atomic weight (238 to 234). Usually the emission is not written with atomic number and weight indicated since it is a common particle whose properties should be memorized. Quite often the alpha emission is accompanied by gamma \(\left( \gamma \right)\) radiation, a form of energy release. Many of the largest elements in the periodic table are alpha-emitters.
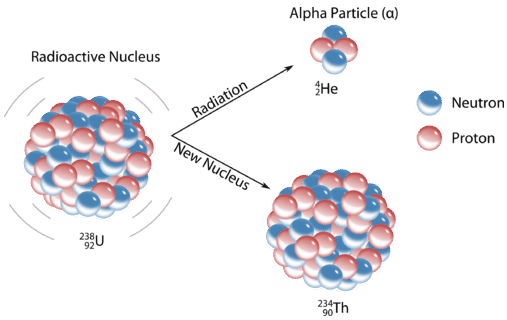
Beta Emission
Beta \(\left( \beta \right)\) decay is a more complicated process. Unlike the \(\alpha\)-emission, which simply expels a particle, the \(\beta\)-emission involves the transformation of a neutron in the nucleus to a proton and an electron. The electron is then ejected from the nucleus. In the process, the atomic number increases by one while the atomic weight stays the same. As is the case with \(\alpha\)-emissions, \(\beta\)-emissions are often accompanied by \(\gamma\)-radiation.
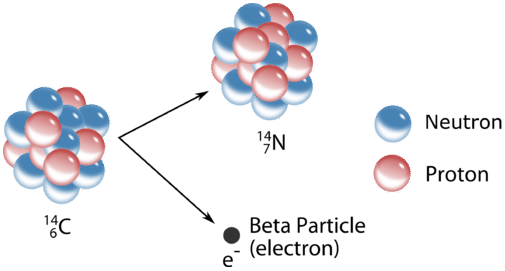
A typical beta decay process involves carbon-14, often used in radioactive dating techniques. The reaction forms nitrogen-14 and an electron:
\[\ce{^{14}_6C} \rightarrow \ce{^{14}_7N} + \ce{^0_{-1}e}\nonumber \]
Again, the beta emission is usually simply indicated by the Greek letter \(\beta\); memorization of the process is necessary in order to follow nuclear calculations in which the Greek letter \(\beta\) appears without further notation.
Positron Emission
A positron is a positive electron (a form of antimatter). This rare type of emission occurs when a proton is converted to a neutron and a positron in the nucleus, with ejection of the positron. The atomic number will decrease by one, while the atomic weight does not change. A positron is often designated by \(\beta^+\).
Carbon-11 emits a positron to become boron-11:
\[\ce{^{11}_6C} \rightarrow \ce{^{11}_5B} + \ce{^0_{+1} \beta}\nonumber \]
Electron Capture
An alternate way for a nuclide to increase its neutron to proton ratio is by a phenomenon called electron capture. In electron capture, an electron from an inner orbital is captured by the nucleus of the atom, and combined with a proton to form a neutron. For example, silver-106 undergoes electron capture to become palladium-106.
\[\ce{^{106}_{47}Ag} + \ce{^0_{-1}e} \rightarrow \ce{^{106}_{46}Pd}\nonumber \]
Note that the overall result of electron capture is identical to positron emission. The atomic number decreases by one while the mass number remains the same.
Gamma Emission
Gamma \(\left( \gamma \right)\) radiation is high energy electromagnetic radiation, as introduced earlier in this text. It may be released by itself or, more commonly, in association with other radiation events. There is no change of atomic number or atomic weight in a simple \(\gamma\)-emission. Often, an isotope may produce \(\gamma\)-radiation as a result of a transition in a metastable isotope. This type of isotope may just "settle", with a shifting of particles in the nucleus. The composition of the atom is not altered, but the nucleus could be considered more "comfortable" after the shift. This shift increases the stability of the isotope from the energetically unstable (or "metastable") isotope to a more stable form of the nucleus.
Penetrating Ability of Emissions
The various emissions differ considerably in their ability to go through matter, known as their penetrating ability. The \(\alpha\)-particle has the least penetrating power since it is the largest and slowest emission. It can be blocked by a sheet of paper or a human hand. Beta particles are more penetrating than alpha particles, but can be stopped by a thin sheet of aluminum. Of the three basic types of emissions, gamma particles are the most penetrating. A thick lead shield is required to stop gamma emissions. Positrons represent a special case in that they annihilate when they come in contact with electrons. The collision of a positron and an electron results in the formation of two gamma emissions that go 180 degrees away from each other.
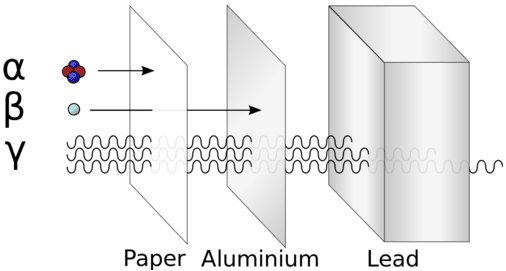
Blocking of alpha particles can easily be accomplished by as little as \(10 \: \text{mm}\) plastic or paper. Beta emissions represent a somewhat different situation. The negative charge on a beta particle has the potential for activating the element being used to block the radiation. Lead and tungsten are large atoms with many protons and neutrons in their nuclei. While the beta electron may be blocked, the target material could become irradiated in the process.
High-density materials are much more effective protection against gamma emissions than low-density ones. Gamma rays are usually blocked effectively by lead shielding. The thickness of the shielding will determine the effectiveness of the protection offered by the lead.
Section Summary
- Radioactive decay involves the emission of a particle and/or energy as one atom changes into another.
- Alpha \(\left( \alpha \right)\) decay involves the release of helium ions from the nucleus of an atom.
- Beta \(\left( \beta \right)\) decay involves the transformation of a neutron in the nucleus to a proton and an electron.
- A positron is a positive electron (a form of antimatter). This rare type of emission occurs when a proton is converted to a neutron and a positron in the nucleus, with ejection of the positron.
- Gamma \(\left( \gamma \right)\) radiation is simply energy. It may be released by itself or, more commonly, in association with other radiation events.
- The penetrating ability of a radioactive emission is its ability to go through matter.
- The relative penetrating abilities of radioactive emissions are described.
Glossary
- Alpha \(\left( \alpha \right)\) decay
- The release of helium ions from the nucleus of an atom.
- Beta \(\left( \beta \right)\) decay
- The release of an electron from the nucleus, as a neutron transforms into a proton.
- positron emission
- The release of a positive electron from the nucleus, as a proton transforms into a neutron.
- Gamma \(\left( \gamma \right)\)
- High energy electromagnetic radiation.
- Penetrating ability
- Ability of nuclear radiation to go through matter.
- Electron capture (EC)
- An electron from an inner orbital is captured by the nucleus of the atom, and combined with a proton to form a neutron.