Chapter 20.2: The Chemistry of Hydrogen
- Page ID
- 28605
\( \newcommand{\vecs}[1]{\overset { \scriptstyle \rightharpoonup} {\mathbf{#1}} } \)
\( \newcommand{\vecd}[1]{\overset{-\!-\!\rightharpoonup}{\vphantom{a}\smash {#1}}} \)
\( \newcommand{\id}{\mathrm{id}}\) \( \newcommand{\Span}{\mathrm{span}}\)
( \newcommand{\kernel}{\mathrm{null}\,}\) \( \newcommand{\range}{\mathrm{range}\,}\)
\( \newcommand{\RealPart}{\mathrm{Re}}\) \( \newcommand{\ImaginaryPart}{\mathrm{Im}}\)
\( \newcommand{\Argument}{\mathrm{Arg}}\) \( \newcommand{\norm}[1]{\| #1 \|}\)
\( \newcommand{\inner}[2]{\langle #1, #2 \rangle}\)
\( \newcommand{\Span}{\mathrm{span}}\)
\( \newcommand{\id}{\mathrm{id}}\)
\( \newcommand{\Span}{\mathrm{span}}\)
\( \newcommand{\kernel}{\mathrm{null}\,}\)
\( \newcommand{\range}{\mathrm{range}\,}\)
\( \newcommand{\RealPart}{\mathrm{Re}}\)
\( \newcommand{\ImaginaryPart}{\mathrm{Im}}\)
\( \newcommand{\Argument}{\mathrm{Arg}}\)
\( \newcommand{\norm}[1]{\| #1 \|}\)
\( \newcommand{\inner}[2]{\langle #1, #2 \rangle}\)
\( \newcommand{\Span}{\mathrm{span}}\) \( \newcommand{\AA}{\unicode[.8,0]{x212B}}\)
\( \newcommand{\vectorA}[1]{\vec{#1}} % arrow\)
\( \newcommand{\vectorAt}[1]{\vec{\text{#1}}} % arrow\)
\( \newcommand{\vectorB}[1]{\overset { \scriptstyle \rightharpoonup} {\mathbf{#1}} } \)
\( \newcommand{\vectorC}[1]{\textbf{#1}} \)
\( \newcommand{\vectorD}[1]{\overrightarrow{#1}} \)
\( \newcommand{\vectorDt}[1]{\overrightarrow{\text{#1}}} \)
\( \newcommand{\vectE}[1]{\overset{-\!-\!\rightharpoonup}{\vphantom{a}\smash{\mathbf {#1}}}} \)
\( \newcommand{\vecs}[1]{\overset { \scriptstyle \rightharpoonup} {\mathbf{#1}} } \)
\( \newcommand{\vecd}[1]{\overset{-\!-\!\rightharpoonup}{\vphantom{a}\smash {#1}}} \)
\(\newcommand{\avec}{\mathbf a}\) \(\newcommand{\bvec}{\mathbf b}\) \(\newcommand{\cvec}{\mathbf c}\) \(\newcommand{\dvec}{\mathbf d}\) \(\newcommand{\dtil}{\widetilde{\mathbf d}}\) \(\newcommand{\evec}{\mathbf e}\) \(\newcommand{\fvec}{\mathbf f}\) \(\newcommand{\nvec}{\mathbf n}\) \(\newcommand{\pvec}{\mathbf p}\) \(\newcommand{\qvec}{\mathbf q}\) \(\newcommand{\svec}{\mathbf s}\) \(\newcommand{\tvec}{\mathbf t}\) \(\newcommand{\uvec}{\mathbf u}\) \(\newcommand{\vvec}{\mathbf v}\) \(\newcommand{\wvec}{\mathbf w}\) \(\newcommand{\xvec}{\mathbf x}\) \(\newcommand{\yvec}{\mathbf y}\) \(\newcommand{\zvec}{\mathbf z}\) \(\newcommand{\rvec}{\mathbf r}\) \(\newcommand{\mvec}{\mathbf m}\) \(\newcommand{\zerovec}{\mathbf 0}\) \(\newcommand{\onevec}{\mathbf 1}\) \(\newcommand{\real}{\mathbb R}\) \(\newcommand{\twovec}[2]{\left[\begin{array}{r}#1 \\ #2 \end{array}\right]}\) \(\newcommand{\ctwovec}[2]{\left[\begin{array}{c}#1 \\ #2 \end{array}\right]}\) \(\newcommand{\threevec}[3]{\left[\begin{array}{r}#1 \\ #2 \\ #3 \end{array}\right]}\) \(\newcommand{\cthreevec}[3]{\left[\begin{array}{c}#1 \\ #2 \\ #3 \end{array}\right]}\) \(\newcommand{\fourvec}[4]{\left[\begin{array}{r}#1 \\ #2 \\ #3 \\ #4 \end{array}\right]}\) \(\newcommand{\cfourvec}[4]{\left[\begin{array}{c}#1 \\ #2 \\ #3 \\ #4 \end{array}\right]}\) \(\newcommand{\fivevec}[5]{\left[\begin{array}{r}#1 \\ #2 \\ #3 \\ #4 \\ #5 \\ \end{array}\right]}\) \(\newcommand{\cfivevec}[5]{\left[\begin{array}{c}#1 \\ #2 \\ #3 \\ #4 \\ #5 \\ \end{array}\right]}\) \(\newcommand{\mattwo}[4]{\left[\begin{array}{rr}#1 \amp #2 \\ #3 \amp #4 \\ \end{array}\right]}\) \(\newcommand{\laspan}[1]{\text{Span}\{#1\}}\) \(\newcommand{\bcal}{\cal B}\) \(\newcommand{\ccal}{\cal C}\) \(\newcommand{\scal}{\cal S}\) \(\newcommand{\wcal}{\cal W}\) \(\newcommand{\ecal}{\cal E}\) \(\newcommand{\coords}[2]{\left\{#1\right\}_{#2}}\) \(\newcommand{\gray}[1]{\color{gray}{#1}}\) \(\newcommand{\lgray}[1]{\color{lightgray}{#1}}\) \(\newcommand{\rank}{\operatorname{rank}}\) \(\newcommand{\row}{\text{Row}}\) \(\newcommand{\col}{\text{Col}}\) \(\renewcommand{\row}{\text{Row}}\) \(\newcommand{\nul}{\text{Nul}}\) \(\newcommand{\var}{\text{Var}}\) \(\newcommand{\corr}{\text{corr}}\) \(\newcommand{\len}[1]{\left|#1\right|}\) \(\newcommand{\bbar}{\overline{\bvec}}\) \(\newcommand{\bhat}{\widehat{\bvec}}\) \(\newcommand{\bperp}{\bvec^\perp}\) \(\newcommand{\xhat}{\widehat{\xvec}}\) \(\newcommand{\vhat}{\widehat{\vvec}}\) \(\newcommand{\uhat}{\widehat{\uvec}}\) \(\newcommand{\what}{\widehat{\wvec}}\) \(\newcommand{\Sighat}{\widehat{\Sigma}}\) \(\newcommand{\lt}{<}\) \(\newcommand{\gt}{>}\) \(\newcommand{\amp}{&}\) \(\definecolor{fillinmathshade}{gray}{0.9}\)
Learning Objectives
- To describe the physical and chemical properties of hydrogen and predict its reactivity.
We now turn from an overview of periodic trends to a discussion of the s-block elements, first by focusing on hydrogen, whose chemistry is sufficiently distinct and important to be discussed in a category of its own. Most versions of the periodic table place hydrogen in the upper left corner immediately above lithium, implying that hydrogen, with a 1s1 electron configuration, is a member of group 1. In fact, the chemistry of hydrogen does not greatly resemble that of the metals of group 1. Indeed, some versions of the periodic table place hydrogen above fluorine in group 17 because the addition of a single electron to a hydrogen atom completes its valence shell.
Note the Pattern
Although hydrogen has an ns1 electron configuration, its chemistry does not resemble that of the metals of group 1.
Isotopes of Hydrogen
Hydrogen, the most abundant element in the universe, is the ultimate source of all other elements by the process of nuclear fusion. (For more information on nuclear fusion, see Chapter 24). Table 20.2.1 compares the three isotopes of hydrogen, all of which contain one proton and one electron per atom. The most common isotope is protiumThe most common isotope of hydrogen, consisting of one proton and one electron. (1H or H), followed by deuteriumAn isotope of hydrogen that consists of one proton, one neutron, and one electron. (2H or D), which has an additional neutron. The rarest isotope of hydrogen is tritiumA rare isotope of hydrogen that consists of one proton, two neutrons, and one electron. (3H or T), which is produced in the upper atmosphere by a nuclear reaction when cosmic rays strike nitrogen and other atoms; it is then washed into the oceans by rainfall. Tritium is radioactive, decaying to 3He with a half-life of only 12.32 years. Consequently, the atmosphere and oceans contain only a very low, steady-state level of tritium. The term hydrogen and the symbol H normally refer to the naturally occurring mixture of the three isotopes.
Table 20.2.1 The Isotopes of Hydrogen
Protium | Deuterium | Tritium | |
---|---|---|---|
symbol | >\( _{1}^{1}\textrm{H} \) | \( _{1}^{2}\textrm{H} \) | \( _{1}^{3}\textrm{H} \) |
neutrons | 0 | 1 | 2 |
mass (amu) | 1.00783 | 2.0140 | 3.01605 |
abundance (%) | 99.9885 | 0.0115 | ∼10−17 |
half-life (years) | — | — | 12.32 |
boiling point of X2 (K) | 20.28 | 23.67 | 25 |
melting point/boiling point of X2O (°C) | 0.0/100.0 | 3.8/101.4 | 4.5/? |
The different masses of the three isotopes of hydrogen cause them to have different physical properties. Thus H2, D2, and T2 differ in their melting points, boiling points, densities, and heats of fusion and vaporization. In 1931, Harold Urey and coworkers discovered deuterium by slowly evaporating several liters of liquid hydrogen until a volume of about 1 mL remained. When that remaining liquid was vaporized and its emission spectrum examined, they observed new absorption lines in addition to those previously identified as originating from hydrogen. The natural abundance of tritium, in contrast, is so low that it could not be detected by similar experiments; it was first prepared in 1934 by a nuclear reaction.
Harold Urey (1893–1981)
Urey won the Nobel Prize in Chemistry in 1934 for his discovery of deuterium (2H). Urey was born and educated in rural Indiana. After earning a BS in zoology from the University of Montana in 1917, Urey changed career directions. He earned his PhD in chemistry at Berkeley with G. N. Lewis (of Lewis electron structure fame) and subsequently worked with Niels Bohr in Copenhagen. During World War II, Urey was the director of war research for the Atom Bomb Project at Columbia University. In later years, his research focused on the evolution of life. In 1953, he and his graduate student, Stanley Miller, showed that organic compounds, including amino acids, could be formed by passing an electric discharge through a mixture of compounds thought to be present in the atmosphere of primitive Earth.
Because the normal boiling point of D2O is 101.4°C (compared to 100.0°C for H2O), evaporation or fractional distillation can be used to increase the concentration of deuterium in a sample of water by the selective removal of the more volatile H2O. Thus bodies of water that have no outlet, such as the Great Salt Lake and the Dead Sea, which maintain their level solely by evaporation, have significantly higher concentrations of deuterated water than does lake or seawater with at least one outlet. A more efficient way to obtain water highly enriched in deuterium is by prolonged electrolysis of an aqueous solution. Because a deuteron (D+) has twice the mass of a proton (H+), it diffuses more slowly toward the electrode surface. Consequently, the gas evolved at the cathode is enriched in H, the species that diffuses more rapidly, favoring the formation of H2 over D2 or HD. Meanwhile, the solution becomes enriched in deuterium. Deuterium-rich water is called heavy water because the density of D2O (1.1044 g/cm3 at 25°C) is greater than that of H2O (0.99978 g/cm3). Heavy water was an important constituent of early nuclear reactors. (For more information on nuclear reactors, see Chapter 23)
Because deuterons diffuse so much more slowly, D2O will not support life and is actually toxic if administered to mammals in large amounts. The rate-limiting step in many important reactions catalyzed by enzymes involves proton transfer. The transfer of D+ is so slow compared with that of H+ because bonds to D break more slowly than those to H, so the delicate balance of reactions in the cell is disrupted. Nonetheless, deuterium and tritium are important research tools for biochemists. By incorporating these isotopes into specific positions in selected molecules, where they act as labels, or tracers, biochemists can follow the path of a molecule through an organism or a cell. Tracers can also be used to provide information about the mechanism of enzymatic reactions.
Bonding in Hydrogen and Hydrogen-Containing Compounds
The 1s1 electron configuration of hydrogen indicates a single valence electron. Because the 1s orbital has a maximum capacity of two electrons, hydrogen can form compounds with other elements in three ways (Figure 20.2.1 ):
- Losing its electron to form a proton (H+) with an empty 1s orbital. The proton is a Lewis acid that can accept a pair of electrons from another atom to form an electron-pair bond. In the acid–base reactions discussed in Chapter 16, for example, the proton always binds to a lone pair of electrons on an atom in another molecule to form a polar covalent bond. If the lone pair of electrons belongs to an oxygen atom of a water molecule, the result is the hydronium ion (H3O+).
- Accepting an electron to form a hydride ionThe anion formed when a hydrogen atom accepts an electron.(H−), which has a filled 1s2 orbital. Hydrogen reacts with relatively electropositive metals, such as the alkali metals (group 1) and alkaline earth metals (group 2), to form ionic hydrides, which contain metal cations and H− ions.
- Sharing its electron with an electron on another atom to form an electron-pair bond. With a half-filled 1s1 orbital, the hydrogen atom can interact with singly occupied orbitals on other atoms to form either a covalent or a polar covalent electron-pair bond, depending on the electronegativity of the other atom.
Figure 20.2.1 Three Types of Bonding in Compounds of Hydrogen Because of its 1s1 electron configuration and the fact that the 1s orbital can accommodate no more than two electrons, hydrogen can (a) bond to other elements by losing an electron to form a proton, which can accept a pair of electrons from a more electronegative atom to form a polar covalent bond; (b) gain an electron from an electropositive metal to form a hydride ion, resulting in an ionic hydride; or (c) share its half-filled 1s orbital with a half-filled orbital on another atom to form a covalent or a polar covalent electron-pair bond.
Hydrogen can also act as a bridge between two atoms. One familiar example is the hydrogen bondAn unusually strong dipole-dipole interaction (intermolecular force) that results when H is bonded to very electronegative elements such as O, N, and F., an electrostatic interaction between a hydrogen bonded to an electronegative atom and an atom that has one or more lone pairs of electrons (Figure 20.2.2 . An example of this kind of interaction is the hydrogen bonding network found in water (Figure 11.2.6). Hydrogen can also form a three-center bond (or electron-deficient bond)A bond in which a hydride ion bridges two electropositive atoms., in which a hydride bridges two electropositive atoms. Compounds that contain hydrogen bonded to boron and similar elements often have this type of bonding. The B–H–B units found in boron hydrides cannot be described in terms of localized electron-pair bonds. Because the H atom in the middle of such a unit can accommodate a maximum of only two electrons in its 1s orbital, the B–H–B unit can be described as containing a hydride that interacts simultaneously with empty sp3 orbitals on two boron atoms (Figure 20.2.3). In these bonds, only two bonding electrons are used to hold three atoms together, making them electron-deficient bonds. You encountered a similar phenomenon in the discussion of π bonding in ozone and the nitrite ion in Section 6.6. Recall that in both these cases, we used the presence of two electrons in a π molecular orbital extending over three atoms to explain the fact that the two O–O bond distances in ozone and the two N–O bond distances in nitrite are the same, which otherwise can be explained only by the use of resonance structures.
Figure 20.2.2 The Hydrogen Bond The covalent bond between hydrogen and a very electronegative element, such as nitrogen, oxygen, or fluorine, is highly polar. The resulting partial positive charge on H allows it to interact with a lone pair of electrons on another atom to form a hydrogen bond, which is typically a linear arrangement of the three atoms, with the hydrogen atom placed asymmetrically between the two heavier atoms.
Figure 20.2.3 A Three-Center Bond Uses Two Electrons to Link Three Atom In the B–H–B unit shown, a hydride, with a filled 1s orbital, interacts simultaneously with empty sp3 hybrids on the boron atoms of two BH3 units to give three molecular orbitals. The two bonding electrons occupy the lowest-energy (σ) bonding orbital, thereby holding all three atoms together.
Note the Pattern
Hydrogen can lose its electron to form H+, accept an electron to form H−, share its electron, hydrogen bond, or form a three-center bond.
Synthesis, Reactions, and Compounds of Hydrogen
The first known preparation of elemental hydrogen was in 1671, when Robert Boyle dissolved iron in dilute acid and obtained a colorless, odorless, gaseous product. Hydrogen was finally identified as an element in 1766, when Henry Cavendish showed that water was the sole product of the reaction of the gas with oxygen. The explosive properties of mixtures of hydrogen with air were not discovered until early in the 18th century; they partially caused the spectacular explosion of the hydrogen-filled dirigible Hindenburg in 1937 (Figure 20.2.4). Due to its extremely low molecular mass, hydrogen gas is difficult to condense to a liquid (boiling point = 20.3 K), and solid hydrogen has one of the lowest melting points known (13.8 K).
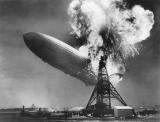
Figure 20.2.4 The Explosive Properties of Hydrogen When mixed with air and ignited by a spark, hydrogen gas can explode. The photograph shows the German dirigible Hindenburg on fire at Lakehurst, New Jersey, in 1937, after its hydrogen compartment was accidentally ignited as a consequence of an electrical discharge that caused the dirigible’s flammable skin to catch fire.
The most common way to produce small amounts of highly pure hydrogen gas in the laboratory was discovered by Boyle: reacting an active metal (M), such as iron, magnesium, or zinc, with dilute acid:
\( M\left ( s \right ) +2H^{+} \left ( aq \right ) \rightarrow H_{2}\left ( g \right ) + M^{2+}\left ( aq \right ) \tag{20.2.1}\)
Hydrogen gas can also be generated by reacting metals such as aluminum or zinc with a strong base:
\( Al\left ( s \right ) + OH^{-}\left ( aq \right ) + 3H_{2}O\left ( l \right ) \rightarrow \dfrac{3}{2}H_{2}\left ( g \right ) + \left [ Al\left ( OH_{4} \right ) \right ]^{-} \left ( aq \right ) \tag{20.2.2}\)
Solid commercial drain cleaners such as Drano use this reaction to generate gas bubbles that help break up clogs in a drainpipe. (For more information on redox reactions like that of Drano, see Section 19.2.) Hydrogen gas is also produced by reacting ionic hydrides with water. Because ionic hydrides are expensive, however, this reaction is generally used for only specialized purposes, such as producing HD gas by reacting a hydride with D2O:
\( MH \left (s \right ) + D_{2}O\left ( l \right ) \rightarrow HD\left ( g \right ) + OD^{-}\left ( aq \right ) + M^{+}\left ( aq \right ) \tag{20.2.3}\)
On an industrial scale, H2 is produced from methane by means of catalytic steam reforming, a method used to convert hydrocarbons to a mixture of CO and H2 known as synthesis gas, or syngas. (For more information on steam reforming, see Section 14.8) The process is carried out at elevated temperatures (800°C) in the presence of a nickel catalyst:
\( CH_{4} \left (g \right ) + H_{2}O\left ( l \right ) \rightarrow 3H_{2}\left ( g \right ) + NiCO\left ( g \right ) \tag{20.2.4}\)
Most of the elements in the periodic table form binary compounds with hydrogen, which are collectively referred to as hydrides. Binary hydrides in turn can be classified in one of three ways, each with its own characteristic properties. Covalent hydrides contain hydrogen bonded to another atom via a covalent bond or a polar covalent bond. Covalent hydrides are usually molecular substances that are relatively volatile and have low melting points. Ionic hydrides contain the hydride ion as the anion with cations derived from electropositive metals. Like most ionic compounds, they are typically nonvolatile solids that contain three-dimensional lattices of cations and anions. Unlike most ionic compounds, however, they often decompose to H2(g) and the parent metal after heating. Metallic hydrides are formed by hydrogen and less electropositive metals such as the transition metals. The properties of metallic hydrides are usually similar to those of the parent metal. Consequently, metallic hydrides are best viewed as metals that contain many hydrogen atoms present as interstitial impurities.
Note the Pattern
Covalent hydrides are relatively volatile and have low melting points; ionic hydrides are generally nonvolatile solids in a lattice framework.
Summary
The three isotopes of hydrogen—protium (1H or H), deuterium (2H or D), and tritium (3H or T)—have different physical properties. Deuterium and tritium can be used as tracers, substances that enable biochemists to follow the path of a molecule through an organism or a cell. Hydrogen can form compounds that contain a proton (H+), a hydride ion (H−), an electron-pair bond to H, a hydrogen bond, or a three-center bond (or electron-deficient bond), in which two electrons are shared between three atoms. Hydrogen gas can be generated by reacting an active metal with dilute acid, reacting Al or Zn with a strong base, or industrially by catalytic steam reforming, which produces synthesis gas, or syngas.
Key Takeaway
- Hydrogen can lose an electron to form a proton, gain an electron to form a hydride ion, or form a covalent bond or polar covalent electron-pair bond.
Conceptual Problems
-
Some periodic tables include hydrogen as a group 1 element, whereas other periodic tables include it as a group 17 element. Refer to the properties of hydrogen to propose an explanation for its placement in each group. In each case, give one property of hydrogen that would exclude it from groups 1 and 17.
-
If there were a planet where the abundances of D2O and H2O were reversed and life had evolved to adjust to this difference, what would be the effects of consuming large amounts of H2O?
-
Describe the bonding in a hydrogen bond and the central B–H bond in B2H7−. Why are compounds containing isolated protons unknown?
-
With which elements does hydrogen form ionic hydrides? covalent hydrides? metallic hydrides? Which of these types of hydrides can behave like acids?
-
Indicate which elements are likely to form ionic, covalent, or metallic hydrides and explain your reasoning:
- Sr
- Si
- O
- Li
- B
- Be
- Pd
- Al
-
Which has the higher ionization energy—H or H−? Why?
-
The electronegativities of hydrogen, fluorine, and iodine are 2.20, 3.98, and 2.66, respectively. Why, then, is HI a stronger acid than HF?
-
If H2O were a linear molecule, would the density of ice be less than or greater than that of liquid water? Explain your answer.
-
In addition to ion–dipole attractions, hydrogen bonding is important in solid crystalline hydrates, such as Na4XeO6·8H2O. Based on this statement, explain why anhydrous Na4XeO6 does not exist.
Answers
-
H has one electron in an s orbital, like the group 1 metals, but it is also one electron short of a filled principal shell, like the group 17 elements. Unlike the alkali metals, hydrogen is not a metal. Unlike the halogens, elemental hydrogen is not a potent oxidant.
-
-
-
-
- ionic; it is an alkaline earth metal.
- covalent; it is a semimetal.
- covalent; it is a nonmetal.
- ionic; it is an alkali metal.
- covalent; it is a semimetal.
- covalent; it is a period 2 alkaline earth metal.
- metallic; it is a transition metal.
- covalent; it is a group 13 metal.
-
-
-
-
Hydrogen bonding with waters of hydration will partially neutralize the negative charge on the terminal oxygen atoms on the XeO64− ion, which stabilizes the solid.
Structure and Reactivity
-
One of the largest uses of methane is to produce syngas, which is a source of hydrogen for converting nitrogen to ammonia. Write a complete equation for formation of syngas from methane and carbon dioxide. Calculate ΔG° for this reaction at 298 K and determine the temperature at which the reaction becomes spontaneous.
-
An alternative method of producing hydrogen is the water–gas shift reaction:
CO(g) + H2O(g) → CO2(g) + H2(g)Use Standard Thermodynamic Quantities to calculate ΔG° for this reaction at 298 K and determine the temperature at which the reaction changes from spontaneous to nonspontaneous (or vice versa).
-
Predict the products of each reaction at 25°C and then balance each chemical equation.
- CsH(s) + D2O(l) →
- CH3CO2H(l) + D2O(l) →
- H3PO4(aq) + D2O(l) →
- NH2CH2CO2H(s) + D2O(l) →
- NH4Cl(s) + D2O(l) →
-
Using heavy water (D2O) as the source of deuterium, how could you conveniently prepare
- D2SO4?
- LiD?
-
What are the products of reacting NaH with D2O? Do you expect the same products from reacting NaD and H2O? Explain your answer.
-
A 2.50 g sample of zinc metal reacts with 100.0 mL of 0.150 M HCl. What volume of H2 (in liters) is produced at 23°C and 729 mmHg?
-
A chemical reaction requires 16.8 L of H2 gas at standard temperature and pressure. How many grams of magnesium metal are needed to produce this amount of hydrogen gas?
-
Seawater contains 3.5% dissolved salts by mass and has an average density of 1.026 g/mL. The volume of the ocean is estimated to be 1.35 × 1021 L. Using the data in Table 19.2.1, calculate the total mass of deuterium in the ocean.
-
From the data in Table 19.2.1, determine the molarity of DOH in water. Do you expect the molarity of D2O in water to be similar? Why or why not?
-
From the data in Table 19.2.1 calculate how many liters of water you would have to evaporate to obtain 1.0 mL of TOD (tritium-oxygen-deuterium). The density of TOD is 1.159 g/mL.
Contributors
- Anonymous
Modified by Joshua B. Halpern