6.3: Lewis Concept and Frontier Orbitals
- Page ID
- 326213
\( \newcommand{\vecs}[1]{\overset { \scriptstyle \rightharpoonup} {\mathbf{#1}} } \)
\( \newcommand{\vecd}[1]{\overset{-\!-\!\rightharpoonup}{\vphantom{a}\smash {#1}}} \)
\( \newcommand{\id}{\mathrm{id}}\) \( \newcommand{\Span}{\mathrm{span}}\)
( \newcommand{\kernel}{\mathrm{null}\,}\) \( \newcommand{\range}{\mathrm{range}\,}\)
\( \newcommand{\RealPart}{\mathrm{Re}}\) \( \newcommand{\ImaginaryPart}{\mathrm{Im}}\)
\( \newcommand{\Argument}{\mathrm{Arg}}\) \( \newcommand{\norm}[1]{\| #1 \|}\)
\( \newcommand{\inner}[2]{\langle #1, #2 \rangle}\)
\( \newcommand{\Span}{\mathrm{span}}\)
\( \newcommand{\id}{\mathrm{id}}\)
\( \newcommand{\Span}{\mathrm{span}}\)
\( \newcommand{\kernel}{\mathrm{null}\,}\)
\( \newcommand{\range}{\mathrm{range}\,}\)
\( \newcommand{\RealPart}{\mathrm{Re}}\)
\( \newcommand{\ImaginaryPart}{\mathrm{Im}}\)
\( \newcommand{\Argument}{\mathrm{Arg}}\)
\( \newcommand{\norm}[1]{\| #1 \|}\)
\( \newcommand{\inner}[2]{\langle #1, #2 \rangle}\)
\( \newcommand{\Span}{\mathrm{span}}\) \( \newcommand{\AA}{\unicode[.8,0]{x212B}}\)
\( \newcommand{\vectorA}[1]{\vec{#1}} % arrow\)
\( \newcommand{\vectorAt}[1]{\vec{\text{#1}}} % arrow\)
\( \newcommand{\vectorB}[1]{\overset { \scriptstyle \rightharpoonup} {\mathbf{#1}} } \)
\( \newcommand{\vectorC}[1]{\textbf{#1}} \)
\( \newcommand{\vectorD}[1]{\overrightarrow{#1}} \)
\( \newcommand{\vectorDt}[1]{\overrightarrow{\text{#1}}} \)
\( \newcommand{\vectE}[1]{\overset{-\!-\!\rightharpoonup}{\vphantom{a}\smash{\mathbf {#1}}}} \)
\( \newcommand{\vecs}[1]{\overset { \scriptstyle \rightharpoonup} {\mathbf{#1}} } \)
\( \newcommand{\vecd}[1]{\overset{-\!-\!\rightharpoonup}{\vphantom{a}\smash {#1}}} \)
\(\newcommand{\avec}{\mathbf a}\) \(\newcommand{\bvec}{\mathbf b}\) \(\newcommand{\cvec}{\mathbf c}\) \(\newcommand{\dvec}{\mathbf d}\) \(\newcommand{\dtil}{\widetilde{\mathbf d}}\) \(\newcommand{\evec}{\mathbf e}\) \(\newcommand{\fvec}{\mathbf f}\) \(\newcommand{\nvec}{\mathbf n}\) \(\newcommand{\pvec}{\mathbf p}\) \(\newcommand{\qvec}{\mathbf q}\) \(\newcommand{\svec}{\mathbf s}\) \(\newcommand{\tvec}{\mathbf t}\) \(\newcommand{\uvec}{\mathbf u}\) \(\newcommand{\vvec}{\mathbf v}\) \(\newcommand{\wvec}{\mathbf w}\) \(\newcommand{\xvec}{\mathbf x}\) \(\newcommand{\yvec}{\mathbf y}\) \(\newcommand{\zvec}{\mathbf z}\) \(\newcommand{\rvec}{\mathbf r}\) \(\newcommand{\mvec}{\mathbf m}\) \(\newcommand{\zerovec}{\mathbf 0}\) \(\newcommand{\onevec}{\mathbf 1}\) \(\newcommand{\real}{\mathbb R}\) \(\newcommand{\twovec}[2]{\left[\begin{array}{r}#1 \\ #2 \end{array}\right]}\) \(\newcommand{\ctwovec}[2]{\left[\begin{array}{c}#1 \\ #2 \end{array}\right]}\) \(\newcommand{\threevec}[3]{\left[\begin{array}{r}#1 \\ #2 \\ #3 \end{array}\right]}\) \(\newcommand{\cthreevec}[3]{\left[\begin{array}{c}#1 \\ #2 \\ #3 \end{array}\right]}\) \(\newcommand{\fourvec}[4]{\left[\begin{array}{r}#1 \\ #2 \\ #3 \\ #4 \end{array}\right]}\) \(\newcommand{\cfourvec}[4]{\left[\begin{array}{c}#1 \\ #2 \\ #3 \\ #4 \end{array}\right]}\) \(\newcommand{\fivevec}[5]{\left[\begin{array}{r}#1 \\ #2 \\ #3 \\ #4 \\ #5 \\ \end{array}\right]}\) \(\newcommand{\cfivevec}[5]{\left[\begin{array}{c}#1 \\ #2 \\ #3 \\ #4 \\ #5 \\ \end{array}\right]}\) \(\newcommand{\mattwo}[4]{\left[\begin{array}{rr}#1 \amp #2 \\ #3 \amp #4 \\ \end{array}\right]}\) \(\newcommand{\laspan}[1]{\text{Span}\{#1\}}\) \(\newcommand{\bcal}{\cal B}\) \(\newcommand{\ccal}{\cal C}\) \(\newcommand{\scal}{\cal S}\) \(\newcommand{\wcal}{\cal W}\) \(\newcommand{\ecal}{\cal E}\) \(\newcommand{\coords}[2]{\left\{#1\right\}_{#2}}\) \(\newcommand{\gray}[1]{\color{gray}{#1}}\) \(\newcommand{\lgray}[1]{\color{lightgray}{#1}}\) \(\newcommand{\rank}{\operatorname{rank}}\) \(\newcommand{\row}{\text{Row}}\) \(\newcommand{\col}{\text{Col}}\) \(\renewcommand{\row}{\text{Row}}\) \(\newcommand{\nul}{\text{Nul}}\) \(\newcommand{\var}{\text{Var}}\) \(\newcommand{\corr}{\text{corr}}\) \(\newcommand{\len}[1]{\left|#1\right|}\) \(\newcommand{\bbar}{\overline{\bvec}}\) \(\newcommand{\bhat}{\widehat{\bvec}}\) \(\newcommand{\bperp}{\bvec^\perp}\) \(\newcommand{\xhat}{\widehat{\xvec}}\) \(\newcommand{\vhat}{\widehat{\vvec}}\) \(\newcommand{\uhat}{\widehat{\uvec}}\) \(\newcommand{\what}{\widehat{\wvec}}\) \(\newcommand{\Sighat}{\widehat{\Sigma}}\) \(\newcommand{\lt}{<}\) \(\newcommand{\gt}{>}\) \(\newcommand{\amp}{&}\) \(\definecolor{fillinmathshade}{gray}{0.9}\)Lewis Acids and Bases
The Lewis acid base concept generalizes the Brønsted and solvent system acid base concepts by describing acid-base reactions in terms of the donation and acceptance of an electron pair. Under the Lewis definition Lewis acids are electron pair acceptors and Lewis bases are electron pair donors. In Lewis acid-base reactions a Lewis base donates an electron pair to the Lewis acid, which accepts it. The reaction between borane, BH3, and NH3 is the classic example:
Definition: Lewis acid
A species that is an electron pair acceptor
Definition: Lewis base
A species that is an electron pair donor
In this case the Lewis acid-base reaction results in the formation of a bond between BH3, and NH3 . When the acid and base combine to form a larger unit that unit is said to be an adduct and the resulting bond is said to be a coordinate covalent or dative bond. Such coordinate covalent bonds are sometimes represented by an arrow that indicates the direction of electron donation from the base to the acid. For instance the reaction between BH3, and NH3 could also have been written as
The arrow notation for the coordinate covalent bond is really just a convenient formalism - a bookkeeping tool to help keep track of where the electrons came from and where they might return if the reverse reaction occurs.
For example, in Brønsted acid base reactions the hydrogen ion is an acid because it accepts an electron pair from the Brønsted base. Consequently under the Lewis acid-base concept in Brønsted acid base reactions involve the formation of an adduct between \(H^+\) and a base.
\[\]
The Lewis acid base concept nicely explains ionization reactions involving nonaqueous solvents. For instance, the autoionization of SOCl2 as an acid-base reaction between two SOCl2 molecules.
\[\]
Example \(\PageIndex{1}\)
Explain how the autoionization of SOCl2 is a Lewis acid-base displacement reaction.
Solution
In the autoionization of SOCl2 the pair of electrons donated comes from the S-Cl bond and the S-Cl bond is broken to give a lone pair bearing Cl- base and a SOCl+ Lewis acid fragment. If you are having trouble seeing how this works it can be instructive to consider the reverse of this process. It is a Lewis acid-base reaction to give a Lewis acid-base adduct:
From the autoionization reaction it is also apparent that \(SOCl_2\) itself acts as a Lewis acid towards the liberated \(Cl^-\). So the autoionization reaction involves a transfer of the base \(Cl^-\) base between the two Lewis acids - a Lewis acid-base displacement reaction.
Lewis acid-base model and chemical reactions
It can be helpful to keep several distinctions in mind when using the Lewis acid-base concept to describe chemical reactions.
- Many Lewis-Acid base reactions are displacement reactions. This is because the hydrogen ion is usually bound to something at the start of the reaction. In such cases the Lewis acid \(H^+\) unit is transferred from one Lewis base and another. Such acid-base reactions are sometimes called displacement reactions since the base group in the initial Lewis acid-base complex is displaced by the incoming Lewis base to generate another complex.
\[\]
- Substances are sometimes considered amphoteric because they exhibit Lewis acidity and basicity at different types of atomic centers. The classic example is aluminum hydroxide, \(Al(OH)_3\). In water \(Al(OH)_3\) can act as a Lewis acid towards OH- ion. The reaction occurs by formation of an adduct at \(Al(OH)_3\)'s Al3+ center:
\[Al(OH)_3 + OH^- \rightarrow Al(OH)_4^-\]
Notice that in addition to acting as a Lewis acid, in this reaction \(Al(OH)_3\)
- does not act as a Brønsted acid or base since no H+ ion transfer occurs
- but does act as an Arhennius acid since OH- ion is consumed from solution, decreasing [OH-] and increasing [H+]
In water \(Al(OH)_3\) also acts as a Lewis base towards H+ through the lone pairs on its hydroxide ligands.
\[Al(OH)_3 + 3 H^+ + 3 H_2O \rightarrow Al(H_2O)_6^{3+}\]
Notice that in addition to acting as a Lewis base, in this reaction \(Al(OH)_3\) also acts as a
- Brønsted base since a H+ ion is transferred onto the OH- ligand
- Arhennius base since H+ ion is consumed from solution, decreasing [H+]
- Lewis acid at its Al3+ center since Al-O metal-ligand bonds are formed
Frontier Orbitals
Another way the Lewis acid-base concept is widely employed for understanding chemical reactivity is through the frontier orbital approach to chemical reactions. The frontier orbital concept is a simplified version of molecular orbital theory where chemical bonding and reactivity are visualized in terms of the interactions between frontier molecular orbitals on the chemical species undergoing an interaction (e.g. molecules, atoms, ions, or groups as they interact to form a bond or undergo a reaction). Frontier orbitals are those at the frontier between occupied and unoccupied. They are often taken to be the highest energy occupied and lowest energy unoccupied molecular orbitals, called the HOMO and LUMO levels. However, it can sometimes be more convenient to think about them as atomic orbitals or Valence Bond approach-derived orbitals. When developing rough qualitative frontier orbital descriptions of the orbital interactions involved in a given system the choice of what types of orbitals to use is often a matter of what is the most informative and convenient.
In particular the frontier orbital concept envisions a Lewis acid-base interaction as involving an interaction between some of the frontier orbitals of the Lewis acid and base, specifically the donation of electrons from the bases' HOMO level into the acid's LUMO level. For example, in the frontier orbital approach adduct formation between \(NH_3\) and \(BH_3\) involves the donation of electrons from ammonia's HOMO into BH3's LUMO level.
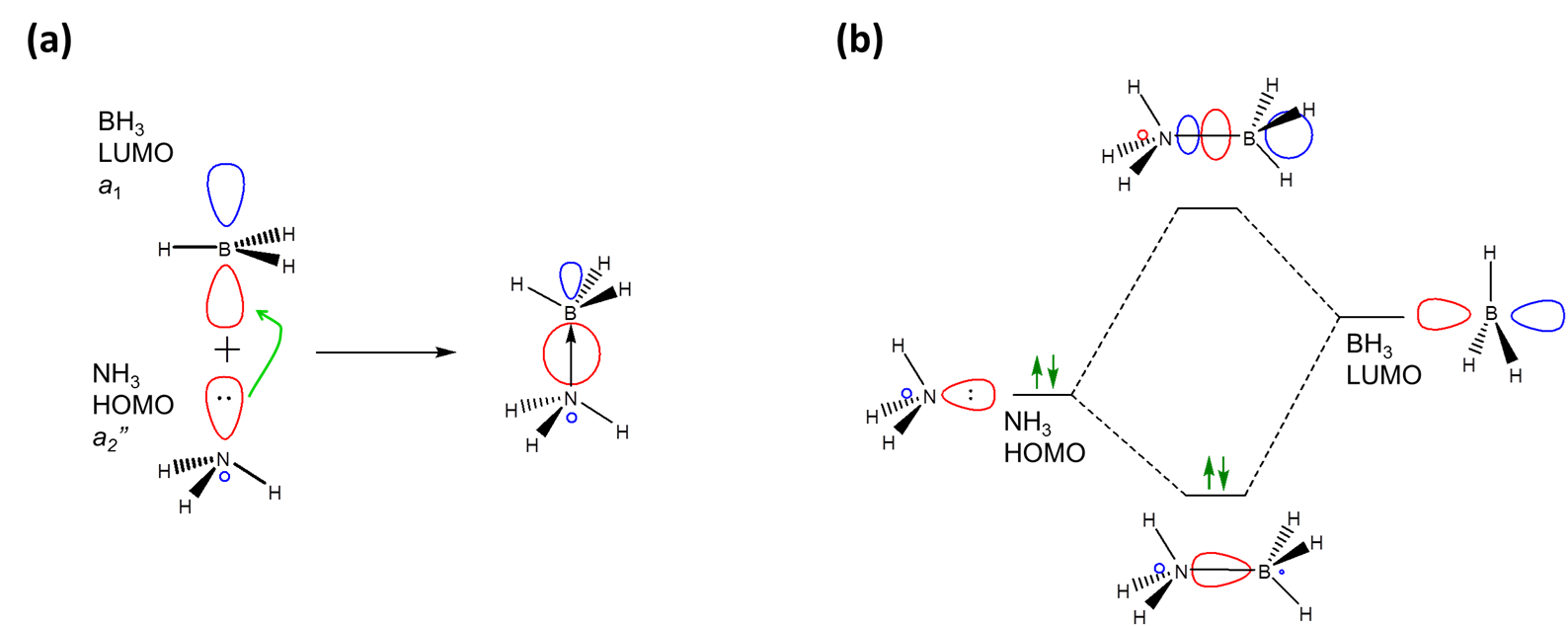
As expected when two orbitals of the appropriate symmetry combine, the result of the interaction is the formation of a lower energy bonding orbital between the acid and base (Figure \( \PageIndex{1}\)). Since it is occupied by an electron the net result of the interaction is the lowering of the base's lone pair as it interacts with the Lewis acid. In this case the interaction just follows the general pattern for Lewis-Acid base adduct formation, which is shown in Figure \(\PageIndex{2}\).
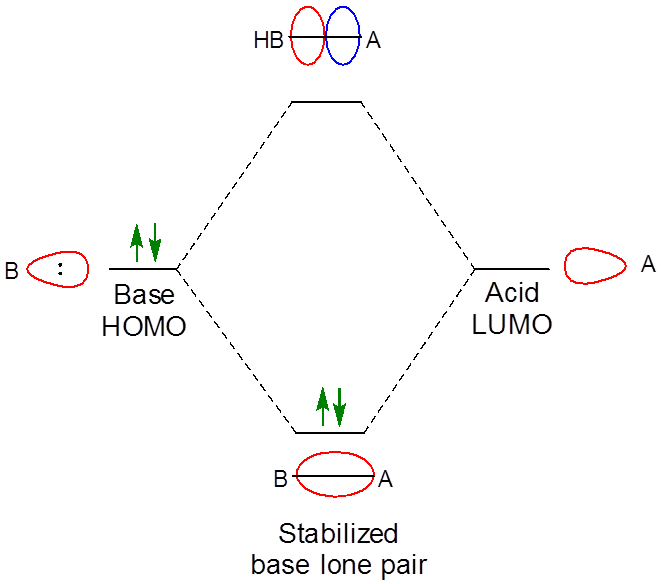
The frontier orbital concept illuminates the orbital interactions involved in reactions. For instance, from a frontier orbital perspective the alkyl halide substitution reaction between hydroxide and CH3Cl via the SN2 mechanism involves a Lewis acid-base interaction:
Notice how the frontier orbital approach even explains the displacement of the chloride leaving group. The donation of electrons from the hydroxide HOMO populates the antibonding CH3Cl LUMO, breaking the C-Cl bond.
Using the frontier orbital approach it becomes apparent that pericyclic reactions are Lewis acid-base reactions. For example, in the Diels-Alder reaction the dienophile acts as a Lewis base and the diene as a Lewis acid.
The orbital interactions involved in a given reaction can include both reactants acting as both an acid and base. In these cases the HOMO of each reactant interacts with the LUMO of the other. A good example of this involves \( \Pi \)-type interactions between a metal ion with occupied d orbitals and a pi-acceptor ligand. The ligand acts as a base and the metal as an acid to give a M-CO single bond.
However, the metal can also act as a base towards the ligand LUMO ( \( \pi ^*\) ) orbitals. Bonding between metals and ligands will be discussed further in chapter 9
Substituent Effects
The electrons donated from a Lewis base to a Lewis acid in a Lewis acid-base reaction are donated and accepted at particular atomic centers. For instance, the formation of an adduct between ammonia and BF3 involves the donation of the lone pair on the ammonia nitrogen atom to the Lewis acid site on BF3.
Because Lewis acid-base reactions involve electron donation and acceptance at particular sites subsistent groups which alter the electron density at a site through inductively donating or withdrawing electron density will affect the Lewis acid-base properties of that site. For instance, the BF3 affinities of 4-substituted pyridines increase slightly as the substituent on the aromatic ring is changed from electron donating Me to electron withdrawing CF3.
Substituent inductive effects are much as one might predict. Since Lewis bases donate electron pairs and Lewis acids accept them:
- electron withdrawing substituents tend to decrease the Lewis basicity of basic sites while electron donating substituents increase site Lewis basicity by making them more electron rich.
- electron withdrawing substituents increase the Lewis acidity of acidic sites by making those sites more electron deficient while electron donating substituents tend decrease Lewis acidity by making sites less electron deficient.
Nevertheless it can be difficult to predict substituent-based trends in Lewis acidity and basicity by inductive effects alone. This is because inductive effects are modest and often exists in competition with other substituent effects, such as
- Steric effects
- Hardness effects
- \(\pi\)-donation and acceptance effects, which can increase or decrease electron density at a given site as well as create an energy barrier for any structural distortions that might occur on adduct formation.
\(\pi\) Donation and Acceptance and Lewis Acid-Base Affinity
As with \(\sigma\)-based induction effects\(\pi\)-donation tends to increase Lewis basicity and decrease Lewis acditiy while \(\pi\)-withdrawal tends to decrease Lewis basicity and increase Lewis acidity. However, care needs to be taken in assessing the effect of \(\pi\)-donation effects on Lewis acidity and basicity. For example, some textbooks claim that the Lewis acidity of boron trihalides is dominated by the reduction of boron acidity through\(\pi\)-donation from the halide substituents:
According to this explanation the extent of this \(\pi\)-donation decreases down the halogen group as the boron-halogen bond distance decreases. This is consistent with the observed trend in Lewis acidity of the boron trihalides towards most bases, which runs counter to that suggested by inductive effects alone:
BI3 > BBr3 > BCl3 >> BF3
However, computation work suggests that this explanation is incorrect since
- atomic size effects are important mainly for substituents in which the connected atom is row 3+ or higher
- atomic size effects mainly involve changes in the extent of \(\sigma\)-overlap. In other words the larger halogens are less able to reduce the electron deficiency at the boron center through \(\sigma\) interactions while \(\pi\)-interactions play little or no role.
References:
- Plumley, J. A.; Evanseck, J. D., Periodic Trends and Index of Boron Lewis Acidity. The Journal of Physical Chemistry A 2009, 113 (20), 5985-5992.
- Jupp, A. R.; Johnstone, T. C.; Stephan, D. W., Improving the Global Electrophilicity Index (GEI) as a Measure of Lewis Acidity. Inorganic Chemistry 2018, 57 (23), 14764-14771.
Conjugate Bases of Brønsted Superacids
The nonreactivity of Brønsted superacids' conjugate bases towards hydrogen ions is often mirrored in nonreactivity towards other Lewis Acids/electrophiles, most notably metals. This makes these substances useful as inert or noncoordinating ions, although since all are reactive towards a suitably electrophilic centers they are perhaps better understood as weakly coordinating.
A number of noncoordinating anions are commonly used in synthetic and other applications. The conjugate base of perchloric acid, perchlorate, was a common noncoordinating inert anion in classical coordination chemistry and continues to be used widely in electrochemistry. However metal perchlorate salts can be explosive, so synthesis of metal salts with perchlorate anions, especially on large scales is much less common in modern inorganic chemistry. In contrast, the conjugate bases of triflic acid, hexaflouroboric acid, and tetrafloroboric acid are now more commonly used as counterions for when isolating reactive cations.
Even less reactive noncoordinating anions include derivatives of tetraphenylborate, particularly those with electron withdrawing substituents.
Other classes of noncoordinating ions include floroantimonate clusters, derivatives of the carborane anion (\(CB_{11}H_{11}^-\)), and flourinated aluminum tetraalkoxides.
References:
- Engesser, T. A.; Lichtenthaler, M. R.; Schleep, M.; Krossing, I., Reactive p-block cations stabilized by weakly coordinating anions. Chemical Society Reviews 2016, 45 (4), 789-899.
Lewis Acid-Base Adducts
When a Lewis acid-base adduct is formed electron density and negative charge is transferred from the Lewis base to the acid.
\[\]
For example, the formation of an adduct between borane and ammonia involves the transfer of a small amount of electron density, as shown by the movement of negative charge from \(NH_3\) to \(BH_3\) depicted in Figure \( \PageIndex{3}\).
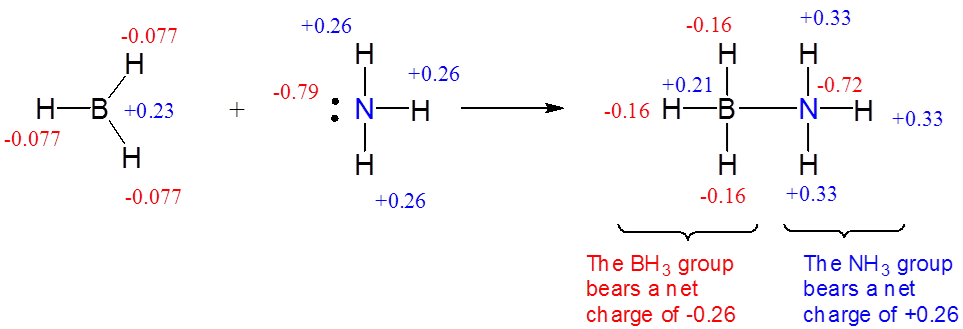
In many weakly bound Lewis acid-base complexes the transfer of electron density and consequently, charge, from the base group to the acid group is only partial:
\[A~+~B~\rightleftharpoons~A^{\delta-}B^{\delta+}\]
Such Lewis acid-base adducts are commonly called charge transfer complexes (CT complexes) or donor-acceptor complexes (DA complexes). In these
- the base is called the donor (D) since it is a net donor of electrons and, consequently, their negative charge
- the acid is called the acceptor (A) since it is a net acceptor of electrons and, consequently, their negative charge
A particularly well-known class of charge transfer complexes are the iodine charge transfer complexes. In iodine charge transfer complexes the \(I_2\) acts as a Lewis acid. This is possible since iodine is a Row 3+ element and so is capable of forming hypervalent complexes on reaction with a Lewis base. For example, \(I_2\) reacts with \(I^-\) to give the triiodide ion.
\[\]
Triiodie is well-known from introductory chemistry from the bright blue color that appears when the triiodie is complexes with starch to give the dark blue starch-iodide complex.
In contrast to stable triiodide anion, iodine charge transfer complexes are only weakly associated. Complexes between iodine and amines are a well known example:
\[\]
Iodine also forms weakly-associated charge transfer complexes with many solvents. For instance, iodine can weakly associate with both acetone and benzene:
Steric Effects
Steric effects can influence the ability of a Lewis acid or base to form adducts by introducing:
- front strain (F-strain) whereby bulky groups make it difficult for the Lewis acid and Lewis base centers to approach and interact.
- back strain (B-strain) associated with steric interactions that do not directly impede the Lewis acid and base centers from interacting but instead occur as the acid and base rearrange on adduct formation. For instance, when trivalent boron compounds form adducts with amines the boron center changes from a more open trigonal pyramidal geometry to a more hindered tetrahedral one:
- internal strain (I-strain) is also associated with the geometry changes incident on adduct formation. However, while B-strain involves direct steric clashes that occur on adduct formation I-strain is the strain involved in deforming bond and torsional angles away from more stable local geometries. Thus it is more important for Lewis base centers embedded in rings or clusters.
References
1. Alder, R. W., Strain effects on amine basicities. Chemical Reviews 1989, 89 (5), 1215-1223.
Contributors and Attributions
Stephen M. Contakes, Westmont College
Consistent with the policy for original artwork made as part of this project, all unlabeled drawings of chemical structures are by Stephen Contakes and licensed under a Creative Commons Attribution 4.0 International License.