16.9: Climate Mitigation Strategies
- Page ID
- 434975
\( \newcommand{\vecs}[1]{\overset { \scriptstyle \rightharpoonup} {\mathbf{#1}} } \)
\( \newcommand{\vecd}[1]{\overset{-\!-\!\rightharpoonup}{\vphantom{a}\smash {#1}}} \)
\( \newcommand{\id}{\mathrm{id}}\) \( \newcommand{\Span}{\mathrm{span}}\)
( \newcommand{\kernel}{\mathrm{null}\,}\) \( \newcommand{\range}{\mathrm{range}\,}\)
\( \newcommand{\RealPart}{\mathrm{Re}}\) \( \newcommand{\ImaginaryPart}{\mathrm{Im}}\)
\( \newcommand{\Argument}{\mathrm{Arg}}\) \( \newcommand{\norm}[1]{\| #1 \|}\)
\( \newcommand{\inner}[2]{\langle #1, #2 \rangle}\)
\( \newcommand{\Span}{\mathrm{span}}\)
\( \newcommand{\id}{\mathrm{id}}\)
\( \newcommand{\Span}{\mathrm{span}}\)
\( \newcommand{\kernel}{\mathrm{null}\,}\)
\( \newcommand{\range}{\mathrm{range}\,}\)
\( \newcommand{\RealPart}{\mathrm{Re}}\)
\( \newcommand{\ImaginaryPart}{\mathrm{Im}}\)
\( \newcommand{\Argument}{\mathrm{Arg}}\)
\( \newcommand{\norm}[1]{\| #1 \|}\)
\( \newcommand{\inner}[2]{\langle #1, #2 \rangle}\)
\( \newcommand{\Span}{\mathrm{span}}\) \( \newcommand{\AA}{\unicode[.8,0]{x212B}}\)
\( \newcommand{\vectorA}[1]{\vec{#1}} % arrow\)
\( \newcommand{\vectorAt}[1]{\vec{\text{#1}}} % arrow\)
\( \newcommand{\vectorB}[1]{\overset { \scriptstyle \rightharpoonup} {\mathbf{#1}} } \)
\( \newcommand{\vectorC}[1]{\textbf{#1}} \)
\( \newcommand{\vectorD}[1]{\overrightarrow{#1}} \)
\( \newcommand{\vectorDt}[1]{\overrightarrow{\text{#1}}} \)
\( \newcommand{\vectE}[1]{\overset{-\!-\!\rightharpoonup}{\vphantom{a}\smash{\mathbf {#1}}}} \)
\( \newcommand{\vecs}[1]{\overset { \scriptstyle \rightharpoonup} {\mathbf{#1}} } \)
\( \newcommand{\vecd}[1]{\overset{-\!-\!\rightharpoonup}{\vphantom{a}\smash {#1}}} \)
\(\newcommand{\avec}{\mathbf a}\) \(\newcommand{\bvec}{\mathbf b}\) \(\newcommand{\cvec}{\mathbf c}\) \(\newcommand{\dvec}{\mathbf d}\) \(\newcommand{\dtil}{\widetilde{\mathbf d}}\) \(\newcommand{\evec}{\mathbf e}\) \(\newcommand{\fvec}{\mathbf f}\) \(\newcommand{\nvec}{\mathbf n}\) \(\newcommand{\pvec}{\mathbf p}\) \(\newcommand{\qvec}{\mathbf q}\) \(\newcommand{\svec}{\mathbf s}\) \(\newcommand{\tvec}{\mathbf t}\) \(\newcommand{\uvec}{\mathbf u}\) \(\newcommand{\vvec}{\mathbf v}\) \(\newcommand{\wvec}{\mathbf w}\) \(\newcommand{\xvec}{\mathbf x}\) \(\newcommand{\yvec}{\mathbf y}\) \(\newcommand{\zvec}{\mathbf z}\) \(\newcommand{\rvec}{\mathbf r}\) \(\newcommand{\mvec}{\mathbf m}\) \(\newcommand{\zerovec}{\mathbf 0}\) \(\newcommand{\onevec}{\mathbf 1}\) \(\newcommand{\real}{\mathbb R}\) \(\newcommand{\twovec}[2]{\left[\begin{array}{r}#1 \\ #2 \end{array}\right]}\) \(\newcommand{\ctwovec}[2]{\left[\begin{array}{c}#1 \\ #2 \end{array}\right]}\) \(\newcommand{\threevec}[3]{\left[\begin{array}{r}#1 \\ #2 \\ #3 \end{array}\right]}\) \(\newcommand{\cthreevec}[3]{\left[\begin{array}{c}#1 \\ #2 \\ #3 \end{array}\right]}\) \(\newcommand{\fourvec}[4]{\left[\begin{array}{r}#1 \\ #2 \\ #3 \\ #4 \end{array}\right]}\) \(\newcommand{\cfourvec}[4]{\left[\begin{array}{c}#1 \\ #2 \\ #3 \\ #4 \end{array}\right]}\) \(\newcommand{\fivevec}[5]{\left[\begin{array}{r}#1 \\ #2 \\ #3 \\ #4 \\ #5 \\ \end{array}\right]}\) \(\newcommand{\cfivevec}[5]{\left[\begin{array}{c}#1 \\ #2 \\ #3 \\ #4 \\ #5 \\ \end{array}\right]}\) \(\newcommand{\mattwo}[4]{\left[\begin{array}{rr}#1 \amp #2 \\ #3 \amp #4 \\ \end{array}\right]}\) \(\newcommand{\laspan}[1]{\text{Span}\{#1\}}\) \(\newcommand{\bcal}{\cal B}\) \(\newcommand{\ccal}{\cal C}\) \(\newcommand{\scal}{\cal S}\) \(\newcommand{\wcal}{\cal W}\) \(\newcommand{\ecal}{\cal E}\) \(\newcommand{\coords}[2]{\left\{#1\right\}_{#2}}\) \(\newcommand{\gray}[1]{\color{gray}{#1}}\) \(\newcommand{\lgray}[1]{\color{lightgray}{#1}}\) \(\newcommand{\rank}{\operatorname{rank}}\) \(\newcommand{\row}{\text{Row}}\) \(\newcommand{\col}{\text{Col}}\) \(\renewcommand{\row}{\text{Row}}\) \(\newcommand{\nul}{\text{Nul}}\) \(\newcommand{\var}{\text{Var}}\) \(\newcommand{\corr}{\text{corr}}\) \(\newcommand{\len}[1]{\left|#1\right|}\) \(\newcommand{\bbar}{\overline{\bvec}}\) \(\newcommand{\bhat}{\widehat{\bvec}}\) \(\newcommand{\bperp}{\bvec^\perp}\) \(\newcommand{\xhat}{\widehat{\xvec}}\) \(\newcommand{\vhat}{\widehat{\vvec}}\) \(\newcommand{\uhat}{\widehat{\uvec}}\) \(\newcommand{\what}{\widehat{\wvec}}\) \(\newcommand{\Sighat}{\widehat{\Sigma}}\) \(\newcommand{\lt}{<}\) \(\newcommand{\gt}{>}\) \(\newcommand{\amp}{&}\) \(\definecolor{fillinmathshade}{gray}{0.9}\)- Identify the difference between adaptation and mitigation in dealing with climate change.
- Discuss different adaptation strategies that can be put in place to deal with a changing environment.
- Describe alternative energy sources that can be put in place to mitigate carbon dioxide emissions.
- Describe how carbon sequestration can lower carbon dioxide emissions.
- Identify different strategies that you as an individual can put in place to reduce greenhouse gas emissions.
While the situation surrounding global climate change is in serious need of our attention, it is important to realize that many scientists, leaders, and concerned citizens are making solutions to climate change part of their life’s work. The two solutions to the problems caused by climate change are adaptation and mitigation, and we will likely need a combination of both in order to prosper in the future.
Adaptation strategies
We know that climate change is already occurring, as we can see and feel the effects of it. For this reason, it is essential to be able to adapt to our changing environment. This means that we must change our behaviors in response to the changing environment around us. Adaption strategies will vary greatly by region, depending on the largest specific impacts in that area. For example, in the city of Delhi, India, a dramatic decrease in rainfall is projected over the next century. This city will likely need to implement policies and practices relating to conservation of water such as rainwater harvesting, water re-use, and increased irrigation efficiency. Rain-limited cities near oceans, such as Los Angeles, California may choose to use desalination to provide drinking water to their citizens. Desalination involves taking the salt out of seawater to make it potable.
Cities with low elevations near oceans may need to implement adaptation strategies to cope with rising sea levels, from seawalls and levees to relocation of citizens. One adaptation strategy gaining use is the creation or conservation of wetlands, which provide natural protection against storm surges and flooding.
Green chemistry, biochemistry, and biology can be used to deal with global warming when it occurs. Crops, fertilizers, and pesticides can be developed that enable plants to grow under the drought conditions that will follow global warming in some locations. Another approach is the development of salt-tolerant crops that can be grown on soil irrigated with saline water, where fresh water supplies are limited.
Mitigation Strategies - Energy Usage
In general, a strategy to mitigate climate change is one that reduces the amount of greenhouse gases in the atmosphere or prevents additional emissions. As noted in the previous section, the most significant of these is carbon dioxide. One way to reduce the release of carbon dioxide is through the use of alternative energy sources.
Until about 1800 the sun provided virtually all of the energy used by humans. Biomass produced by photosynthesis was burned for heat or used to feed horses, oxen, and even humans who provided muscle power. Because the carbon dioxide generated by this burning was replacing that used to grow the plants, there was no net increase in atmospheric carbon dioxide levels. Wind resulting from uneven solar heating of air masses powered sailing ships and windmills. The solar powered hydrologic cycle produced running water that drove water wheels.
Around 1800 the dramatically increased use of coal began the era of fossil fuel energy sources. This use was enabled by the invention of the steam engine, which provided abundant and reliable power for stationary sources and locomotives and the power used for mining the coal. By 1900 coal was the dominant energy source in industrialized societies, but petroleum, especially well suited as fuel for the newly developed internal combustion engine, began a century of rapid development, becoming the favored fuel for transportation. Often encountered in wells drilled for petroleum, natural gas also developed during the 1900s, predominantly as a fuel for non-transportation needs. During this century hydroelectric power took on a significant share of electrical power production and remains a substantial renewable energy source. By around 1975, nuclear energy was supplying significant amounts of electricity and has maintained an appreciable share worldwide until the present. Miscellaneous renewable sources including geothermal and, more recently, solar and wind energy are making increasing contributions to total energy supply. Biomass still contributes significantly to the total of the sources of energy used and will become even more important as emphasis on renewable sources increases.
Despite so many different sources of energy that are available to us, most are not directly useful and must be converted to other forms. Therefore, much of what is done with energy involves changing it from one form to another. This is not always done efficiently using current technologies. If those efficiencies can be improved, less energy needs to be generated to do the same work. This has been seen in heat engines such as automobile engines and gas turbines by higher combustion temperatures made possible by improved materials and heat-resistant lubricating oils. Computerized design and operation of engines enabling optimum ignition timing, valve timing, and fuel injection have made possible extremely efficient engines with vastly improved gas mileage.
Much of the lost energy comes in the form of heat. In stationary power plants much of this energy can be reclaimed for heating buildings or chemical processes with combined power cycles as illustrated in Figure \(\PageIndex{1}\). Typically, in combined power cycle installations, gas or fuel oil is burned in a turbine engine that is much like the engine of a turboprop airplane, and the rotating shaft of this engine is coupled to a generator to produce electricity. The hot exhaust gases from the combustion turbine can be injected into a boiler where their heat turns liquid water to steam. This steam can be run through a steam turbine coupled to a generator to produce more electricity. Steam leaving the steam turbine still contains a lot of heat, and can be conveyed to homes and other structures for heating. The water condensed from this steam is pure and is recycled to the boiler, thus minimizing the amount of makeup boiler feedwater, which requires expensive treatment to make it suitable for use in boilers. Such a system as the one described is in keeping with the best practice of industrial ecology. Heating with steam that has been through a steam turbine, a concept known as district heating, is commonly practiced in Europe (and many university campuses in the U.S.) and can save large amounts of fuel otherwise required for heating.
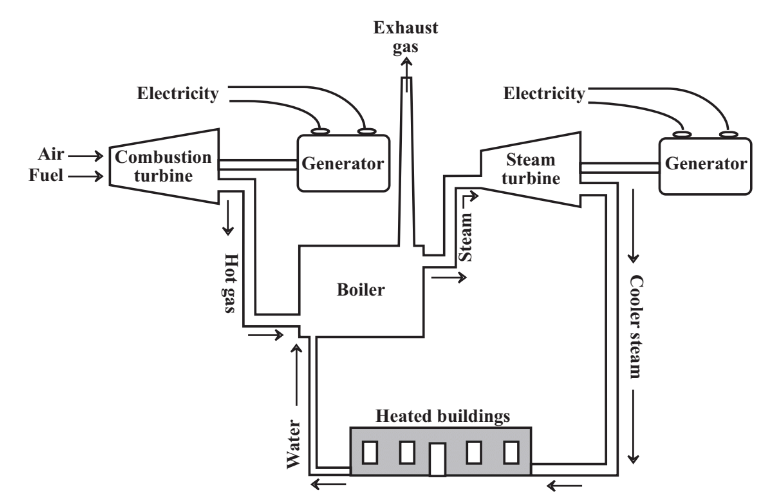
Part of what makes the US and other industrialized countries so dependent on fossil fuels is the relative ease by which they are obtained. One only needs to drill a hole in the ground and transportation can occur through existing pipelines and tankers to refineries that are already in existence. However, energy has been wasted to a shocking extent by the US and some other industrialized nations. This points to the opportunity afforded by energy conservation as the most effective means of providing adequate energy while reducing greenhouse gas emissions. The potential for energy conservation is illustrated by the fact that some nations that have living standards near or even exceeding those of the US have much lower energy consumption per capita.
The economic sector with the greatest potential for increased energy efficiency is transportation. Private automobiles and commercial aircraft are only about one-third as efficient as trains and buses in moving people. Movement of freight by truck requires 5-6 times as much energy as transport by train. Furthermore, electrified railways are much better adapted to using renewable sources of energy than are private automobiles, trucks, and aircraft.
As illustrated in Figure \(\PageIndex{2}\), US automobile fuel economy increased impressively from the first “energy crisis” of the 1970s until about 1990. This was achieved along with much lower emissions of exhaust pollutants. Unfortunately, the trend toward better fuel economy, which if continued would have meant average mileage figures of at least 40 miles per gallon (mpg) by 2010, stopped moving up with increased popularity of oversized vehicles, especially the “sport utility vehicle” type. In 2007 the US Congress passed legislation mandating improved fuel economy for automobiles sold in the US. An average of 40 mpg is readily achievable without significantly compromising safety and comfort and has the added benefit of substantially reduced emissions of the greenhouse gas carbon dioxide.
Hybrid vehicles that are propelled by an electric motor connected to a battery rechargeable with a small internal combustion engine now deliver impressive fuel economy. The improvement is especially pronounced for stop-and-go driving in traffic where at least 50% better mileage figures have been achieved. In operation, the main battery in the vehicle, which is much larger than a conventional automobile battery, but smaller than one required for an all-electric vehicle, is kept charged by the onboard internal combustion engine coupled to a generator. A contribution is also made by regenerative braking that generates electricity during braking. When the vehicle is slowing, coasting downhill, or stopped, the internal combustion engine turns off which saves fuel.
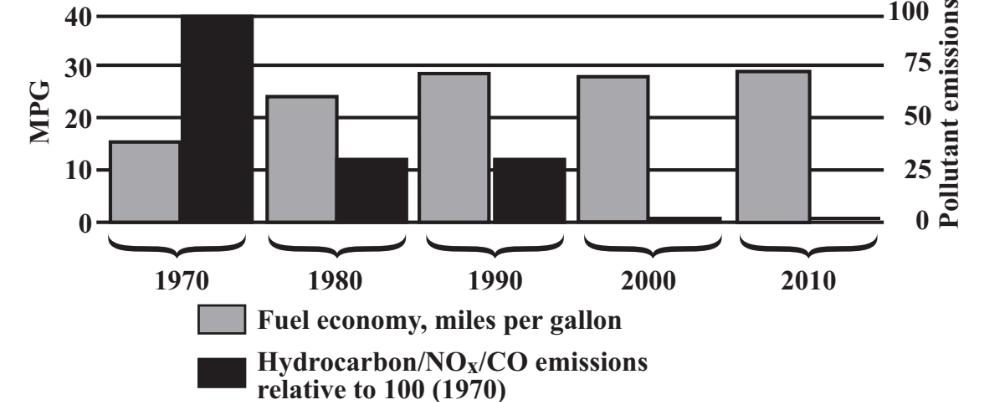
Until recently, the battery of choice for hybrid vehicles has been the nickel-metal-hydride battery. As of 2010, development was actively underway on hybrid vehicles using lithium ion batteries which hold a relatively greater charge per unit mass. These batteries can be charged from an external source of electricity providing 40-50 km of driving range before the onboard internal combustion engine has to engage. Charging the battery from an external source is much less expensive than using an internal combustion engine, affording rather spectacular fuel economy.
One advantage of electrical energy, whether in driving cars or in the home, is that there are multiple ways of generating the electricity. While many electrical power plants in the US still use coal to generate electricity, there are a number of other options. Some areas use nuclear power plants which use nuclear power as a heat source to produce steam used to generate mechanical and then electrical energy. While this source of energy does not release any greenhouse gases, it does generate high-level nuclear wastes which must be dealt with for centuries.
Other energy types--considered to be renewable energy sources--are solar, wind, and water energy. Solar energy can be used simply for heating. It can also be converted directly to electricity using photovoltaic cells. While solar energy obviously cannot be collected at night, the use of photovoltaic cells in combination with storage devices will allow for a continuous supply of electricity. Wind and water energy are among the oldest sources of energy used by mankind, such as wind used to propel sailing ships and waterwheels used for centuries to grind grain. They are also among the newest sources of energy with technologically advanced wind turbines and ingenious devices that capture the energy of moving water in ocean tides.
Wind power is undergoing rapid growth in a number of countries and has become competitive in cost with more conventional sources in some areas. In parts of Europe, California, Wyoming, and other locations, the sight of wind-powered generators mounted on towers has become common (Figure \(\PageIndex{3}\)). World wind power capacity increased by 31% in 2009 alone, reaching a capacity of 158 gigawatts (GW). During 2009, China’s wind power capacity doubled from 12 GW to 25 GW and the US capacity grew by 10 GW to 35 GW total capacity. This gave the US the largest wind power capacity of any nation in the world, though it is rapidly being overtaken by China.
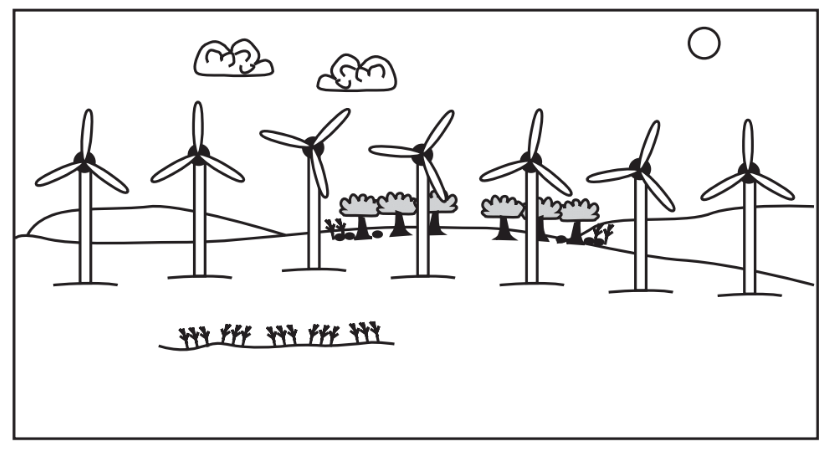
Modern wind turbines are generally large and sophisticated machines with diameters of 40 to 50 meters and rated between 0.5 to 2 megawatts (MW). The largest turbines available as of 2010 were rated at 7 MW. Wind turbines are designed to operate consistently at varying wind speeds, to respond to changing wind direction, and to operate over a wide range of temperatures. Provision is made for electrical resistance heating of turbine blades in cold climates where ice accumulation is likely.
Offshore locations with turbines firmly anchored to the sea bed offer several advantages for production of wind power including generally consistent winds in coastal areas and lack of conflict with uses of land. The largest offshore wind power project to date began operation off the coast of southeast England in September 2010. This facility operated by Vattenfall, a Swedish energy company, has 100 turbines each 115 meters tall, and produces up to 300 megawatts of electricity, enough to power 200,000 homes.
A renaissance in waterpower occurred in the late 1800s when it became practical to drive electrical generators with water. Starting with the first hydroelectric plant in the U.S. on the Fox River near Appleton, Wisconsin, in 1882, hydroelectric power driven by sophisticated power turbines grew rapidly in the U.S. and throughout the world. By 1980 hydroelectric power composed 25% of world electricity production and 5% of total world energy generation. Norway generates essentially all of its electric power and about 50% of its total energy from hydroelectric sources.
The morphology of the geosphere has a strong influence on the potential for hydroelectric power generation, which is favored by mountainous terrain and large river valleys. Abundant rainfall and snowmelt are also important factors. China has about 1/10 of the world’s potential for hydroelectric energy and its enormous Three Gorges installation on the Yangtze River is the world’s largest. The reservoir for this power source has been impounded with a 185-meter high, 1,300-m long dam located at the end of a number of steep canyons holding a body of water that extends for 630 km with an average width of 1.2 km. When fully operational, this massive installation will have 32 generating units and a capacity of 22.5 gigawatts, equivalent to 22 large coal-fired power plants.
With the hydrologic cycle continuously pumping water into it, hydroelectric power is certainly sustainable, and prevents release of greenhouse gases. Bodies of water impounded to provide power can serve as water supplies for agriculture, municipalities, and industries. The potential exists to practice aquaculture in reservoirs by raising fish and freshwater shrimp(prawns). On the negative side, the development of hydroelectric power can present some serious environmental problems. In the modern era, construction of a large power reservoir displaces significant numbers of people (more than 1 million for China’s Three Gorges project), alters river flow, changes aquatic ecology, and fills once scenic valleys with water. In several significant cases dams have been removed from rivers to restore their valleys to their former state.
For those instances where electrical energy is not practical, new technologies are making it possible to use biomass, or the liquid and gaseous fuels made by processing it, for combustion. This is especially promising for transportation. Again, biomass consists of photosynthetically generated materials such as wood, charcoal, forestry residues, agricultural by-products, rapidly growing grasses, livestock manure, and algae. Since these compounds are made by removing carbon dioxide from the atmosphere during photosynthesis, the carbon dioxide generated during their combustion does not lead to a net increase in the levels in the atmosphere. In addition, there tends to be very little sulfur dioxide in biofuels and the ash contains nutrients that can be returned to the soil without adding the heavy metals that is a problem with coal ash. Today, more than 14% of the world's primary energy comes from biofuels, especially wood and charcoal.
Despite concerns that not enough biomass can be grown to produce fuel and that it detracts from food supplies, it should be noted that about 150 billion metric tons of biomass are produced in the world each year by photosynthesis, mostly from uncontrolled plant growth. Corn, the most productive common field crop, produces about 4 metric tons per acre of dry biomass annually (including stalks, leaves, husks, and corncobs). Switchgrass, a prolific producer of biomass, typically generates 11-12 tons of biomass per acre per year (there are 640 acres in a square mile of land). About 6% of the biomass generated globally each year would be equivalent to the world’s demand for fossil fuels. Cultivation for fuel biomass of 6-8% of the land area of the 48 contiguous states would provide energy equivalent to annual U.S. consumption of petroleum and natural gas. Furthermore, only a small fraction of widely grown grain crops goes into grain; the rest is plant biomass, much of which could be used for energy production. And the U.S. has vast areas of underutilized land that could be devoted to the cultivation of energy-yielding plants. Much of this neglected, erosion-prone land would benefit from the cultivation of perennial plants that could be harvested for energy and regrow from roots left in the ground, thus lowering water and wind erosion.
In order for some of these alternative energy sources to become viable options in large-scale generation of energy and electricity, investments do need to be made in technology and infrastructure to support it. One area where governments can help with this is by providing incentives to citizens and companies for investing in renewable energy sources. This is one type of institutionalized mitigation strategy. While the research may be initially expensive, we often develop technologies that have major impacts in other sectors of the economy as well, such as improved batteries that can be used for other types of devices. Thus, it is imperative that initiatives be taken now instead of waiting for others to develop the technology and make the US dependent on them for its energy needs.
Governmental regulations regarding fuel efficiency of vehicles is another example of an institutionalized mitigation strategy already in place in the United States and in many other countries around the world. Unlike some other countries, there are no carbon taxes or charges on burning fossil fuels in the United States. This is another governmental mitigation strategy that has been shown to be effective in many countries including India, Japan, France, Costa Rica, Canada, and the United Kingdom.
Mitigation Strategies - Carbon Capture and Sequestration
In situations where carbon dioxide must be released on a large scale, technology can also be harnessed to mitigate climate change. One strategy for this is the use of carbon capture and sequestration (CCS). Through CCS, 80-90% of the CO2 that would have been emitted to the atmosphere from sources such as a coal-fired power plant is instead captured and then stored deep beneath the Earth’s surface. The CO2 is often injected and sequestered hundreds of miles underground into porous rock formations sealed below an impermeable layer, where it is stored permanently (Figure \(\PageIndex{4}\)).

Another option when burning coal is that the hot carbon from the burning coal can be reacted with oxygen and water to produce elemental hydrogen and carbon dioxide. The net reaction for this production is the following:
\[2\;{\mathrm C}_{(s)}\;+\:{\mathrm O}_{2(g)}\:+\:2\;{\mathrm H}_2{\mathrm O}_{(g)}\rightarrow\;2\;{\mathrm{CO}}_{2(g)}\;+\:2{\mathrm H}_{2(g)}\]
The hydrogen generated can be used as a pollution-free fuel in fuel cells or combustion engines. The carbon dioxide can be pumped deep underground. A side benefit of this approach is that in some areas carbon dioxide pumped underground can be used to recover additional crude oil from depleted oil-bearing formations.
Scientists are also looking into the use of soils and vegetation for carbon storage potential. Proper management of soil and forest ecosystems has been shown to create additional carbon sinks for atmospheric carbon, reducing the overall atmospheric CO2 burden. Increasing soil carbon further benefits communities by providing better-quality soil for agriculture and cultivation.
Green chemistry can also be applied in the prevention of release of greenhouse gases other than carbon dioxide, especially ultrastable volatile compounds that have a high greenhouse gas potential. An excellent example of green chemistry has been the replacement of chlorofluorocarbons (Freons) with analogous compounds having at least one C-H bond, that are rather readily destroyed in the troposphere. Although this was done to prevent destruction of stratospheric ozone by chlorofluorocarbons, it has also been useful to reduce greenhouse warming. Both kinds of compounds act as greenhouse gases, but those with at least one C-H bond last for much shorter times during which they are available to absorb infrared radiation.
Another approach is to limit the emissions of methane, CH4. Large quantities of methane are released by anoxic bacteria growing in flooded rice paddies. By developing strains of rice and means of cultivation that enable the crop to be grown on unflooded soil, this source of methane can be greatly reduced. Methane collection systems placed in municipal waste landfills can prevent the release of methane from this source and provide a source of methane fuel.
Adaptation, Mitigation and You
While we may look to institutions to solve global warming and the resultant climate change problems, we each have choices that we make every day that can influence greenhouse gas emissions or help with adaptation. When possible, use energy efficient appliances, lighting, heating and cooling equipment, and other products. Heat and cool your home smartly to reduce your energy needs. If financially feasible, consider rooftop solar or other self-supplied green power. Encourage your employer to do the same for larger impact.
Transportation is another area where we have an impact on the environment. Look for ways to use greener transportation such as biking, walking, carpooling, and public transportation. If you do need to drive your own vehicle, choose energy efficient vehicles, ideally hybrid or electric with energy-saving electric vehicle chargers. In addition, drive smarter. Improve your fuel economy by going easy on the brakes and gas, using cruise control, and keeping your vehicle well-maintained. Limiting the number of trips that you need to take by grouping errands and teleworking, if available, can also reduce fuel use and greenhouse gas emissions.
While the area in which you live may not be experiencing water shortages, it does take a lot of energy to pump, treat, and heat water. Thus, conserving water reduces greenhouse emissions as well. Again, look for energy efficient clothes washers and dishwashers. Check for water leaks in your home and workplace and turn off running faucets. Install a low-flow showerhead and take shorter showers. If you have a yard, develop a water-smart landscape suitable to your climate. Choose drought-resistant plants and be cognizant of when and how much to water.
As mentioned, waste contributes to greenhouse gases through the methane gas produced during the breakdown of wastes in landfills. Thus, the most effective to reduce waste emissions is to not create it in the first place by practicing the three R's: reduce, reuse, and recycle. In addition, by buying used items you also reduce the emissions created by producing the new materials.
While these may seem like small things, they do add up, especially if adopted by large community populations. Take a moment to identify ways that you personally can be involved in the mitigation of or adaptation to climate change. What changes can you make in your own life to prevent excess carbon emissions? You can calculate your carbon footprint using the EPA’s carbon footprint calculator.
Summary
- Adaptation strategies involve changing behaviors based on changes to the environment which have already occurred.
- Most mitigation strategies are efforts to minimize the amount of carbon dioxide which is released into the atmosphere. The best way to accomplish this is through improved energy efficiency and through alternative energy sources such as wind, solar, water, and biomass.
- In addition to reducing the amount of carbon dioxide generated, the gas can be sequestered by pumping it deep into the Earth or by properly managing forests and soil to provide carbon sinks.
- Green chemistry can be utilized to develop alternative compounds which do not have as detrimental of an effect on the atmosphere as ones used in the past.
- Everyone can do their part to reduce greenhouse gas emissions in the choices that we make every day.
Contributors and Attributions
- Libretext: Introduction to Environmental Science (Zendher et al.)
- US EPA
- National Oceanic and Atmospheric Administration
- Stanley E. Manahan (University of Missouri)
- Vicki MacMurdo (Anoka-Ramsey Community College)