12.1: Gases and Pressure
- Page ID
- 435126
\( \newcommand{\vecs}[1]{\overset { \scriptstyle \rightharpoonup} {\mathbf{#1}} } \)
\( \newcommand{\vecd}[1]{\overset{-\!-\!\rightharpoonup}{\vphantom{a}\smash {#1}}} \)
\( \newcommand{\id}{\mathrm{id}}\) \( \newcommand{\Span}{\mathrm{span}}\)
( \newcommand{\kernel}{\mathrm{null}\,}\) \( \newcommand{\range}{\mathrm{range}\,}\)
\( \newcommand{\RealPart}{\mathrm{Re}}\) \( \newcommand{\ImaginaryPart}{\mathrm{Im}}\)
\( \newcommand{\Argument}{\mathrm{Arg}}\) \( \newcommand{\norm}[1]{\| #1 \|}\)
\( \newcommand{\inner}[2]{\langle #1, #2 \rangle}\)
\( \newcommand{\Span}{\mathrm{span}}\)
\( \newcommand{\id}{\mathrm{id}}\)
\( \newcommand{\Span}{\mathrm{span}}\)
\( \newcommand{\kernel}{\mathrm{null}\,}\)
\( \newcommand{\range}{\mathrm{range}\,}\)
\( \newcommand{\RealPart}{\mathrm{Re}}\)
\( \newcommand{\ImaginaryPart}{\mathrm{Im}}\)
\( \newcommand{\Argument}{\mathrm{Arg}}\)
\( \newcommand{\norm}[1]{\| #1 \|}\)
\( \newcommand{\inner}[2]{\langle #1, #2 \rangle}\)
\( \newcommand{\Span}{\mathrm{span}}\) \( \newcommand{\AA}{\unicode[.8,0]{x212B}}\)
\( \newcommand{\vectorA}[1]{\vec{#1}} % arrow\)
\( \newcommand{\vectorAt}[1]{\vec{\text{#1}}} % arrow\)
\( \newcommand{\vectorB}[1]{\overset { \scriptstyle \rightharpoonup} {\mathbf{#1}} } \)
\( \newcommand{\vectorC}[1]{\textbf{#1}} \)
\( \newcommand{\vectorD}[1]{\overrightarrow{#1}} \)
\( \newcommand{\vectorDt}[1]{\overrightarrow{\text{#1}}} \)
\( \newcommand{\vectE}[1]{\overset{-\!-\!\rightharpoonup}{\vphantom{a}\smash{\mathbf {#1}}}} \)
\( \newcommand{\vecs}[1]{\overset { \scriptstyle \rightharpoonup} {\mathbf{#1}} } \)
\( \newcommand{\vecd}[1]{\overset{-\!-\!\rightharpoonup}{\vphantom{a}\smash {#1}}} \)
\(\newcommand{\avec}{\mathbf a}\) \(\newcommand{\bvec}{\mathbf b}\) \(\newcommand{\cvec}{\mathbf c}\) \(\newcommand{\dvec}{\mathbf d}\) \(\newcommand{\dtil}{\widetilde{\mathbf d}}\) \(\newcommand{\evec}{\mathbf e}\) \(\newcommand{\fvec}{\mathbf f}\) \(\newcommand{\nvec}{\mathbf n}\) \(\newcommand{\pvec}{\mathbf p}\) \(\newcommand{\qvec}{\mathbf q}\) \(\newcommand{\svec}{\mathbf s}\) \(\newcommand{\tvec}{\mathbf t}\) \(\newcommand{\uvec}{\mathbf u}\) \(\newcommand{\vvec}{\mathbf v}\) \(\newcommand{\wvec}{\mathbf w}\) \(\newcommand{\xvec}{\mathbf x}\) \(\newcommand{\yvec}{\mathbf y}\) \(\newcommand{\zvec}{\mathbf z}\) \(\newcommand{\rvec}{\mathbf r}\) \(\newcommand{\mvec}{\mathbf m}\) \(\newcommand{\zerovec}{\mathbf 0}\) \(\newcommand{\onevec}{\mathbf 1}\) \(\newcommand{\real}{\mathbb R}\) \(\newcommand{\twovec}[2]{\left[\begin{array}{r}#1 \\ #2 \end{array}\right]}\) \(\newcommand{\ctwovec}[2]{\left[\begin{array}{c}#1 \\ #2 \end{array}\right]}\) \(\newcommand{\threevec}[3]{\left[\begin{array}{r}#1 \\ #2 \\ #3 \end{array}\right]}\) \(\newcommand{\cthreevec}[3]{\left[\begin{array}{c}#1 \\ #2 \\ #3 \end{array}\right]}\) \(\newcommand{\fourvec}[4]{\left[\begin{array}{r}#1 \\ #2 \\ #3 \\ #4 \end{array}\right]}\) \(\newcommand{\cfourvec}[4]{\left[\begin{array}{c}#1 \\ #2 \\ #3 \\ #4 \end{array}\right]}\) \(\newcommand{\fivevec}[5]{\left[\begin{array}{r}#1 \\ #2 \\ #3 \\ #4 \\ #5 \\ \end{array}\right]}\) \(\newcommand{\cfivevec}[5]{\left[\begin{array}{c}#1 \\ #2 \\ #3 \\ #4 \\ #5 \\ \end{array}\right]}\) \(\newcommand{\mattwo}[4]{\left[\begin{array}{rr}#1 \amp #2 \\ #3 \amp #4 \\ \end{array}\right]}\) \(\newcommand{\laspan}[1]{\text{Span}\{#1\}}\) \(\newcommand{\bcal}{\cal B}\) \(\newcommand{\ccal}{\cal C}\) \(\newcommand{\scal}{\cal S}\) \(\newcommand{\wcal}{\cal W}\) \(\newcommand{\ecal}{\cal E}\) \(\newcommand{\coords}[2]{\left\{#1\right\}_{#2}}\) \(\newcommand{\gray}[1]{\color{gray}{#1}}\) \(\newcommand{\lgray}[1]{\color{lightgray}{#1}}\) \(\newcommand{\rank}{\operatorname{rank}}\) \(\newcommand{\row}{\text{Row}}\) \(\newcommand{\col}{\text{Col}}\) \(\renewcommand{\row}{\text{Row}}\) \(\newcommand{\nul}{\text{Nul}}\) \(\newcommand{\var}{\text{Var}}\) \(\newcommand{\corr}{\text{corr}}\) \(\newcommand{\len}[1]{\left|#1\right|}\) \(\newcommand{\bbar}{\overline{\bvec}}\) \(\newcommand{\bhat}{\widehat{\bvec}}\) \(\newcommand{\bperp}{\bvec^\perp}\) \(\newcommand{\xhat}{\widehat{\xvec}}\) \(\newcommand{\vhat}{\widehat{\vvec}}\) \(\newcommand{\uhat}{\widehat{\uvec}}\) \(\newcommand{\what}{\widehat{\wvec}}\) \(\newcommand{\Sighat}{\widehat{\Sigma}}\) \(\newcommand{\lt}{<}\) \(\newcommand{\gt}{>}\) \(\newcommand{\amp}{&}\) \(\definecolor{fillinmathshade}{gray}{0.9}\)- Understand properties of gases, gas pressure and atmospheric pressure.
- List common pressure units, understand the workings of a barometer.
- Describe the relationship between atmospheric pressure and altitude.
- Understand systolic and diastolic blood pressures.
- Know the elements that are monoatomic and diatomic gases.
- Recognize STP conditions.
The gas phase is unique among the three states of matter. There are some simple models we can use to predict the physical behavior of all gases—independent of their identities. We cannot do this for the solid and liquid states. In fact, the development of this understanding of the behavior of gases represents the historical dividing point between alchemy and modern chemistry. Initial advances in the understanding of gas behavior were made in the mid 1600s by Robert Boyle, an English scientist who founded the Royal Society (one of the world’s oldest scientific organizations).
How is it that we can model all gases independent of their chemical identity? The answer is in a group of statements called the kinetic theory of gases:
- Gases are composed of tiny particles that are separated by large distances.
- Gas particles are constantly moving, experiencing collisions with other gas particles and the walls of their container.
- The kinetic energy of gas particles is related to the temperature of a gas.
- Gas particles do not experience any force of attraction or repulsion with each other.
Did you notice that none of these statements relates to the identity of the gas? This means that all gases should behave similarly. A gas that follows these statements perfectly is called an ideal gas. Most gases show slight deviations from these statements and are called real gases. The kinetic theory also states that there is no interaction between individual gas particles. There are, in fact, intermolecular interactions in real gases, however the kinetic theory assumes that gas particles are so far apart that the individual particles don’t “feel” each other.
Gas Pressure
One of the statements of the kinetic theory mentions collisions. As gas particles are constantly moving, they are also constantly colliding with each other and with the walls of their container. There are forces involved as gas particles bounce off the container walls (Figure \(\PageIndex{1}\)). The force generated by gas particles divided by the area of the container walls yields pressure.
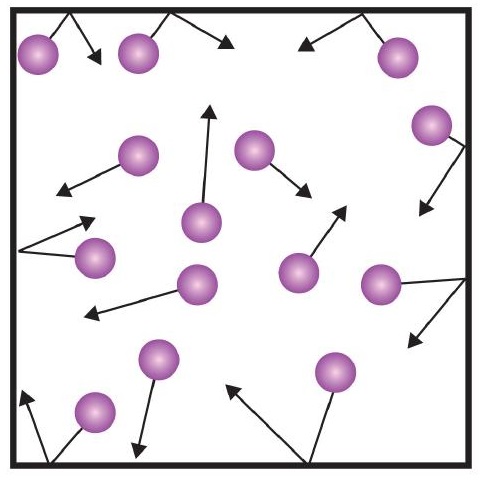
Atmospheric Pressure
Just as we exert pressure on a surface because of gravity, so does our atmosphere. We live at the bottom of an ocean of gases that becomes progressively less dense with increasing altitude. Approximately 99% of the mass of the atmosphere lies within 30 km of Earth’s surface, and half of it is within the first 5.5 km. Every point on Earth’s surface experiences a net pressure called atmospheric pressure.
Barometer
Atmospheric pressure can be measured using a barometer. This device invented in 1643 by one of Galileo’s students, Evangelista Torricelli (1608–1647). A barometer may be constructed from a long glass tube that is closed at one end. It is filled with mercury and placed upside down in a dish of mercury without allowing any air to enter the tube. Some of the mercury will run out of the tube, but a relatively tall column remains inside (Figure \(\PageIndex{2}\)) . Why doesn’t all the mercury run out? Gravity is exerting a downward force on the mercury in the tube, but it is opposed by the pressure of the atmosphere pushing down on the surface of the mercury in the dish, which has the net effect of pushing the mercury up into the tube. Because there is no air above the mercury inside the tube in a properly filled barometer (it contains a vacuum), there is no pressure pushing down on the column. Thus the mercury runs out of the tube until the pressure exerted by the mercury column itself exactly balances the pressure of the atmosphere. Under normal weather conditions at sea level, the two forces are balanced when the top of the mercury column is approximately 760 mm above the level of the mercury in the dish, as shown in (Figure \(\PageIndex{2}\)) . This value varies with meteorological conditions and altitude. In Denver, Colorado, for example, at an elevation of about 1 mile, or 1609 m (5280 ft), the height of the mercury column is 630 mm rather than 760 mm.
Figure \(\PageIndex{2}\): The pressure exerted by the atmosphere on the surface of the pool of mercury supports a column of mercury in the tube that is about 760 mm tall.
While mercury barometers were the workhorses for pressure measurement into the last quarter of the 20th century, they have been replaced by electronic gauges. One motivation was safety. Mercury and mercury vapor are heavy metal poisons.
Units of Atmospheric Pressure
Common units of pressure are the atmosphere (atm), which was originally defined as the average pressure of Earth’s atmosphere at sea level; and mmHg (millimeters of mercury), which is the pressure generated by a column of mercury 1 mm high. The unit millimeters of mercury is also called a torr, named after the Italian scientist Evangelista Torricelli, who invented the barometer in the mid-1600s. A more precise definition of atmosphere, in terms of torr, is that there are exactly 760 torr in 1 atm.
1 atm = 14.7 psi = 760 torr = 760 mm of Hg
Given all the relationships between these pressure units, the ability to convert from one pressure unit to another is a useful skill.
Write a conversion factor to determine how many atmospheres are in 1,547 mm of Hg.
Solution
Because there are 760.0 mm of Hg in 1 atm, we can use this conversion factor to do the mathematical conversion:
\(\mathrm{1,547\: mmHg\times \dfrac{1\: atm}{760.0\: mmHg}=2.036\: atm}\)
Note how the mm of Hg units cancel algebraically.
Write a conversion factor to determine how many millimeters of mercury are in 9.65 atm.
- Answer
-
\(\mathrm{9.65\: atm\times \dfrac{760\: mm Hg}{1\: atm}=7,330 \: mm Hg}\).
Atmospheric Pressure and Altitude
As altitude increases, the air becomes thinner, the atmospheric pressure decreases (Figure \(\PageIndex{3}\)) . The oxygen levels in the air decreases as the altitude increases. That is why mountain climbers experience an inadequate supply of oxygen to the body at high altitudes -a medical condition called Acute Mountain Sickness.
Figure \(\PageIndex{3}\): Air pressure decreases and the air thins and oxygen levels in it decreases as the altitude increases. Source: Brendan Scott/The Conversation, CC BY-ND https://images.theconversation.com/f....png?ixlib=rb-
Blood pressure
The heart pumps the blood into the circulatory system. When the heart contracts, it applies the pressure on the blood in it, and the blood pumps out of the heart into the circulatory system, as illustrated in (Figure \(\PageIndex{4}\)). The blood pressure in the circulatory system is highest at this point, and it is called systolic pressure. It can be in the range of 100 to 200 mm Hg. A desirable systolic pressure range is 100 to 120 mm Hg. When heart muscles relax, the cavity in the heart expands and more blood fills in the heart. The blood pressure in the circulatory system is minimum at this point, and it is called diastolic pressure. The diastolic pressure may vary in the range of 60 to 110 mm Hg. A desirable diastolic pressure is less than 80 mm Hg.

The device used to measure blood pressure is called a sphygmometer or sphygmomanometer. It consists of a cuff that wraps around the upper arm, a pump to inflate air in the cuff, and a stethoscope to hear the sound of blood flow, as illustrated in (Figure \(\PageIndex{5}\)) . The cuff is inflated with air to a pressure above the systolic pressure and it results in cutting off the blood flow through the brachial artery in the upper arm. A stethoscope is used to hear the sound of blood flow. No sound is heard at this point. The pressure in the cuff is slowly reduced. When the pressure in the cuff is equal to systolic pressure, the blood begins to spurt into the artery, and a tapping sound is heard through the stethoscope. At the point when the pressure is equal to the diastolic pressure, the blood flows freely through the artery, and the tapping sound disappears. The blood pressure reading is reported as a set of two numbers, e.g., 120/80, where the higher number is systolic, and the lower number is the diastolic pressure.
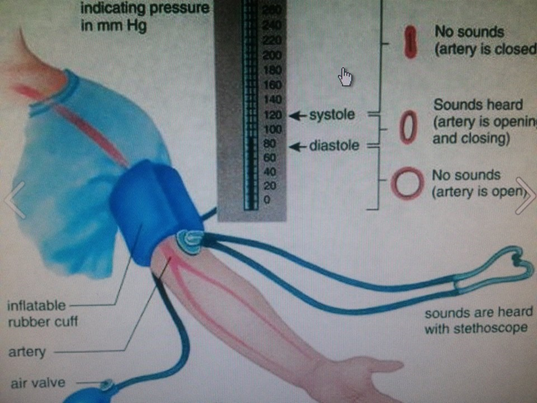
Monoatomic and Diatomic gases
Figure 12.1.6 shows the locations in the periodic table of elements that are commonly found in the gaseous, liquid, and solid states. Except for hydrogen, the elements that occur naturally as gases are on the right side of the periodic table. Of these, all the noble gases He, Ne, Ar, Kr, Xe, and Rn are monatomic gases, whereas the other gaseous elements are diatomic molecules (H2, N2, O2, F2, and Cl2). Bromine (Br2) and mercury (Hg) are liquids under normal conditions (25°C and 1.0 atm, commonly referred to as “room temperature and pressure”). The rest of the elements are all solids under normal conditions.
Figure \(\PageIndex{6}\): Elements That Occur Naturally as Gases, Liquids, and Solids at 25°C and 1 atm
Standard Temperature and Pressure (STP)
A temperature of 0.0oC (273.15 Kelvin) and under 1 atmosphere of pressure (760 mm of Hg) is referred to as STP.
Key Takeaway
The gas phase has certain general properties characteristic of that phase. The atmosphere applies a pressure on the surface of the earth known as the atmospheric pressure. The barometer is used to measure the atmospheric pressure. The atmospheric pressure changes with altitude. Blood pressure is the pressure applied by the blood on the walls of the arteries and is measured by a device called the sphygmometer.