Overview of Acids and Bases
- Page ID
- 78247
\( \newcommand{\vecs}[1]{\overset { \scriptstyle \rightharpoonup} {\mathbf{#1}} } \)
\( \newcommand{\vecd}[1]{\overset{-\!-\!\rightharpoonup}{\vphantom{a}\smash {#1}}} \)
\( \newcommand{\id}{\mathrm{id}}\) \( \newcommand{\Span}{\mathrm{span}}\)
( \newcommand{\kernel}{\mathrm{null}\,}\) \( \newcommand{\range}{\mathrm{range}\,}\)
\( \newcommand{\RealPart}{\mathrm{Re}}\) \( \newcommand{\ImaginaryPart}{\mathrm{Im}}\)
\( \newcommand{\Argument}{\mathrm{Arg}}\) \( \newcommand{\norm}[1]{\| #1 \|}\)
\( \newcommand{\inner}[2]{\langle #1, #2 \rangle}\)
\( \newcommand{\Span}{\mathrm{span}}\)
\( \newcommand{\id}{\mathrm{id}}\)
\( \newcommand{\Span}{\mathrm{span}}\)
\( \newcommand{\kernel}{\mathrm{null}\,}\)
\( \newcommand{\range}{\mathrm{range}\,}\)
\( \newcommand{\RealPart}{\mathrm{Re}}\)
\( \newcommand{\ImaginaryPart}{\mathrm{Im}}\)
\( \newcommand{\Argument}{\mathrm{Arg}}\)
\( \newcommand{\norm}[1]{\| #1 \|}\)
\( \newcommand{\inner}[2]{\langle #1, #2 \rangle}\)
\( \newcommand{\Span}{\mathrm{span}}\) \( \newcommand{\AA}{\unicode[.8,0]{x212B}}\)
\( \newcommand{\vectorA}[1]{\vec{#1}} % arrow\)
\( \newcommand{\vectorAt}[1]{\vec{\text{#1}}} % arrow\)
\( \newcommand{\vectorB}[1]{\overset { \scriptstyle \rightharpoonup} {\mathbf{#1}} } \)
\( \newcommand{\vectorC}[1]{\textbf{#1}} \)
\( \newcommand{\vectorD}[1]{\overrightarrow{#1}} \)
\( \newcommand{\vectorDt}[1]{\overrightarrow{\text{#1}}} \)
\( \newcommand{\vectE}[1]{\overset{-\!-\!\rightharpoonup}{\vphantom{a}\smash{\mathbf {#1}}}} \)
\( \newcommand{\vecs}[1]{\overset { \scriptstyle \rightharpoonup} {\mathbf{#1}} } \)
\( \newcommand{\vecd}[1]{\overset{-\!-\!\rightharpoonup}{\vphantom{a}\smash {#1}}} \)
\(\newcommand{\avec}{\mathbf a}\) \(\newcommand{\bvec}{\mathbf b}\) \(\newcommand{\cvec}{\mathbf c}\) \(\newcommand{\dvec}{\mathbf d}\) \(\newcommand{\dtil}{\widetilde{\mathbf d}}\) \(\newcommand{\evec}{\mathbf e}\) \(\newcommand{\fvec}{\mathbf f}\) \(\newcommand{\nvec}{\mathbf n}\) \(\newcommand{\pvec}{\mathbf p}\) \(\newcommand{\qvec}{\mathbf q}\) \(\newcommand{\svec}{\mathbf s}\) \(\newcommand{\tvec}{\mathbf t}\) \(\newcommand{\uvec}{\mathbf u}\) \(\newcommand{\vvec}{\mathbf v}\) \(\newcommand{\wvec}{\mathbf w}\) \(\newcommand{\xvec}{\mathbf x}\) \(\newcommand{\yvec}{\mathbf y}\) \(\newcommand{\zvec}{\mathbf z}\) \(\newcommand{\rvec}{\mathbf r}\) \(\newcommand{\mvec}{\mathbf m}\) \(\newcommand{\zerovec}{\mathbf 0}\) \(\newcommand{\onevec}{\mathbf 1}\) \(\newcommand{\real}{\mathbb R}\) \(\newcommand{\twovec}[2]{\left[\begin{array}{r}#1 \\ #2 \end{array}\right]}\) \(\newcommand{\ctwovec}[2]{\left[\begin{array}{c}#1 \\ #2 \end{array}\right]}\) \(\newcommand{\threevec}[3]{\left[\begin{array}{r}#1 \\ #2 \\ #3 \end{array}\right]}\) \(\newcommand{\cthreevec}[3]{\left[\begin{array}{c}#1 \\ #2 \\ #3 \end{array}\right]}\) \(\newcommand{\fourvec}[4]{\left[\begin{array}{r}#1 \\ #2 \\ #3 \\ #4 \end{array}\right]}\) \(\newcommand{\cfourvec}[4]{\left[\begin{array}{c}#1 \\ #2 \\ #3 \\ #4 \end{array}\right]}\) \(\newcommand{\fivevec}[5]{\left[\begin{array}{r}#1 \\ #2 \\ #3 \\ #4 \\ #5 \\ \end{array}\right]}\) \(\newcommand{\cfivevec}[5]{\left[\begin{array}{c}#1 \\ #2 \\ #3 \\ #4 \\ #5 \\ \end{array}\right]}\) \(\newcommand{\mattwo}[4]{\left[\begin{array}{rr}#1 \amp #2 \\ #3 \amp #4 \\ \end{array}\right]}\) \(\newcommand{\laspan}[1]{\text{Span}\{#1\}}\) \(\newcommand{\bcal}{\cal B}\) \(\newcommand{\ccal}{\cal C}\) \(\newcommand{\scal}{\cal S}\) \(\newcommand{\wcal}{\cal W}\) \(\newcommand{\ecal}{\cal E}\) \(\newcommand{\coords}[2]{\left\{#1\right\}_{#2}}\) \(\newcommand{\gray}[1]{\color{gray}{#1}}\) \(\newcommand{\lgray}[1]{\color{lightgray}{#1}}\) \(\newcommand{\rank}{\operatorname{rank}}\) \(\newcommand{\row}{\text{Row}}\) \(\newcommand{\col}{\text{Col}}\) \(\renewcommand{\row}{\text{Row}}\) \(\newcommand{\nul}{\text{Nul}}\) \(\newcommand{\var}{\text{Var}}\) \(\newcommand{\corr}{\text{corr}}\) \(\newcommand{\len}[1]{\left|#1\right|}\) \(\newcommand{\bbar}{\overline{\bvec}}\) \(\newcommand{\bhat}{\widehat{\bvec}}\) \(\newcommand{\bperp}{\bvec^\perp}\) \(\newcommand{\xhat}{\widehat{\xvec}}\) \(\newcommand{\vhat}{\widehat{\vvec}}\) \(\newcommand{\uhat}{\widehat{\uvec}}\) \(\newcommand{\what}{\widehat{\wvec}}\) \(\newcommand{\Sighat}{\widehat{\Sigma}}\) \(\newcommand{\lt}{<}\) \(\newcommand{\gt}{>}\) \(\newcommand{\amp}{&}\) \(\definecolor{fillinmathshade}{gray}{0.9}\)There are three major classifications of substances known as acids or bases. The Arrhenius definition states that an acid produces H+ in solution and a base produces OH-. This theory was developed by Svante Arrhenius in 1883. Later, two more sophisticated and general theories were proposed. These are the Brønsted-Lowry and the Lewis definitions of acids and bases. The Lewis theory is discussed elsewhere.
The Arrhenius Theory of Acids and Bases
In 1884, the Swedish chemist Svante Arrhenius proposed two specific classifications of compounds; acids and bases. When dissolved in an aqueous solution, certain ions were released into the solution. An Arrhenius acid is a compound that increases the concentration of H+ ions that are present when added to water. These H+ ions form the hydronium ion (H3O+) when they combine with water molecules. This process is represented in a chemical equation by adding H2O to the reactants side.
\[ HCl_{(aq)} \rightarrow H^+_{(aq)} + Cl^-_{(aq)} \]
In this reaction, hydrochloric acid (\(HCl\)) dissociates completely into hydrogen (H+) and chlorine (Cl-) ions when dissolved in water, thereby releasing H+ ions into solution. Formation of the hydronium ion equation:
\[ HCl_{(aq)} + H_2O_{(l)} \rightarrow H_3O^+_{(aq)} + Cl^-_{(aq)} \]
The Arrhenius theory, which is the simplest and least general description of acids and bases, includes acids such as HClO4 and HBr and bases such as \(NaOH\) or \(Mg(OH)_2\). For example the complete dissociation of \(HBr\) gas into water results generates free \(H_3O^+\) ions.
\[HBr_{(g)} + H_2O_{(l)} \rightarrow H_3O^+_{(aq)} + Br^-_{(aq)}\]
This theory successfully describes how acids and bases react with each other to make water and salts. However, it does not explain why some substances that do not contain hydroxide ions, such as \(F^-\) and \(NO_2^-\), can make basic solutions in water. The Brønsted-Lowry definition of acids and bases addresses this problem.
An Arrhenius base is a compound that increases the concentration of OH- ions that are present when added to water. The dissociation is represented by the following equation:
\[ NaOH \; (aq) \rightarrow Na^+ \; (aq) + OH^- \; (aq) \]
In this reaction, sodium hydroxide (NaOH) disassociates into sodium (Na+) and hydroxide (OH-) ions when dissolved in water, thereby releasing OH- ions into solution.
- Arrhenius acids are substances which produce hydrogen ions in solution.
- Arrhenius bases are substances which produce hydroxide ions in solution.
Free Hydrogen Ions do not Exist in Water
Owing to the overwhelming excess of \(H_2O\) molecules in aqueous solutions, a bare hydrogen ion has no chance of surviving in water. The hydrogen ion in aqueous solution is no more than a proton, a bare nucleus. Although it carries only a single unit of positive charge, this charge is concentrated into a volume of space that is only about a hundred-millionth as large as the volume occupied by the smallest atom. (Think of a pebble sitting in the middle of a sports stadium!) The resulting extraordinarily high charge density of the proton strongly attracts it to any part of a nearby atom or molecule in which there is an excess of negative charge. In the case of water, this will be the lone pair (unshared) electrons of the oxygen atom; the tiny proton will be buried within the lone pair and will form a shared-electron (coordinate) bond with it, creating a hydronium ion, \(H_3O^+\). In a sense, \(H_2O\) is acting as a base here, and the product \(H_3O^+\) is the conjugate acid of water:
Although other kinds of dissolved ions have water molecules bound to them more or less tightly, the interaction between H+ and \(H_2O\) is so strong that writing “H+(aq)” hardly does it justice, although it is formally correct. The formula \(H_3O^+\) more adequately conveys the sense that it is both a molecule in its own right, and is also the conjugate acid of water.
The equation "HA → H+ + A–" is so much easier to write that chemists still use it to represent acid-base reactions in contexts in which the proton donor-acceptor mechanism does not need to be emphasized. Thus, it is permissible to talk about “hydrogen ions” and use the formula H+ in writing chemical equations as long as you remember that they are not to be taken literally in the context of aqueous solutions.
Limitations to the Arrhenius Theory
The Arrhenius theory has many more limitations than the other two theories. The theory suggests that in order for a substance to release either H+ or OH- ions, it must contain that particular ion. However, this does not explain the weak base ammonia (NH3) which, in the presence of water, releases hydroxide ions into solution, but does not contain OH- itself.
Hydrochloric acid is neutralized by both sodium hydroxide solution and ammonia solution. In both cases, you get a colourless solution which you can crystallize to get a white salt - either sodium chloride or ammonium chloride. These are clearly very similar reactions. The full equations are:
\[ NaOH \; (aq) + HCl \; (aq) \rightarrow NaCl \; (aq) + H_2O \; (l) \]
\[ NH_3 \; (aq) + HCl \; (aq) \rightarrow NH_4Cl \; (aq) \]
In the sodium hydroxide case, hydrogen ions from the acid are reacting with hydroxide ions from the sodium hydroxide - in line with the Arrhenius theory. However, in the ammonia case, there are no hydroxide ions!
You can get around this by saying that, when the ammonia reacts with the water, it is dissolved in to produce ammonium ions and hydroxide ions:
\[ NH_3 \; (aq) + H_2O \; (l) \rightleftharpoons NH_4^+ \; (aq) + OH^- \;(aq) \]
This is a reversible reaction, and in a typical dilute ammonia solution, about 99% of the ammonia remains as ammonia molecules. Nevertheless, there are hydroxide ions there, and we can squeeze this into the Arrhenius theory. However, this same reaction also happens between ammonia gas and hydrogen chloride gas.
\[ NH_3 \; (g) + HCl \; (g) \rightarrow NH_4Cl \;(s) \]
In this case, there are not any hydrogen ions or hydroxide ions in solution - because there isn't any solution. The Arrhenius theory wouldn't count this as an acid-base reaction, despite the fact that it is producing the same product as when the two substances were in solution. Because of this shortcoming, later theories sought to better explain the behavior of acids and bases in a new manner.
The Brønsted-Lowry Definition
In 1923, chemists Johannes Nicolaus Brønsted and Thomas Martin Lowry independently developed definitions of acids and bases based on the compounds' abilties to either donate or accept protons (H+ ions). In this theory, acids are defined as proton donors; whereas bases are defined as proton acceptors. A compound that acts as both a Brønsted-Lowry acid and base together is called amphoteric.This took the Arrhenius definition one step further, as a substance no longer needed to be composed of hydrogen (H+) or hydroxide (OH-) ions in order to be classified as an acid or base. Consider the following chemical equation:
Here, hydrochloric acid (HCl) "donates" a proton (H+) to ammonia (NH3) which "accepts" it , forming a positively charged ammonium ion (NH4+) and a negatively charged chloride ion (Cl-). Therefore, HCl is a Brønsted-Lowry acid (donates a proton) while the ammonia is a Brønsted-Lowry base (accepts a proton). Also, Cl- is called the conjugate base of the acid HCl and NH4+ is called the conjugate acid of the base NH3.
The Brønsted-Lowry Theory of Acids and Bases
- A Brønsted-Lowry acid is a proton (hydrogen ion) donor.
- A Brønsted-Lowry base is a proton (hydrogen ion) acceptor.
In this theory, an acid is a substance that can release a proton (like in the Arrhenius theory) and a base is a substance that can accept a proton. A basic salt, such as Na+F-, generates OH- ions in water by taking protons from water itself (to make HF):
\[F^-_{(aq)} + H_2O_{(l)} \rightleftharpoons HF_{(aq)} + OH^-\]
When a Brønsted acid dissociates, it increases the concentration of hydrogen ions in the solution, \([H^+]\); conversely, Brønsted bases dissociate by taking a proton from the solvent (water) to generate \([OH^-]\).
- Acid dissociation
\[HA_{(aq)} \rightleftharpoons A^-_{(aq)} + H^+_{(aq)}\]
- Acid Ionization Constant:
\[K_a=\dfrac{[A^-][H^+]}{[HA]}\]
- Base dissociation:
\[B_{(aq)} + H_2O_{(l)} \rightleftharpoons HB^+_{(aq)} + OH^-_{(aq)}\]
- Base Ionization Constant
\[K_b = \dfrac{[HB^+][OH^-]}{[B]}\]
Conjugate Acids and Bases
One important consequence of these equilibria is that every acid (\(HA\)) has a conjugate base (\(A^-\)), and vice-versa. In the base, dissociation equilibrium above the conjugate acid of base \(B\) is \(HB^+\). For a given acid or base, these equilibria are linked by the water dissociation equilibrium:
\[H_2O_{(l)} \rightleftharpoons H^+_{(aq)} + OH^-_{(aq)}\]
with
\[K_w = [H^+][OH^-]\]
for which the equilibrium constant Kw is 1.00 x 10-14 at 25°C. It can be easily shown that the product of the acid and base dissociation constants Ka and Kb is Kw.
Strong and Weak Acids and Bases
Strong acids are molecular compounds that essentially ionize to completion in aqueous solution, disassociating into H+ ions and the additional anion; there are very few common strong acids. All other acids are "weak acids" that incompletely ionized in aqueous solution. Acids and bases that dissociate completely are said to be strong acids, e.g.:
- \(HClO_{4(aq)} \rightarrow H^+_{(aq)} + ClO^-_{4(aq)}\)
- \(HBr_{(aq)} \rightarrow H^+_{(aq)} + Br^-_{(aq)}\)
- \(CH_3O^-_{(aq)} + H_2O_{(l)} \rightarrow CH_3OH_{(aq)} + OH^-_{(aq)}\)
- \(NH^-_{2(aq)} + H_2O_{(l)} \rightarrow NH_{3(aq)} + OH^-_{(aq)}\)
Here the right-handed arrow (\(\rightarrow\)) implies that the reaction goes to completion. That is, a 1.0 M solution of HClO4 in water actually contains 1.0 M H+(aq) and 1.0 M ClO4-(aq), and no undissociated HClO4.
Conversely, weak acids such as acetic acid (CH3COOH) and weak bases such as ammonia (NH3) dissociate only slightly in water - typically a few percent, depending on their concentration and exist mostly as the undissociated molecules.
- STRONG ACIDS: HCl, HNO3, H2SO4, HBr, HI, HClO4
- WEAK ACIDS: All other acids, such as HCN, HF, H2S, HCOOH
Strong acids such as \(HCl\) dissociate to produce spectator ions such as \(Cl^-\) as conjugate bases, whereas weak acids produce weak conjugate bases. This is illustrated below for acetic acid and its conjugate base, the acetate anion. Acetic acid is a weak acid (Ka = 1.8 x 10-5) and acetate is a weak base (Kb = Kw/Ka = 5.6 x 10-10)
Like acids, strong and weak bases are classified by the extent of their ionization. Strong bases disassociate almost or entirely to completion in aqueous solution. Similar to strong acids, there are very few common strong bases. Weak bases are molecular compounds where the ionization is not complete.
- STRONG BASES: The hydroxides of the Group I and Group II metals such as LiOH, NaOH, KOH, RbOH, CsOH
- WEAK BASES: All other bases, such as NH3, CH3NH2, C5H5N
The strength of a conjugate acid/base varies inversely with the strength or weakness of its parent acid or base. Any acid or base is technically a conjugate acid or conjugate base also; these terms are simply used to identify species in solution (i.e acetic acid is the conjugate acid of the acetate anion, a base, while acetate is the conjugate base of acetic acid, an acid).
How does one define acids and bases? In chemistry, acids and bases have been defined differently by three sets of theories. One is the Arrhenius definition, which revolves around the idea that acids are substances that ionize (break off) in an aqueous solution to produce hydrogen (H+) ions while bases produce hydroxide (OH-) ions in solution. On the other hand, the Brønsted-Lowry definition defines acids as substances that donate protons (H+) whereas bases are substances that accept protons. Also, the Lewis theory of acids and bases states that acids are electron pair acceptors while bases are electron pair donors. Acids and bases can be defined by their physical and chemical observations.
pH Scale
Since acids increase the amount of H+ ions present and bases increase the amount of OH- ions, under the pH scale, the strength of acidity and basicity can be measured by its concentration of H+ ions. This scale is shown by the following formula:
pH = -log[H+]
with [H+] being the concentration of H+ ions.
To see how these calculations are done, refer to Calculating the pH of the solution of a Polyprotic Base/Acid
The pH scale is often measured on a 1 to 14 range, but this is incorrect (see pH for more details). Something with a pH less than 7 indicates acidic properties and greater than 7 indicates basic properties. A pH at exactly 7 is neutral. The higher the [H+], the lower the pH.
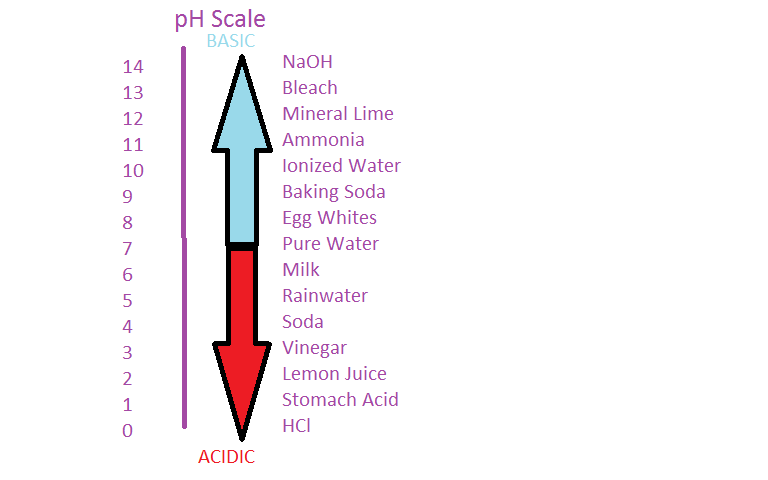
Lewis Theory
The Lewis theory of acids and bases states that acids act as electron pair acceptors and bases act as electron pair doners. This definition doesn't mention anything about the hydrogen atom at all, unlike the other definitions. It only talks about the transfer of electron pairs. To demonstrate this theory, consider the following example.
This is a reaction between ammonia (NH3) and boron trifluoride (BF3). Since there is no transfer of hydrogen atoms here, it is clear that this is a Lewis acid-base reaction. In this reaction, NH3 has a lone pair of electrons and BF3 has an incomplete octet, since boron doesn't have enough electrons around it to form an octet.

Because boron only has 6 electrons around it, it can hold 2 more. BF3 can act as a Lewis acid and accept the pair of electrons from the nitrogen in NH3, which will then form a bond between the nitrogen and the boron.

This is considered an acid-base reaction where NH3 (base) is donating the pair of electrons to BF3. BF3 (acid) is accepting those electrons to form a new compound, H3NBF3.
Neutralization
A special property of acids and bases is their ability to neutralize the other's properties. In an acid-base (or neutralization) reaction, the H+ ions from the acid and the OH- ions from the base react to create water (H2O). Another product of a neutralization reaction is an ionic compound called a salt. Therefore, the general form of an acid-base reaction is:
The following are examples of neutralization reactions:
1.
(NOTE: To see this reaction done experimentally, refer to the YouTube video link under the section "References".)
2.
Titrations
Titrations are performed with acids and bases to determine their concentrations. At the equivalence point, the number of moles of the acid will equal the number of moles of the base. This indicates that the reaction has been neutralized.
Neutralization: moles of acid = moles of base
Here's how the calculations are done:
For instance, hydrochloric acid is titrated with sodium hydroxide:
For instance, 30 mL of 1.00 M NaOH is needed to titrate 60 mL of an HCl solution. The concentration of HCl needs to be determined. At the eqivalence point:
moles of HCl = moles of NaOH
To solve for the molarity of HCl, plug in the given data into the equation above.
MHCl(60 mL HCl) = (1.00 M NaOH)(30 mL NaOH)
MHCl=0.5 M
The concentration of HCl is 0.5 M.
Sample Problems
1. Which of the following compounds is a strong acid?
- CaSO4
- NaCl
- HNO3
- NH3
Solution: There are 6 strong acids and all other acids are considered weak. HNO3 is one of those 6 strong acids, while NH3 is actuallly a weak base.
The answer is (3) HNO3.
2. Which of the following compounds is a Brønsted-Lowry base?
- HCl
- HPO42-
- H3PO4
- NH4+
- CH3NH3+
Solution: A Brønsted-Lowry Base is a proton acceptor, which means it will take in an H+. This eliminates HCl, H3PO4 ,NH4+ and CH3NH3+ because they are Brønsted-Lowry acids. They all give away protons. In the case of HPO42-, consider the following equation:
Here, it is clear that HPO42- is the acid since it donates a proton to water to make H3O+ and PO43-. Now consider the following equation:
In this case, HPO42- is the base since it accepts a proton from water to form H2PO4- and OH-. Thus, HPO42- is an acid and base together, making it amphoteric.
Since HPO42- is the only compound from the options that can act as a base, the answer is (2) HPO42-.
3. A 50 ml solution of 0.5 M NaOH is titrated until neutralized into a 25 ml sample of HCl. What was the concentration of the HCl?
Solution: Since the number of moles of acid equals the number of moles of base at neutralization, the following equation is used to solve for the molarity of HCl:
Now, plug into the equation all the information that is given:
MHCl(25 mL HCl) = (0.5 MNaOH)(50 mL NaOH)
MHCl = 1
The correct answer is 1 MHCl.
4. In the following acid-base neutralization, 2.79 g of the acid HBr (80.91g/mol) neutralized 22.72 mL of a basic aqueous solution by the reaction:
Calculate the molarity of the basic solution.
Solution:
First, the number of moles of the acid needs to be calculated. This is done by using the molar mass of HBr to convert 2.79 g of HBr to moles.
(2.79 g HBr)/(80.91 g/mol HBr) = 0.0345 moles HBr
Since this is a neutralization reaction, the number of moles of the acid (HBr) equals the number of moles of the base (NaOH) at neutralization:
moles of acid = moles of base
0.0345 moles HBr = 0.0345 moles NaOH
The molarity of NaOH can now be determined since the amount of moles are found and the volume is given. Convert 22.72 mL to Liters first since molarity is in units of moles/L.
Molarity = (0.0345 moles NaOH)/(0.02272 L NaOH) = 1.52 MNaOH
The correct answer is 1.52 MNaOH.
5. Which of the following is a Brønsted-Lowry base but not an Arrhenius base?
- NH3
- NaOH
- Ca(OH)2
- KOH
Solution: The Brønsted-Lowry definition says that a base accepts protons (H+ ions). NaOH, Ca(OH)2, and KOH are all Arrhenius bases because they yield the hydroxide ion (OH-) when they ionize. However, NH3 does not dissociate in water like the others. Instead, it takes a proton from water and becomes NH4 while water becomes a hydroxide.
Therefore, the correct answer is (1) NH3.
References
-
Brent, Lynnette. Acids and Bases. New York, NY: Crabtree Pub., 2009. Print.
-
Hulanicki, Adam. Reactions of Acids and Bases in Analytical Chemistry. Ellis Horwood Limited: 1987.
-
Oxlade, Chris. Acids & Bases. Chicago, IL: Heinemann Library, 2002. Print.
- Petrucci, Ralph H. General Chemistry: Principles and Modern Applications. Macmillian: 2007.
- Vanderwerd, Calvin A. Acids, Bases, and the Chemistry of the Covalent Bond. Reinhold: 1961.
Outside Links
- en.Wikipedia.org/wiki/Br%C3%B8nsted%E2%80%93Lowry_acid-base_theory (Wikipedia~Brønsted-Lowry Acid-Base Theory)
- www.youtube.com/watch?v=erWTsWut7Vc
- http://www.livestrong.com/article/105395-acids-bases/
- www.files.chem.vt.edu/RVGS/AC...and_bases.html
Contributors and Attributions
- Catherine Broderick (UCD), Marianne Moussa (UCD)
Jim Clark (Chemguide.co.uk)