8.7: Making radioisotopes for medical uses
- Page ID
- 372955
Natural radioisotopes usually have a long half-life and are not best suited for medical applications. The medical application usually requires short-lived radioisotopes. The radioisotopes are usually produced in nuclear reactors where particles, like \(\ce{\alpha}\)-particles, \(\ce{\beta}\)-particles, and neutrons, are abundant. Particle accelerators, such as the one shown in Fig. 8.7.1 also accelerate and direct the nuclear particles at the targets. The high-energy nuclear particles may be absorbed by and transmute the target nuclei to radioisotopes in a nuclear reaction.
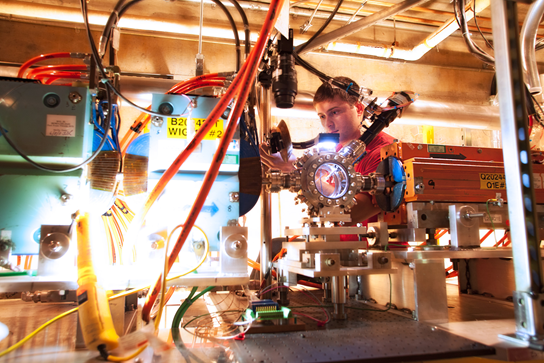
Radioisotopes in medical applications are usually produced by the particle bombardment method. One reaction that happens naturally by neutron bombardment from cosmic rays on nitrogen-14 is the following.
\[\ce{_7^14N + _{0}^{1}{n} -> _6^14C + _{1}^{1}{p}}\nonumber\]
An example of an artificial nuclear reaction initiated by \(\ce{\alpha}\)-particle bombardment on nitrogen, observed by Rutherford that lead to the discovery of proton, is illustrated in Fig. 8.7.2.
\[\ce{_2^4He + _{7}^{14}{N} -> _8^17O + _{1}^{1}{p}}\nonumber\]
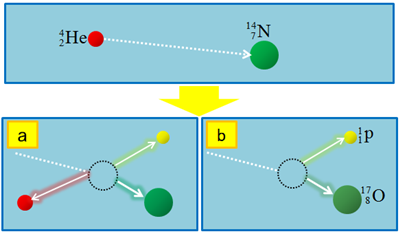
Another example is the nuclear reaction initiated by \(\ce{\alpha}\)-particles on beryllium, observed by James Chadwick, which lead to the discovery of the neutron.
\[\ce{_2^4He + _{4}^{9}{Be} -> _6^12C + _{0}^{1}{n}}\nonumber\]
An example of radioisotope production for medical uses is the following. Gold-198, used as a tracer in the liver, is produced by neutron bombardment on gold-197.
\[\ce{_79^197Au + _{0}^{1}{n} -> _79^198Au}\nonumber\]
Similarly, gallium-67 used in medical diagnostics is produced by proton bombardment on zinc-66.
\[\ce{_30^66Zn + _{1}^{1}{p} -> _31^67Ga}\nonumber\]
Molybdenum-99 is a radioactive isotope produced in a nuclear reactor by neutron bombardment of molybdenum-98.
\[\ce{_42^98Mo + _{0}^{1}{n} -> _42^99Mo} + \gamma\nonumber\]
Molybdenum-99 is also produced as a fission product of uranium-235. Molybdenum-99 decays to technetium-99m that has several uses in nuclear medical imaging and treatment.
\[\ce{_42^99Mo -> _43^{99m}{Tc} + _{-1}^{0}{e}}\nonumber\]
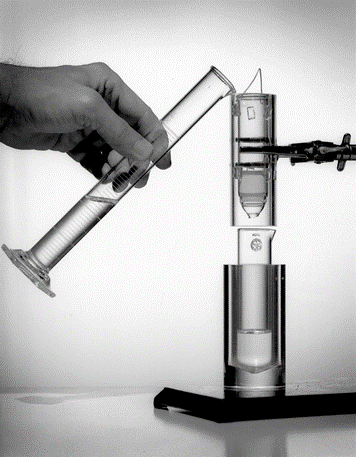
Technetium-99m is short-lived (half-life 6 h), and needs to be produced in the hospital to minimize its decay during the transport. Its parent molybdenum-99 has a half-life of 66h and can be transported without significant decay during the transport. Molybdenum-99/technetium-99m generators are supplied to the hospitals in a shielded container. Fig. 8.7.3 illustrates the first Molybdenum-99/technetium-99m generator developed at Brookhaven National Laboratory. Molybdate (MoO42) ion is adsorbed onto alumina adsorbent in a column. When molybdenum-99 decays to technetium-99m, the ion change to pertechnetate (TcO4‑), which is less tightly bound to the alumina. Pouring a saline solution through the column elutes the technetium-99m as TcO4‑ ion, which is then used for medical purposes in the hospitals.
Destruction of an inoperable tumor has also been tested by \(\ce{\alpha}\)-emission from boron-10 upon neutron bombardment.
\[\ce{_{0}^{1}{n} + _5^10B -> _4^7Li + _2^4He}\nonumber\]