8.8: Nuclear fusion and fission
- Page ID
- 372956
\( \newcommand{\vecs}[1]{\overset { \scriptstyle \rightharpoonup} {\mathbf{#1}} } \)
\( \newcommand{\vecd}[1]{\overset{-\!-\!\rightharpoonup}{\vphantom{a}\smash {#1}}} \)
\( \newcommand{\id}{\mathrm{id}}\) \( \newcommand{\Span}{\mathrm{span}}\)
( \newcommand{\kernel}{\mathrm{null}\,}\) \( \newcommand{\range}{\mathrm{range}\,}\)
\( \newcommand{\RealPart}{\mathrm{Re}}\) \( \newcommand{\ImaginaryPart}{\mathrm{Im}}\)
\( \newcommand{\Argument}{\mathrm{Arg}}\) \( \newcommand{\norm}[1]{\| #1 \|}\)
\( \newcommand{\inner}[2]{\langle #1, #2 \rangle}\)
\( \newcommand{\Span}{\mathrm{span}}\)
\( \newcommand{\id}{\mathrm{id}}\)
\( \newcommand{\Span}{\mathrm{span}}\)
\( \newcommand{\kernel}{\mathrm{null}\,}\)
\( \newcommand{\range}{\mathrm{range}\,}\)
\( \newcommand{\RealPart}{\mathrm{Re}}\)
\( \newcommand{\ImaginaryPart}{\mathrm{Im}}\)
\( \newcommand{\Argument}{\mathrm{Arg}}\)
\( \newcommand{\norm}[1]{\| #1 \|}\)
\( \newcommand{\inner}[2]{\langle #1, #2 \rangle}\)
\( \newcommand{\Span}{\mathrm{span}}\) \( \newcommand{\AA}{\unicode[.8,0]{x212B}}\)
\( \newcommand{\vectorA}[1]{\vec{#1}} % arrow\)
\( \newcommand{\vectorAt}[1]{\vec{\text{#1}}} % arrow\)
\( \newcommand{\vectorB}[1]{\overset { \scriptstyle \rightharpoonup} {\mathbf{#1}} } \)
\( \newcommand{\vectorC}[1]{\textbf{#1}} \)
\( \newcommand{\vectorD}[1]{\overrightarrow{#1}} \)
\( \newcommand{\vectorDt}[1]{\overrightarrow{\text{#1}}} \)
\( \newcommand{\vectE}[1]{\overset{-\!-\!\rightharpoonup}{\vphantom{a}\smash{\mathbf {#1}}}} \)
\( \newcommand{\vecs}[1]{\overset { \scriptstyle \rightharpoonup} {\mathbf{#1}} } \)
\( \newcommand{\vecd}[1]{\overset{-\!-\!\rightharpoonup}{\vphantom{a}\smash {#1}}} \)
\(\newcommand{\avec}{\mathbf a}\) \(\newcommand{\bvec}{\mathbf b}\) \(\newcommand{\cvec}{\mathbf c}\) \(\newcommand{\dvec}{\mathbf d}\) \(\newcommand{\dtil}{\widetilde{\mathbf d}}\) \(\newcommand{\evec}{\mathbf e}\) \(\newcommand{\fvec}{\mathbf f}\) \(\newcommand{\nvec}{\mathbf n}\) \(\newcommand{\pvec}{\mathbf p}\) \(\newcommand{\qvec}{\mathbf q}\) \(\newcommand{\svec}{\mathbf s}\) \(\newcommand{\tvec}{\mathbf t}\) \(\newcommand{\uvec}{\mathbf u}\) \(\newcommand{\vvec}{\mathbf v}\) \(\newcommand{\wvec}{\mathbf w}\) \(\newcommand{\xvec}{\mathbf x}\) \(\newcommand{\yvec}{\mathbf y}\) \(\newcommand{\zvec}{\mathbf z}\) \(\newcommand{\rvec}{\mathbf r}\) \(\newcommand{\mvec}{\mathbf m}\) \(\newcommand{\zerovec}{\mathbf 0}\) \(\newcommand{\onevec}{\mathbf 1}\) \(\newcommand{\real}{\mathbb R}\) \(\newcommand{\twovec}[2]{\left[\begin{array}{r}#1 \\ #2 \end{array}\right]}\) \(\newcommand{\ctwovec}[2]{\left[\begin{array}{c}#1 \\ #2 \end{array}\right]}\) \(\newcommand{\threevec}[3]{\left[\begin{array}{r}#1 \\ #2 \\ #3 \end{array}\right]}\) \(\newcommand{\cthreevec}[3]{\left[\begin{array}{c}#1 \\ #2 \\ #3 \end{array}\right]}\) \(\newcommand{\fourvec}[4]{\left[\begin{array}{r}#1 \\ #2 \\ #3 \\ #4 \end{array}\right]}\) \(\newcommand{\cfourvec}[4]{\left[\begin{array}{c}#1 \\ #2 \\ #3 \\ #4 \end{array}\right]}\) \(\newcommand{\fivevec}[5]{\left[\begin{array}{r}#1 \\ #2 \\ #3 \\ #4 \\ #5 \\ \end{array}\right]}\) \(\newcommand{\cfivevec}[5]{\left[\begin{array}{c}#1 \\ #2 \\ #3 \\ #4 \\ #5 \\ \end{array}\right]}\) \(\newcommand{\mattwo}[4]{\left[\begin{array}{rr}#1 \amp #2 \\ #3 \amp #4 \\ \end{array}\right]}\) \(\newcommand{\laspan}[1]{\text{Span}\{#1\}}\) \(\newcommand{\bcal}{\cal B}\) \(\newcommand{\ccal}{\cal C}\) \(\newcommand{\scal}{\cal S}\) \(\newcommand{\wcal}{\cal W}\) \(\newcommand{\ecal}{\cal E}\) \(\newcommand{\coords}[2]{\left\{#1\right\}_{#2}}\) \(\newcommand{\gray}[1]{\color{gray}{#1}}\) \(\newcommand{\lgray}[1]{\color{lightgray}{#1}}\) \(\newcommand{\rank}{\operatorname{rank}}\) \(\newcommand{\row}{\text{Row}}\) \(\newcommand{\col}{\text{Col}}\) \(\renewcommand{\row}{\text{Row}}\) \(\newcommand{\nul}{\text{Nul}}\) \(\newcommand{\var}{\text{Var}}\) \(\newcommand{\corr}{\text{corr}}\) \(\newcommand{\len}[1]{\left|#1\right|}\) \(\newcommand{\bbar}{\overline{\bvec}}\) \(\newcommand{\bhat}{\widehat{\bvec}}\) \(\newcommand{\bperp}{\bvec^\perp}\) \(\newcommand{\xhat}{\widehat{\xvec}}\) \(\newcommand{\vhat}{\widehat{\vvec}}\) \(\newcommand{\uhat}{\widehat{\uvec}}\) \(\newcommand{\what}{\widehat{\wvec}}\) \(\newcommand{\Sighat}{\widehat{\Sigma}}\) \(\newcommand{\lt}{<}\) \(\newcommand{\gt}{>}\) \(\newcommand{\amp}{&}\) \(\definecolor{fillinmathshade}{gray}{0.9}\)Conversion of matter into energy in nuclear reactions
When free nucleons come together to make a nucleoid, they release energy equal to the nuclear binding energy. The energy is produced at the expense of the mass of the nucleons following the famous Einstein equation:
\(E = mc^2\)
, where E is the energy, m is the mass, and c is the speed of light. In other words, the mass of nucleons in nucleoids is slightly less than the mass of the free nucleons, as some of their mass is released as nuclear binding energy.
The nuclear binding energy per nucleon is different for different nucleoids, as shown in Fig. 8.8.1. The composition of nucleoids changes during the nuclear reaction, and the difference in the nuclear binding energy is released as energy during the process, which is the source of energy in the sun, stars, nuclear power plants, and nuclear weapons.

The most stable nucleoid with the highest average binding energy per nucleon is iron-56.
The lighter nucleoids tend to combine and make heavier nucleoids that are more stable and lease a tremendous amount of energy –a process called nuclear fusions.
The heavier nucleoids, particularly those having a mass number higher than 92, tend to split into two smaller nucleoids that are more stable and lease a large amount of energy –a process called nuclear fission.
Both the fusion and fission processes release a tremendous amount of energy, called nuclear energy or atomic energy, which is the difference between the nuclear binding energy of the parent and the daughter nucleoid.
Nuclear fusion -a source of energy in the sun and stars
The universe is composed of about 98% hydrogen and helium. Sun is composed of about 74% hydrogen, 25% helium, and 1% all other elements. The light nucleoid like hydrogen and helium fuse to make heavier nucleoids and subatomic particles like neutrons in a process called nuclear fusion. Nuclear fusion releases energy that is the difference in the nuclear binding energy of their nucleons in the daughter and parent nucleoids. Fusion energy is the source of energy for the sun and stars. Some of the nuclear fusion reactions, illustrated in Fig. 8.1.1 are the following.
\[\ce{_1^1H + _1^1H -> _2^2H + _{1}^{0}{e}}\nonumber\]
\[\ce{_1^1H + _1^2H -> _2^3H} \nonumber\]
\[\ce{_2^3H + _2^3H -> _2^4H + 2_{1}^{1}{H}}\nonumber\]
\[\ce{_2^3H + _1^1H -> _2^4H + _{1}^{0}{e}}\nonumber\]
Although hydrogen is abundant on earth and a potential source of energy, the controlled fusion reaction is not yet economically feasible. The main difficulty is that the fusion of nuclei requires extremely high temperature, greater than a hundred thousand Celsius, and extremely high pressure, greater than a hundred thousand atmospheres, to overcome the repulsive forces of the like charges of the nuclei before they can fuse. For this reason, nuclear fusion reactions are also called thermonuclear reactions. Such conditions exist in sun and stars where these reactions routinely happen, but there are many technical problems yet to overcome before the energy can be harnessed economically from the nuclear fusion reactions on earth. A nuclear explosion can create the conditions needed for the thermonuclear reactions and are used to carry out uncontrolled thermonuclear reactions to boost the explosive power of nuclear weapons. Such a nuclear weapon is called a thermonuclear or hydrogen bomb.

One of the potential candidate reactions for nuclear fusion reactors is the fusion of deuterium with tritium, illustrated in Fig. 8.8.2, which requires a little less harsh conditions. Most of the research is focused on the device called Tokamak, which uses strong magnetic fields to contain and heat the materials for the reaction, but so far it is not economically feasible to use it as a source of energy for commercial uses.
Nuclear fission -the source of energy in nuclear power plants and in nuclear weapons
Nuclear fission is a radioactive decay process in which a heavier nucleoid splits into two or lighter nucleoids and releases a tremendous amount of energy that is the difference in the binding energy of nucleons in the daughter and the parent nucleoids. The nucleoids that do spontaneous fission are marked green in Fig. 8.2.1.
Nuclear fuel
Induced fission is fission that occurs upon bombardment of a nucleoid with a nuclear particle, like a neutron. For example, the bombardment of uranium-235 or plutonium-239 by a neutron causes the fission and releases about three neutrons along with the smaller nucleoids, as illustrated in Fig. 8.8.3. The neutrons released by the fission cause fission of another nucleoid starting a nuclear chain reaction. The nuclei like uranium-235 or plutonium-239 that produce neutrons in the product that are capable of continuing the fission in a chain reaction are called fissile. The fissile nucleoids are nuclear fuels used in nuclear power plants to produce nuclear energy.
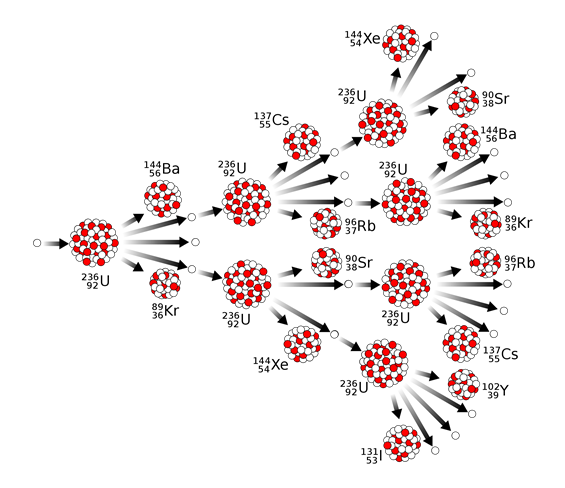
The neutrons released in a fission event may be lost, absorbed by another nucleus like uranium-238 that does not fission, or may be absorbed by another fissile nucleoid and repeat the fission, as illustrated in Fig. 8.8.4. Three situations may arise, If, on the average;
- less than one neutron released causes new fission, the process dyes,
- one neutron released causes new fission, the fission continues in a controlled way as in nuclear power plants,
- more than one neutron released causes new fission, the fission increases exponentially resulting in a nuclear explosion, as illustrated in Fig. 8.8.3.

Note that each fission event does not necessarily split the parent nucleoid into exact same daughter nucleoids, it can split into different combinations of daughter nucleoids resulting in a mixture of products that are usually radioactive, as illustrated in Fig. 8.8.4.
Atomic bomb
A certain minimum mass of the fissile material, called critical mass, is necessary for a fission event to grow exponentially and cause a nuclear explosion. The critical mass is less for a more enriched fissile material. Therefore, usually enriched uranium-235 or plutonium 239 is used as an explosive in an atomic bomb. The mass more than the critical mass, called supercritical mass, is split into sub-critical portions which are kept separate in the weapon to avoid a nuclear explosion. When needed, the conventional chemical explosion is used to force the sub-critical portions to combine and make a supercritical mass for a nuclear explosion to happen, as illustrated in Fig. 8.8.5.
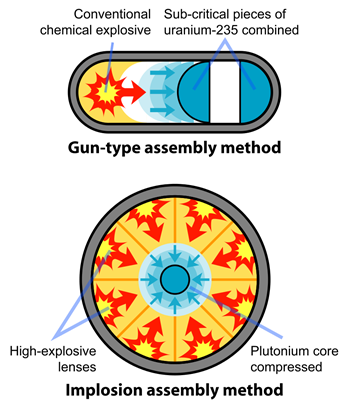
Nuclear power plant
Nuclear power plants, like the one illustrated in Fig. 8.8.6, harness the energy released during fission for electricity production. The fissile material is assembled in the form of fuel rods in the core of a nuclear reactor. It is important to sustain the nuclear chain reaction but it is critical to keep it under control so that the chain reaction may not grow exponentially and cause a nuclear explosion. Rods of material, like palladium or boron that are efficient neutron absorbers, are included in the design of the reactor core. The neutron absorber rods can be lowered or raised to control the neutron flux available to sustain the fission chain reaction.
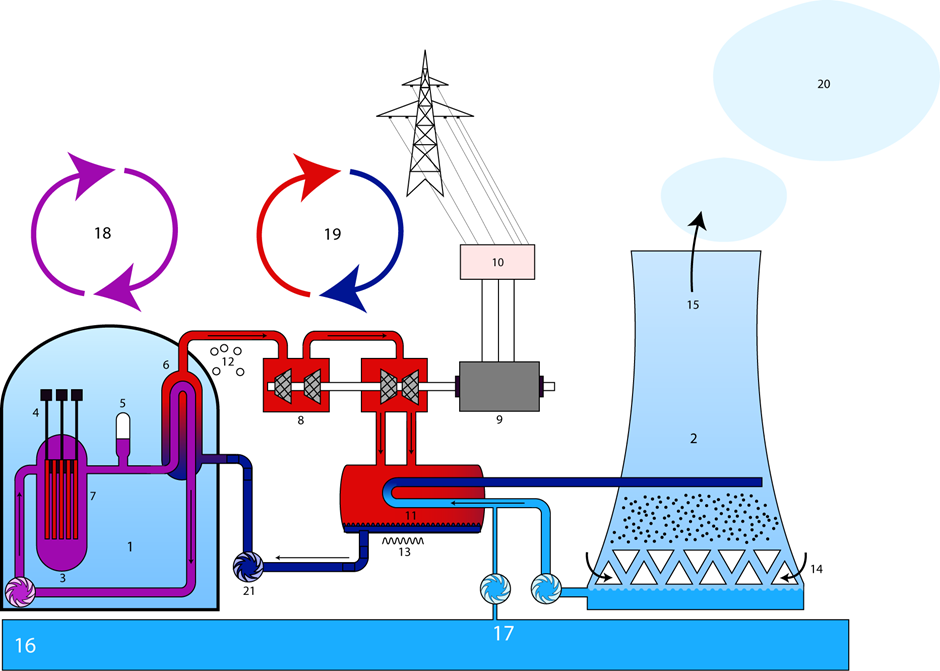
1. reactor block, 2. cooling tower, 3. reactor, 4. control rod, 5. support for pressure, 6. steam generator, 7. fuel element, 8. turbine, 9. generator, 10. transformer, 11. condenser, 12. gaseous, 13. liquid, 14.air, 15.air (humid), 16. river, 17. cooling-water circulation, 18. primary circuit, 19. secondary circuit, 20. water vapor, 21. pump. Source: Steffen Kuntoff / CC BY-SA 2.0 DE (https://creativecommons.org/licenses...2.0/de/deed.en)
The reactor core is surrounded by a coolant and moderator, which may be regular water (H2O) or heavy water (D2O), or some other material. It is called coolant because it takes away the heat produced by the fission reaction and moderator because it also slows down the fast neutrons released in fission. The slow neutrons are more effective for the fission reaction. The coolant transfers its heat to a steam generator. All of these components are housed in a containment building to keep the radioactivity contained, even if a nuclear accident happens. The steam is used to drive a turbine for electricity production, and the condensed water is cooled using water from the cooling tower and returned for reuse, as illustrated in Fig. 8.8.6.
Nuclear power plants produce about 20% of the electricity in the USA. Fig. 8.8.7 shows a nuclear power plant in Arkansas. In some countries, the contribution of nuclear power to the total electricity is much higher, e.g., about 70% of electricity is produced by nuclear power plants in France. Nuclear power is considered an energy source of the future, or at least for a transition from fossil fuel to the next major energy source when the fossil fuel reserves will exhaust.
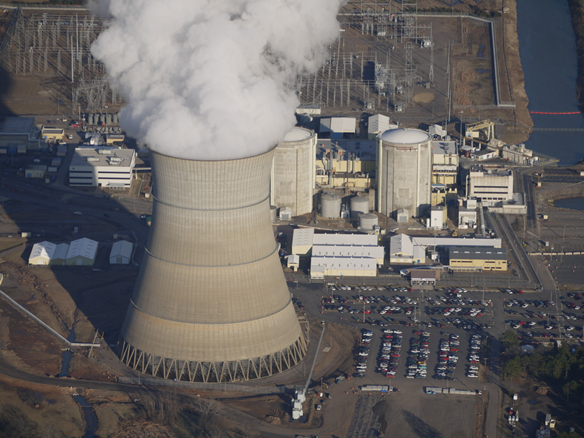
The fission products in the nuclear power plants become radioactive waste that needs to be stored for at least 10 half-lives to reach an acceptable radioactivity level. Based on the 28.8 years half-life of strontium-90 which is the long-lived and dangerous product in nuclear waste, a storage time of 300 years is needed. Handling radioactive waste is an issue of concern associated with nuclear power production. Plutonium-239 is another radioactive isotope produced from neutron absorption by uranium-238. Uranium-238 is a major constituent (99.28%) in the natural uranium along with fissile uranium-235 (0.71%). Plutonium-239 has a long half-life (24,000 Years) but it can be extracted in a fuel reprocessing plant and used as a nuclear fuel.