12.1: Organometallic reactions
- Page ID
- 283108
\( \newcommand{\vecs}[1]{\overset { \scriptstyle \rightharpoonup} {\mathbf{#1}} } \)
\( \newcommand{\vecd}[1]{\overset{-\!-\!\rightharpoonup}{\vphantom{a}\smash {#1}}} \)
\( \newcommand{\id}{\mathrm{id}}\) \( \newcommand{\Span}{\mathrm{span}}\)
( \newcommand{\kernel}{\mathrm{null}\,}\) \( \newcommand{\range}{\mathrm{range}\,}\)
\( \newcommand{\RealPart}{\mathrm{Re}}\) \( \newcommand{\ImaginaryPart}{\mathrm{Im}}\)
\( \newcommand{\Argument}{\mathrm{Arg}}\) \( \newcommand{\norm}[1]{\| #1 \|}\)
\( \newcommand{\inner}[2]{\langle #1, #2 \rangle}\)
\( \newcommand{\Span}{\mathrm{span}}\)
\( \newcommand{\id}{\mathrm{id}}\)
\( \newcommand{\Span}{\mathrm{span}}\)
\( \newcommand{\kernel}{\mathrm{null}\,}\)
\( \newcommand{\range}{\mathrm{range}\,}\)
\( \newcommand{\RealPart}{\mathrm{Re}}\)
\( \newcommand{\ImaginaryPart}{\mathrm{Im}}\)
\( \newcommand{\Argument}{\mathrm{Arg}}\)
\( \newcommand{\norm}[1]{\| #1 \|}\)
\( \newcommand{\inner}[2]{\langle #1, #2 \rangle}\)
\( \newcommand{\Span}{\mathrm{span}}\) \( \newcommand{\AA}{\unicode[.8,0]{x212B}}\)
\( \newcommand{\vectorA}[1]{\vec{#1}} % arrow\)
\( \newcommand{\vectorAt}[1]{\vec{\text{#1}}} % arrow\)
\( \newcommand{\vectorB}[1]{\overset { \scriptstyle \rightharpoonup} {\mathbf{#1}} } \)
\( \newcommand{\vectorC}[1]{\textbf{#1}} \)
\( \newcommand{\vectorD}[1]{\overrightarrow{#1}} \)
\( \newcommand{\vectorDt}[1]{\overrightarrow{\text{#1}}} \)
\( \newcommand{\vectE}[1]{\overset{-\!-\!\rightharpoonup}{\vphantom{a}\smash{\mathbf {#1}}}} \)
\( \newcommand{\vecs}[1]{\overset { \scriptstyle \rightharpoonup} {\mathbf{#1}} } \)
\( \newcommand{\vecd}[1]{\overset{-\!-\!\rightharpoonup}{\vphantom{a}\smash {#1}}} \)
\(\newcommand{\avec}{\mathbf a}\) \(\newcommand{\bvec}{\mathbf b}\) \(\newcommand{\cvec}{\mathbf c}\) \(\newcommand{\dvec}{\mathbf d}\) \(\newcommand{\dtil}{\widetilde{\mathbf d}}\) \(\newcommand{\evec}{\mathbf e}\) \(\newcommand{\fvec}{\mathbf f}\) \(\newcommand{\nvec}{\mathbf n}\) \(\newcommand{\pvec}{\mathbf p}\) \(\newcommand{\qvec}{\mathbf q}\) \(\newcommand{\svec}{\mathbf s}\) \(\newcommand{\tvec}{\mathbf t}\) \(\newcommand{\uvec}{\mathbf u}\) \(\newcommand{\vvec}{\mathbf v}\) \(\newcommand{\wvec}{\mathbf w}\) \(\newcommand{\xvec}{\mathbf x}\) \(\newcommand{\yvec}{\mathbf y}\) \(\newcommand{\zvec}{\mathbf z}\) \(\newcommand{\rvec}{\mathbf r}\) \(\newcommand{\mvec}{\mathbf m}\) \(\newcommand{\zerovec}{\mathbf 0}\) \(\newcommand{\onevec}{\mathbf 1}\) \(\newcommand{\real}{\mathbb R}\) \(\newcommand{\twovec}[2]{\left[\begin{array}{r}#1 \\ #2 \end{array}\right]}\) \(\newcommand{\ctwovec}[2]{\left[\begin{array}{c}#1 \\ #2 \end{array}\right]}\) \(\newcommand{\threevec}[3]{\left[\begin{array}{r}#1 \\ #2 \\ #3 \end{array}\right]}\) \(\newcommand{\cthreevec}[3]{\left[\begin{array}{c}#1 \\ #2 \\ #3 \end{array}\right]}\) \(\newcommand{\fourvec}[4]{\left[\begin{array}{r}#1 \\ #2 \\ #3 \\ #4 \end{array}\right]}\) \(\newcommand{\cfourvec}[4]{\left[\begin{array}{c}#1 \\ #2 \\ #3 \\ #4 \end{array}\right]}\) \(\newcommand{\fivevec}[5]{\left[\begin{array}{r}#1 \\ #2 \\ #3 \\ #4 \\ #5 \\ \end{array}\right]}\) \(\newcommand{\cfivevec}[5]{\left[\begin{array}{c}#1 \\ #2 \\ #3 \\ #4 \\ #5 \\ \end{array}\right]}\) \(\newcommand{\mattwo}[4]{\left[\begin{array}{rr}#1 \amp #2 \\ #3 \amp #4 \\ \end{array}\right]}\) \(\newcommand{\laspan}[1]{\text{Span}\{#1\}}\) \(\newcommand{\bcal}{\cal B}\) \(\newcommand{\ccal}{\cal C}\) \(\newcommand{\scal}{\cal S}\) \(\newcommand{\wcal}{\cal W}\) \(\newcommand{\ecal}{\cal E}\) \(\newcommand{\coords}[2]{\left\{#1\right\}_{#2}}\) \(\newcommand{\gray}[1]{\color{gray}{#1}}\) \(\newcommand{\lgray}[1]{\color{lightgray}{#1}}\) \(\newcommand{\rank}{\operatorname{rank}}\) \(\newcommand{\row}{\text{Row}}\) \(\newcommand{\col}{\text{Col}}\) \(\renewcommand{\row}{\text{Row}}\) \(\newcommand{\nul}{\text{Nul}}\) \(\newcommand{\var}{\text{Var}}\) \(\newcommand{\corr}{\text{corr}}\) \(\newcommand{\len}[1]{\left|#1\right|}\) \(\newcommand{\bbar}{\overline{\bvec}}\) \(\newcommand{\bhat}{\widehat{\bvec}}\) \(\newcommand{\bperp}{\bvec^\perp}\) \(\newcommand{\xhat}{\widehat{\xvec}}\) \(\newcommand{\vhat}{\widehat{\vvec}}\) \(\newcommand{\uhat}{\widehat{\uvec}}\) \(\newcommand{\what}{\widehat{\wvec}}\) \(\newcommand{\Sighat}{\widehat{\Sigma}}\) \(\newcommand{\lt}{<}\) \(\newcommand{\gt}{>}\) \(\newcommand{\amp}{&}\) \(\definecolor{fillinmathshade}{gray}{0.9}\)Introduction
In this chapter we will discuss common reactions and catalysis of organometallic compounds. We can classify reactions into two main categories. The first one includes reactions with gain or loss of ligands. You will hear about four reactions that belong to this category: ligand substitution reactions, oxidative additions, reductive eliminations, and nucleophilic displacement. The latter three can involve a change in coordination number while ligand substitution reactions do not.
The second main category are reactions that involve the modification of ligands. There are two reactions you will learn about: The migratory insertion and the β-hydride elimination.
Oxidative Addition Reactions
Let us start with the oxidative addition. How is it defined? An oxidative addition is a reaction in which one or more ligands add to the metal center of a complex and oxidize it. This addition can occur in a cis- or trans-fashion. A cis-addition means that two ligands are added so that they have cis-orientation to each other after the reaction is complete. A trans-addition implies that two new ligands are in opposite, trans-position after they have been added. We can further distinguish between mononuclear and dinuclear additions. Mononuclear addition involves a complex with a single metal. Dinuclear additions are additions to a metal-metal bond. The addition cleaves the metal-metal bond and adds one ligand to each fragment.
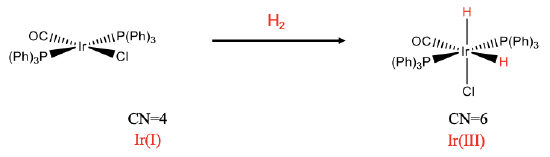
Here are a few of examples. The first example is a mononuclear cis-addition (Fig. \(\PageIndex{1}\):). In the reaction we add dihydrogen to a square planar iridium complex. After the reaction there are two additional hydrido ligands, and the coordination number has increased from 4 to 6. We can see that the two hydrido ligands are oriented in cis-fashion relative to each other. We can verify that the addition of the hydrido ligands oxidized the metal by determining the oxidation number of the metal before and after the reaction. There are three neutral ligands and one negatively charged chloro ligand. There is no overall charge at the complex. This means that the oxidation number of Ir is +1. After the reaction, there are the two hydrido ligands that have both a -1 charge. Remember, we get the charge at a ligand by cleaving the metal-ligand bond so that all electrons in the bond get assigned to the ligand. This means the oxidation number must be increased by +2, and is +3 after the reaction.
We can rationalize why the addition occurs in cis-fashion and not in trans-fashion. The two hydrido ligands are the smallest ligands, therefore, steric repulsion is minimized in the complex is minimized when the two hydrido ligands are in cis-position.
Fig. \(\PageIndex{2}\): Mononuclear oxidative trans-addition (Attribution: A. Vedernikov, U Maryland (modified))
The second example is a mononuclear trans-addition (Fig. \(\PageIndex{2}\):). In this reaction CH3Br is added to the iridium complex. In this case the two new ligands, a CH3 and the Br group are in opposite position. Again, the coordination number increases from four to six. We can again verify that an oxidation has occurred by analyzing the oxidation number of Ir. As previously determined, the oxidation number of iridium in the reactant complex is +1. Let us analyze the product complex. The chloro ligand and the bromo ligand have a 1- charge. In addition to that also the CH3 ligand has a 1- charge assuming that we treat the Ir-C bond as a dative bond. Thus, the oxidation number of Ir must be +3 to give a neutral complex.
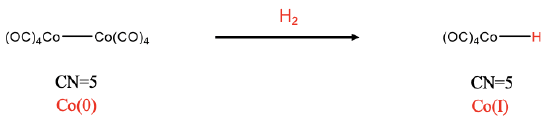
The third example is a dinuclear addition (Fig. \(\PageIndex{3}\):). This addition is neither cis nor trans because it cleaves a metal-metal bond, and the one new ligand is added to each fragment. In the example the Co-Co bond of the dicobalt octacarbonyl gets cleaved upon the reaction with dihydrogen, and two HCo(CO)4 complexes with Co-H bonds form. In this case the coordination number does not increase. Both the reactant and the products have the coordination number 5. We can see that an oxidation of Co has occurred by comparing the oxidation numbers. In the reactant the oxidation number of Co is zero because all CO ligands are neutral and the complex is overall neutral. In the carbonyl hydride product, Co has the oxidation number +1, because the hydrido ligand has a 1- charge.
Oxidative Additions with Intact Ligands
In the previous examples, the ligands that reacted with the complexes lost their integrity. The H-H bond in the H2 molecule cleaved to form the hydrido ligands, and the C-Br bond in CH3Br was cleaved to form separate Br and methyl ligands. Not all ligands lose their integrity upon their addition, in particular when they contain multiple bonds. These ligands are called intact ligands. In these cases the bond order within the ligand is reduced, but the number is still greater than zero.
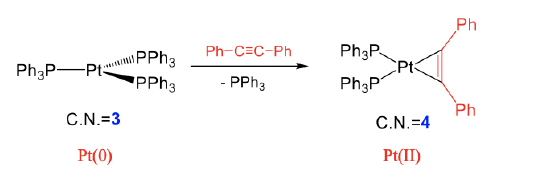
Examples for intact ligands are are alkenes, alkynes, O2, etc. In an oxidative addition with an alkyne for example, the alkyne binds side-on to the transition metal under the formation of two metal-C single bonds, and the reduction of the bond order within the alkyne from 3 to 2 (Fig. \(\PageIndex{4}\):). In the shown reaction, a diphenyl ethine molecule adds to tris(triphenyl phosphine) platinum under the formation of two Pt-C single bonds. The C-C triple bond in the ligand becomes a double bond. This reaction occurs under loss of one of the three triphenyl phosphine ligands. The coordination number at the metal increases from 3 to 4. We can again show that the reaction is oxidative by comparing the oxidation numbers of the metal. In the reactant, Pt has the oxidation number 0 because the three triphenyl phosphine ligands have no charge and the complex has overall no charge. After the reaction, the oxidation number of Pt is +2. This is because when we assign the four electrons of the two Pt-C single bonds to the ligand, the ligand becomes a (Ph-C=C-Ph)2- ligand with each C atom carrying a 1- charge. Because the product complex is overall neutral, the oxidation number of Pt is +2.
Reductive Elimination Reactions
The reductive elimination reaction is the reverse of the oxidative addition. The reductive elimination can only occur when the two ligands to be eliminated are in cis-position.
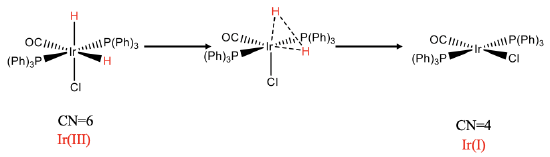
For instance the previously discussed oxidative cis-addition of H2 to the iridium complex is reversible, and the reverse is called the reductive elimination (Fig. \(\PageIndex{5}\):). The cis-orientation of the two hydrido ligands is necessary to form an H-H bond. The reaction can be thought of going along a reaction path in which the Ir-H bonds become gradually larger and and the H-H distance gradually smaller until the H2 molecule is eliminated from the complex.
Catalytic Deuteration of Benzene
Combinations of oxidative additions and reductive eliminations have many applications in the synthesis of organic molecules using an organometallic reactant.
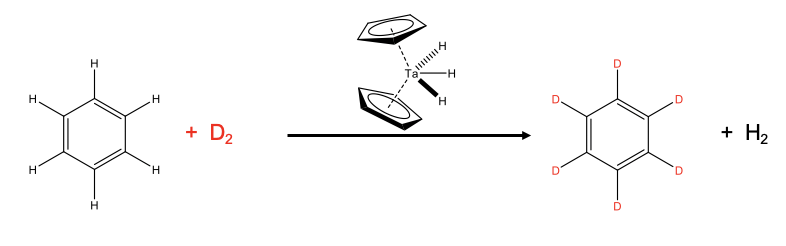
An example is the deuteration of benzene (Fig. \(\PageIndex{6}\):). Deuterated benzene is an important solvent in NMR spectroscopy. Industrially, the deuteration is done using a dicyclopentadienyltrihydridotantalum(V) catalyst starting out from benzene and D2. The D2 is provided in excess to drive the chemical equilibrium to the right side.
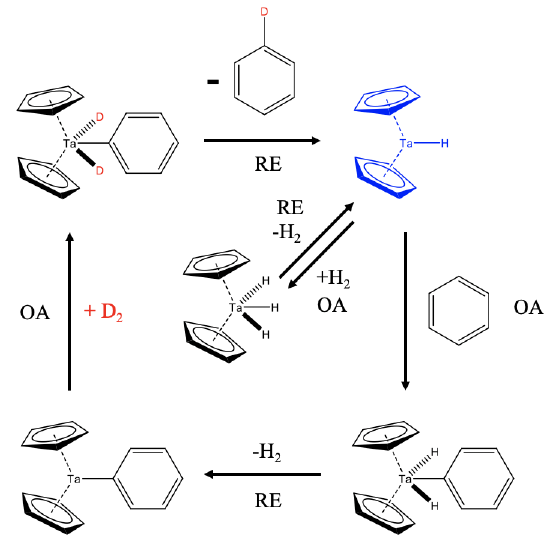
How does this reaction work mechanistically? This 18e Cp2TaH3 is actually a precatalyst that is in chemical equilibrium with H2 and the 16e dicyclopentadienylhydrido tantalum(III) which is the actual catalyst (Fig. \(\PageIndex{7}\):). Elimination of H2 from the Ta(V) catalyst is a reductive elimination (RE) and the re-addition of H2 to the Ta(III) species an oxidative addition (OA). In the presence of benzene, the Ta(III) species can oxidatively add benzene to form an 18e Ta(V) complex. This complex can reductively eliminate H2 to form a 16e Cp2Ta(III)-Ph complex. In the presence of deuterium this complex can add D2 oxidatively to form an 18e Cp2D2Ta(V)-Ph complex. This species can then reductively eliminate a monodeuterated benzene molecule under formation of Cp2Ta(III)-D. In the presence of enough D2 this monodeuterated benzene can be further deuterated in subsequent catalytic cycles.
Nucleophilic Displacement Reactions
Another important reaction is the nucleophilic displacement reaction. In these reactions a complex, typically an anion, acts as a nucleophile. The ligand is added, but no electrons are added to the complex. The reactions can be extremely useful for the synthesis of organic compounds. The utility results mostly from the fact that an organic electrophile is turned into a nucleophile.
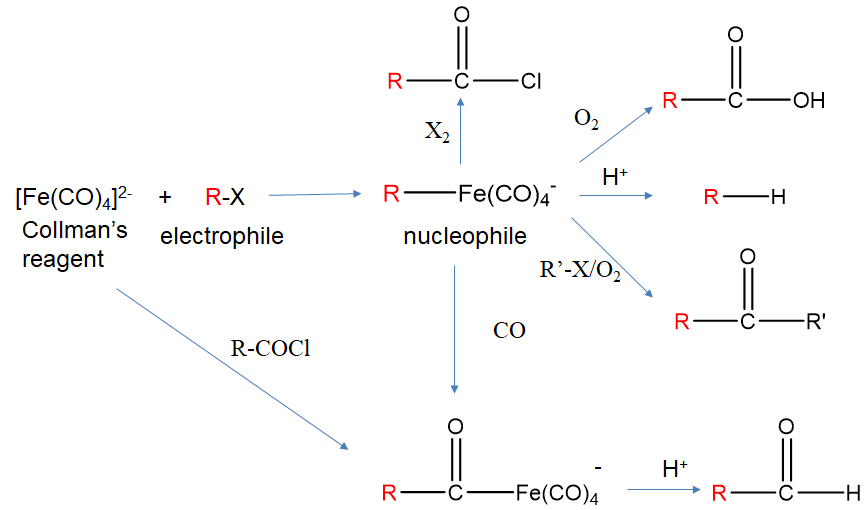
For example, tetracarbonyl ferrate (2-), also known as Collman’s reagent reacts with alkyl halides to form alkyl tetracarbonyl ferrate (-) (Fig. \(\PageIndex{8}\):). We can check that the addition of the alkyl group did not add any electrons by counting the electrons before and after the reaction. The Fe(CO)42- anion is an 18e complex. Applying the neutral atom method to the electron counting in [R-Fe(CO)4]- means that Fe contributes 8e, the 1- charge adds 1e, the CO ligands 4x2=8 electrons and the alkyl ligand 1e. This gives 8+1+8+1=18 electrons. Also the oxidation number of Fe has not changed upon the addition of the ligand. It is -2 before and after the reaction.
In the alkyltetracarbonyl ferrate (1-) anion, the group R which was formerly an electrophile in R-X, can now act as a nucleophile due to the fact that the Fe-C bond is polarized toward the C-atom. As a nucleophile it can for instance react with protons to form an alkane. It can also react with oxygen to form a carboxylic acid. In the presence of another alkyl halide R’-X it can form a ketone R-C(O)-R’. It can also insert CO into the Fe-C bonding in a so-called migratory insertion reaction, and the reaction product can react with acid to form an aldehyde. With dihalogens X2 it can form acyl chloride. Collman’s reagent cannot only add alkyl halides by also acyl halides to form acyl tetracarbonyl ferrates (-).
Migratory Insertion and Deinsertion (Elimination) Reactions
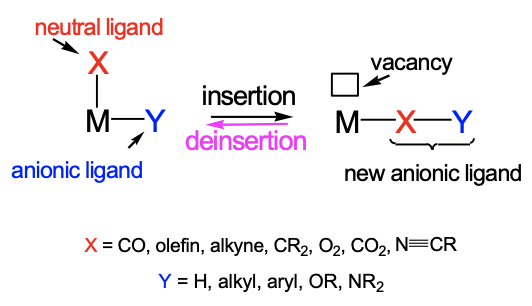
Our next reaction is the migratory insertion. In a migratory insertion reaction, there is an anionic ligand X and a neutral unsaturated ligand Y in cis-position, and the two ligands couple to form another anionic ligand XY that is attached to the same metal (Fig. \(\PageIndex{9}\):). This produces a vacancy at the metal, meaning a coordination site at the metal which is not occupied by a ligand. In an insertion reaction it is more common that the anionic ligand moves. The reaction is reversible and there is typically a chemical equilibrium. The reverse reaction is called a deinsertion. To drive the chemical equilibrium to the right side one can add the neutral ligand in excess or add a different neutral ligand. The neutral ligand then adds to the vacancy and prevents the reverse reaction. Typical neutral, unsaturated ligands are CO, olefins, alkynes, carbenes, dioxygen, carbon dioxide, and nitriles. Typical anionic ligands are hydrido, alkyl, aryl, alkoxy, and amido-ligands.
Carbonyl Migratory Insertions

One of the most common insertions are carbonyl insertions into alkyl groups like methyl groups (Fig. \(\PageIndex{10}\):). The carbonyl insertion produces an acyl group. To drive the reaction, the reaction can be carried out in the presence of a neutral ligand L like free CO, which occupies the vacant site.
Olefin Insertion and β-Hydride Elimination
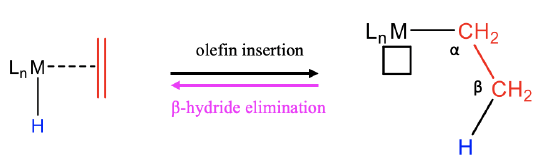
Another very common migratory insertion is the olefin migratory insertion into hydrides (Fig. \(\PageIndex{11}\):). This produces an alkyl group. When the reaction is carried out in the presence of CO which binds to the vacant site, then the reaction is driven to the right side. The reverse of this reaction is the β-hydride elimination. It is called “β”-elimination because of the H-atoms that are attached to the carbon atom in the alkyl chain that is second-closest to the metal are called “β”-hydrogens. The hydrogen atoms that are attached to the carbon which is bound to the carbon bonded to the metal via a metal-carbon bond are called “\(\alpha\)”-hydrogens.
Instability of Transition Metal Complexes Caused by β-Hydride Elimination
The β-hydride elimination is responsible for the frequent instability of transition metal alkyl complexes that possess β-hydrogen atoms.
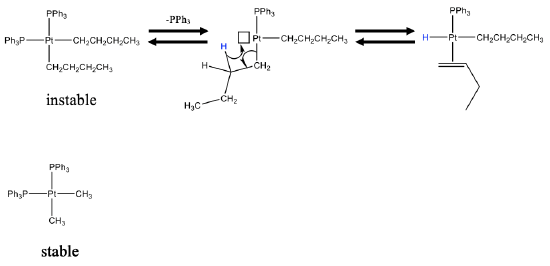
For example, the depicted dialkyldiphosphine platinum complex is unstable because it has β-hydrogen atoms (Fig. \(\PageIndex{12}\):). Statistically, the complex can lose a phospine complex at a certain rate due to the lability of the Pt-P bond leading to a complex with a vacant site. This complex can then undergo a β-elimination to form an olefin complex. In this case the olefin is a volatile butene molecule which can detach from the metal and leave the system unless the system is hermetically closed. In contrast, the respective methyl complex is stable because it only has α-hydrogens, but not β-hydrogens.
Carbene Migratory Insertion

Migratory insertions are also possible for carbenes. In this case, a CH2 group bonded to the metal via a M=C double bond plays the role of the neutral, unsaturated ligand. In the shown Re complex the carbene inserts into a Re-CH3 bond to form an ethyl group. This ethyl group is unstable because it can undergo β-hydride elimination to form an alkene complex with a hydride ligand.
Dr. Kai Landskron (Lehigh University). If you like this textbook, please consider to make a donation to support the author's research at Lehigh University: Click Here to Donate.