9.3: Amino Acids, Proteins, and pH
- Page ID
- 357487
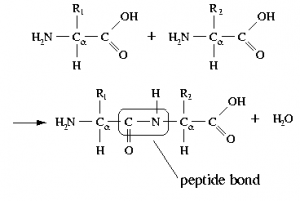
In addition to examining how adding a strong acid or base affects a buffer solution, we can also look at the effect of \(\mathrm{pH}\) on a particular acid or base. This is particularly important in biological systems where there are many weak acid or base groups that can be affected by the \(\mathrm{pH}\). For example, proteins contain both weakly acidic \(–\mathrm{COOH}\) and weakly basic \(–\mathrm{NH}_{2}\) groups. A \(1.0-\mathrm{M}\) solution of a simple carboxylic acid like acetic acid has a \(\mathrm{pH}\) of \(\sim 2.8\); it turns out that most carboxylic acids behave in a similar way. If we manipulate the \(\mathrm{pH}\), for example, by adding a strong base, the acetic acid reacts with the base to form an acetate ion. Based on the Henderson–Hasselbalch equation, when [acetate] = [acetic acid], the \(\mathrm{pH}\) equals the acid’s \(\mathrm{pK}_{a}\), which is \(4.74\). As the \(\mathrm{pH}\) increases, the concentration of acetate must also increase, until by \(\mathrm{pH} \sim 7\) (approximately normal physiological \(\mathrm{pH}\)). At this point the concentration of acetic acid is very small indeed. The ratio of base to acid is about \(200/1\). That is, at physiological \(\mathrm{pH}\)'s groups such as carboxylic acids are deprotonated and exist in the carboxylate (negatively charged) form.
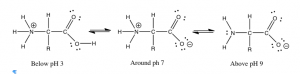
Conversely, if we look at the amino group (\(–\mathrm{NH}_{2}\)) of a protein, it is actually the base part of a conjugate acid-base pair in which the acid is the protonated form \(–\mathrm{NH}_{3}{}^{+}\). The \(\mathrm{pK}_{a}\) of an \(–\mathrm{NH}_{3}{}^{+}\) group is typically \(\sim 9\). At a \(\mathrm{pH}\) of \(9\) there are equal amounts of the protonated (\(–\mathrm{NH}_{2}\)) and unprotonated forms (\(–\mathrm{NH}_{3}{}^{+}\)). So if we change the \(\mathrm{pH}\) by adding an acid, the concentration of \(–\mathrm{NH}_{3}{}^{+}\) form increases as the base form \(–\mathrm{NH}_{2}\) is protonated. At \(\mathrm{pH} \sim 7\) there is little of the \(–\mathrm{NH}_{2}\) form remaining. Interestingly, this means that an amino acid (shown in the figure) never exists in a state where both the amino (\(–\mathrm{NH}_{2}\)) group and the carboxylic acid (\(–\mathrm{CO}_{2}\mathrm{H}\)) exist at the same time. The “neutral” species is in fact the one in which \(–\mathrm{NH}_{3}{}^{+} / –\mathrm{CO}_{2}\)– are present. This zwitterion (that is a neutral molecules with a positive and negative electrical charge at different locations, from the German zwitter, meaning “between”) is the predominant form at physiological \(\mathrm{pH}\).
A protein is composed mainly (sometimes solely) of polymers of amino acids, known as polypeptides. In a polypeptide, the amino (\(–\mathrm{NH}_{2}\)) and carboxylic acid (\(–\mathrm{CO}_{2}\mathrm{H}\)) groups of amino acids are bonded together to form a peptide bond (see figure). The resulting amide group (peptide bond) is neither acidic nor basic under physiological conditions.[9] That being said, many of the amino acids found in proteins have acidic (aspartic acid or glutamic acid) or basic (lysine, arginine, or histidine) side chains. The \(\mathrm{pH}\) of the environment influences the conformations of the protein molecule and the interactions between these charged side chains (the spontaneous native conformations of the molecule are called protein folding). Changes from the “normal” environment can lead to changes in protein structure, and this in turn can change biological activity. In some cases, protein activity is regulated by environmental \(\mathrm{pH}\). In other cases, changes in \(\mathrm{pH}\) can lead to protein misfolding (or denaturation, which in living organisms can cause disruption of cell activity or death). For example, if these groups are protonated or deprotonated, the electronic environment in that region of the protein can change drastically, which may mean that the protein will not only change how it interacts with other species but its shape may change so as to minimize repulsive interactions or produce new attractive interactions. Small changes in protein shape can have profound effects on how the protein interacts with other molecules and, if it is a catalyst, its efficiency and specificity. In fact, there are cases where environmental \(\mathrm{pH}\) is used to regulate protein activity.
Questions
Questions to answer:
- What would be the ratio of \(–\mathrm{NH}_{3}{}^{+} / –\mathrm{NH}_{2}\) in a solution of a protein at \(\mathrm{pH} 5\), \(\mathrm{pH} 7\), and \(\mathrm{pH} 9\)?
- What kinds of interactions would each form participate in?
- What is the predominant form of a carboxylic acid group at \(\mathrm{pH} 5\)? \(\mathrm{pH} 7\), \(\mathrm{pH} 9\)
- What kinds of interactions would each form participate in?
- What is the ratio of \(–\mathrm{NH}_{3}{}^{+} / –\mathrm{NH}_{2}\) in a solution of a protein at \(\mathrm{pH} 5\), \(\mathrm{pH} 7\), and \(\mathrm{pH} 9\)? What kinds of interactions does each form participate in?
- What is the predominant form of a carboxylic acid group at \(\mathrm{pH} 5\)? How about at \(\mathrm{pH} 7\) and \(\mathrm{pH} 9\)? What kinds of interactions does each form participate in?