5.4: Trait-based approaches
- Page ID
- 294565
\( \newcommand{\vecs}[1]{\overset { \scriptstyle \rightharpoonup} {\mathbf{#1}} } \)
\( \newcommand{\vecd}[1]{\overset{-\!-\!\rightharpoonup}{\vphantom{a}\smash {#1}}} \)
\( \newcommand{\id}{\mathrm{id}}\) \( \newcommand{\Span}{\mathrm{span}}\)
( \newcommand{\kernel}{\mathrm{null}\,}\) \( \newcommand{\range}{\mathrm{range}\,}\)
\( \newcommand{\RealPart}{\mathrm{Re}}\) \( \newcommand{\ImaginaryPart}{\mathrm{Im}}\)
\( \newcommand{\Argument}{\mathrm{Arg}}\) \( \newcommand{\norm}[1]{\| #1 \|}\)
\( \newcommand{\inner}[2]{\langle #1, #2 \rangle}\)
\( \newcommand{\Span}{\mathrm{span}}\)
\( \newcommand{\id}{\mathrm{id}}\)
\( \newcommand{\Span}{\mathrm{span}}\)
\( \newcommand{\kernel}{\mathrm{null}\,}\)
\( \newcommand{\range}{\mathrm{range}\,}\)
\( \newcommand{\RealPart}{\mathrm{Re}}\)
\( \newcommand{\ImaginaryPart}{\mathrm{Im}}\)
\( \newcommand{\Argument}{\mathrm{Arg}}\)
\( \newcommand{\norm}[1]{\| #1 \|}\)
\( \newcommand{\inner}[2]{\langle #1, #2 \rangle}\)
\( \newcommand{\Span}{\mathrm{span}}\) \( \newcommand{\AA}{\unicode[.8,0]{x212B}}\)
\( \newcommand{\vectorA}[1]{\vec{#1}} % arrow\)
\( \newcommand{\vectorAt}[1]{\vec{\text{#1}}} % arrow\)
\( \newcommand{\vectorB}[1]{\overset { \scriptstyle \rightharpoonup} {\mathbf{#1}} } \)
\( \newcommand{\vectorC}[1]{\textbf{#1}} \)
\( \newcommand{\vectorD}[1]{\overrightarrow{#1}} \)
\( \newcommand{\vectorDt}[1]{\overrightarrow{\text{#1}}} \)
\( \newcommand{\vectE}[1]{\overset{-\!-\!\rightharpoonup}{\vphantom{a}\smash{\mathbf {#1}}}} \)
\( \newcommand{\vecs}[1]{\overset { \scriptstyle \rightharpoonup} {\mathbf{#1}} } \)
\( \newcommand{\vecd}[1]{\overset{-\!-\!\rightharpoonup}{\vphantom{a}\smash {#1}}} \)
\(\newcommand{\avec}{\mathbf a}\) \(\newcommand{\bvec}{\mathbf b}\) \(\newcommand{\cvec}{\mathbf c}\) \(\newcommand{\dvec}{\mathbf d}\) \(\newcommand{\dtil}{\widetilde{\mathbf d}}\) \(\newcommand{\evec}{\mathbf e}\) \(\newcommand{\fvec}{\mathbf f}\) \(\newcommand{\nvec}{\mathbf n}\) \(\newcommand{\pvec}{\mathbf p}\) \(\newcommand{\qvec}{\mathbf q}\) \(\newcommand{\svec}{\mathbf s}\) \(\newcommand{\tvec}{\mathbf t}\) \(\newcommand{\uvec}{\mathbf u}\) \(\newcommand{\vvec}{\mathbf v}\) \(\newcommand{\wvec}{\mathbf w}\) \(\newcommand{\xvec}{\mathbf x}\) \(\newcommand{\yvec}{\mathbf y}\) \(\newcommand{\zvec}{\mathbf z}\) \(\newcommand{\rvec}{\mathbf r}\) \(\newcommand{\mvec}{\mathbf m}\) \(\newcommand{\zerovec}{\mathbf 0}\) \(\newcommand{\onevec}{\mathbf 1}\) \(\newcommand{\real}{\mathbb R}\) \(\newcommand{\twovec}[2]{\left[\begin{array}{r}#1 \\ #2 \end{array}\right]}\) \(\newcommand{\ctwovec}[2]{\left[\begin{array}{c}#1 \\ #2 \end{array}\right]}\) \(\newcommand{\threevec}[3]{\left[\begin{array}{r}#1 \\ #2 \\ #3 \end{array}\right]}\) \(\newcommand{\cthreevec}[3]{\left[\begin{array}{c}#1 \\ #2 \\ #3 \end{array}\right]}\) \(\newcommand{\fourvec}[4]{\left[\begin{array}{r}#1 \\ #2 \\ #3 \\ #4 \end{array}\right]}\) \(\newcommand{\cfourvec}[4]{\left[\begin{array}{c}#1 \\ #2 \\ #3 \\ #4 \end{array}\right]}\) \(\newcommand{\fivevec}[5]{\left[\begin{array}{r}#1 \\ #2 \\ #3 \\ #4 \\ #5 \\ \end{array}\right]}\) \(\newcommand{\cfivevec}[5]{\left[\begin{array}{c}#1 \\ #2 \\ #3 \\ #4 \\ #5 \\ \end{array}\right]}\) \(\newcommand{\mattwo}[4]{\left[\begin{array}{rr}#1 \amp #2 \\ #3 \amp #4 \\ \end{array}\right]}\) \(\newcommand{\laspan}[1]{\text{Span}\{#1\}}\) \(\newcommand{\bcal}{\cal B}\) \(\newcommand{\ccal}{\cal C}\) \(\newcommand{\scal}{\cal S}\) \(\newcommand{\wcal}{\cal W}\) \(\newcommand{\ecal}{\cal E}\) \(\newcommand{\coords}[2]{\left\{#1\right\}_{#2}}\) \(\newcommand{\gray}[1]{\color{gray}{#1}}\) \(\newcommand{\lgray}[1]{\color{lightgray}{#1}}\) \(\newcommand{\rank}{\operatorname{rank}}\) \(\newcommand{\row}{\text{Row}}\) \(\newcommand{\col}{\text{Col}}\) \(\renewcommand{\row}{\text{Row}}\) \(\newcommand{\nul}{\text{Nul}}\) \(\newcommand{\var}{\text{Var}}\) \(\newcommand{\corr}{\text{corr}}\) \(\newcommand{\len}[1]{\left|#1\right|}\) \(\newcommand{\bbar}{\overline{\bvec}}\) \(\newcommand{\bhat}{\widehat{\bvec}}\) \(\newcommand{\bperp}{\bvec^\perp}\) \(\newcommand{\xhat}{\widehat{\xvec}}\) \(\newcommand{\vhat}{\widehat{\vvec}}\) \(\newcommand{\uhat}{\widehat{\uvec}}\) \(\newcommand{\what}{\widehat{\wvec}}\) \(\newcommand{\Sighat}{\widehat{\Sigma}}\) \(\newcommand{\lt}{<}\) \(\newcommand{\gt}{>}\) \(\newcommand{\amp}{&}\) \(\definecolor{fillinmathshade}{gray}{0.9}\)5.4. Trait-based approaches
Author: Paul J. Van den Brink
Reviewers: Nico van den Brink, Michiel Kraak, Alexa Alexander-Trusiak
Learning objectives:
You should be able to
- describe how the characteristics (traits) of species determine their sensitivity, recovery and the propagation of effects to higher levels of biological organisation.
- explain the concept of response and effect traits.
- explain how traits-based approaches can be implemented into environmental risk assessment.
Keywords: Sensitivity, levels of biological organisation, species traits, recovery, indirect effects
Introduction
It is impossible to assess the sensitivity of all species to all chemicals. Risk assessments therefore, need methods to extrapolate the sensitivity of a limited number of species to all species present in the environment is desired. Statistical approaches, like the species sensitivity distribution concept, perform this extrapolation by fitting a statistical distribution (e.g. log-normal distribution) to a selected set of sensitivity data (e.g. 96h-EC50 data) in order to obtain a distribution of the sensitivity of all species. From this distribution a threshold value associated with the lower end of the distribution can be chosen and used as a protective threshold value (Figure 1).
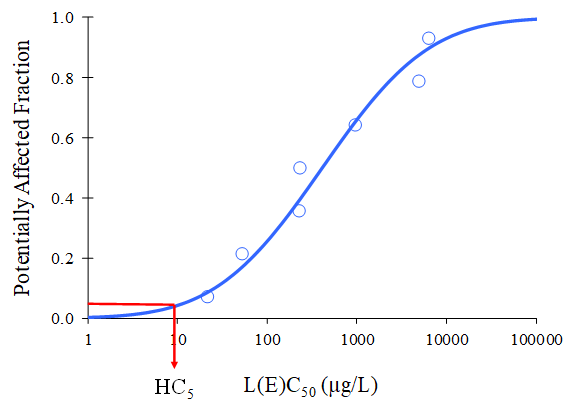
The disadvantage of this approach is that it does not include mechanistic knowledge on what determines species' sensitivity and uses species taxonomy rather than their characteristics. To overcome these and other problems associated with a taxonomy based approach (see Van den Brink et al., 2011 for a review) traits-based bioassessment approaches have been developed for assessing the effects of chemicals on aquatic ecosystem. In traits-based bioassessment approaches, species are not represented by their taxonomy but by their traits. A trait is a phenotypic or ecological characteristic of an organism, usually measured at the individual level but often applied as the average state/condition of a species. Examples of traits are body size, feeding habits, food preference, mode of respiration and lipid content. Traits describe the physical characteristics, ecological niche, and functional role of a species within the ecosystem. The recognized strengths of traits-based bioassessment approaches include: (1) traits add mechanistic and diagnostic knowledge, (2) traits are transferrable across geographies, (3) traits require no new sampling methodology as data that are currently collected can be used, (4) the use of traits has a long-standing tradition in ecology and can supplement taxonomic analysis.
When traits are used to study effects of chemical stressors on ecosystem structure (community composition) and function (e.g. nutrient cycling) it is important to make a distinction between response and effects traits (Figure 2). Response traits are traits that enable a response of the species to the exposure to a chemical. An example of a response trait may be size related surface area of an organism. Smaller organisms have relatively large surface areas, because their surface to volume ratio is higher than for larger animals Herewith, the uptake rate of the chemical stressor is generally higher in smaller animals compared to larger ones (Rubach et al., 2012). Effects traits of organisms influence the surrounding environment by the organisms, by altering the structure and functioning of the ecosystem. An example of an effect trait is the food preference of an organism. For instance, if the small (response trait) and herewith sensitive organisms happen to be herbivorous (effect trait) an increase in algal biomass may be expected when the organisms are affected (Van den Brink, 2008). So, to be able to predict ecosystem level responses it is important to know the (cor)relations between response and effect traits as traits are not independent from each other but can be linked phylogenetically or mechanistically and thus form trait syndromes (Van den Brink et al., 2011).
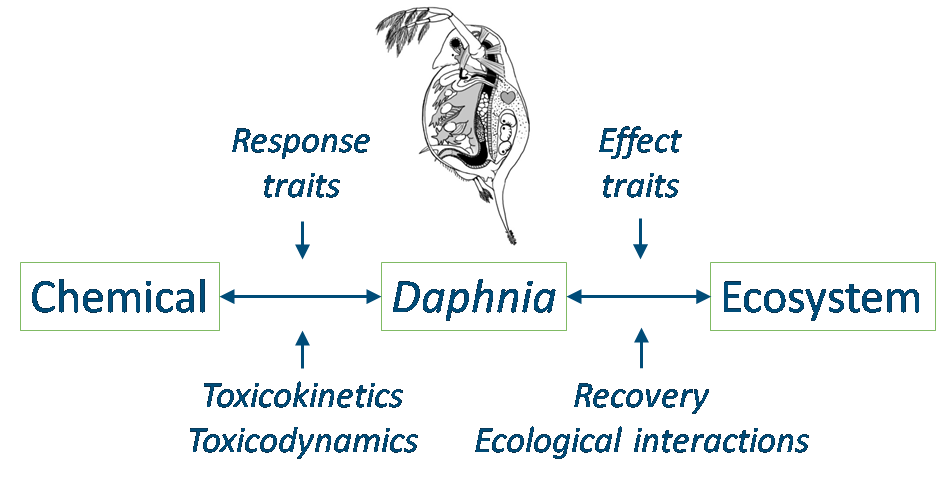
Predictive models for sensitivity using response traits
One of the holy grails of ecotoxicology is to find out which species traits make one species more sensitive to a chemical stressor than another one. In the past, two approaches have been used to assess the (cor)relationships between species traits and their sensitivity, one based on empirical correlations between species' traits and their sensitivity as represented by EC50's (Rico and Van den Brink, 2015) and one based on a more mechanistic approach using toxicokinetic/toxicodynamic experiments and models (Rubach et al., 2012). Toxicokinetic-toxicodynamic models (TKTD models) simulate the time-course of processes leading to toxic effects on organisms (Jager et al., 2011). Toxicokinetics describe what an individual does with the chemical and, in their simplest form, include the processes of uptake and elimination, thereby translating an external concentration of a toxicant to an internal body concentration over time (see Section on Toxicokinetics and Bioaccumulation). Toxicodynamics describes what the chemical does with the organism, herewith linking the internal concentration to the effect at the level of the individual organism over time (e.g., mortality) (Jager et al., 2011) (see Sections on Toxicokinetics and Bioaccumulation and on Toxicodynamics and Molecular Interactions). Rubach et al. (2012) showed that almost 90% of the variation in uptake rates and 80% of the variation in elimination rates of an insecticide in a range of 15 freshwater arthropod species could be explained by 4 species traits. These traits were: i) surface area (without gills), ii) detritivorous feeding, iii) using atmospheric oxygen and iv) phylogeny in case of uptake, and i) thickness of exoskeleton, ii) complete sclerotization, iii) using dissolved oxygen and iv) % lipid of dry weight in case of elimination. For most of these traits, a mechanistic hypothesis between the traits and their influence on the uptake and elimination can be made (Rubach et al., 2012). For instance, a higher surface area to volume ratio increases the uptake of the chemical, so uptake is expected to be higher in small animals compared to larger animals. This shows that it is possible to construct mechanistic models that are able to predict the toxicokinetics of chemicals in species and herewith the sensitivity of species to chemicals based on their traits.
The use of effect traits to model recovery and indirect effects
Traits determining the way organisms within an ecosystem react to chemical stress are related to the intrinsic sensitivity of the organisms on the one hand (response traits) and their recovery potential and food web relations (effect traits) on the other hand (Van den Brink, 2008). Recovery of aquatic invertebrates is, for instance, determined by traits like number of life cycles per year, the presence of insensitive life stages like resting eggs, dispersal ability and having an aerial life stage (Gergs et al., 2011) (Figure 3).
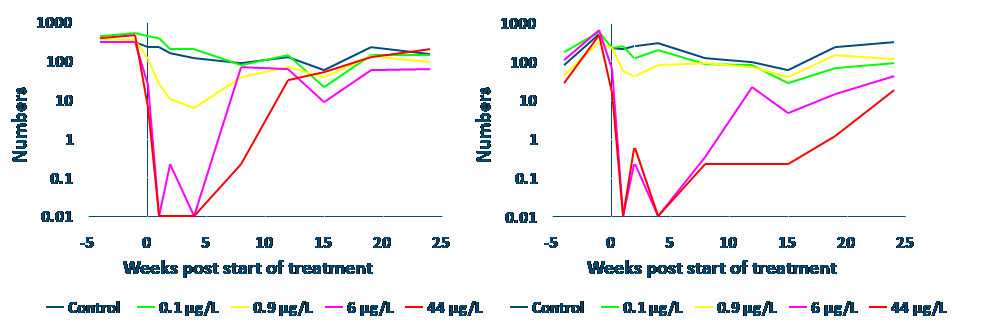
Besides recovery, effect traits will also determine how individual level effects will propagate to higher levels of biological organisation like the community or ecosystem level. For instance, when Daphnia are affected by a chemical, their trait related to food preference (algae) will ensure that, under nutrient-rich conditions, the algae will not be subjected to top-down control and will increase in abundance. The latter effects are called indirect effects, which are not a direct result of the exposure to the toxicant but an indirect one through competition, food-web relationships, etc..
References
Gergs, A., Classen, S., Hommen, U. (2011). Identification of realistic worst case aquatic macroinvertebrate species for prospective risk assessment using the trait concept. Environmental Science and Pollution Research 18, 1316-1323.
Jager, T., Albert, C., Preuss, T.G., Ashauer, R. (2011). General unified threshold model of survival-A toxicokinetic-toxicodynamic framework for ecotoxicology. Environmental Science and Technology 45, 2529-2540
Rico, A., Van den Brink, P.J. (2015). Evaluating aquatic invertebrate vulnerability to insecticides based on intrinsic sensitivity, biological traits and toxic mode-of-action. Environmental Toxicology and Chemistry 34, 1907-1917.
Rubach, M.N., D.J. Baird, M-C. Boerwinkel, S.J. Maund, I. Roessink, Van den Brink P.J. (2012). Species traits as predictors for intrinsic sensitivity of aquatic invertebrates to the insecticide chlorpyrifos. Ecotoxicology 21, 2088-2101.
Van den Brink, P.J. (2008). Ecological risk assessment: from book-keeping to chemical stress ecology. Environmental Science and Technology 42, 8999-9004.
Van den Brink P.J., Alexander, A., Desrosiers, M., Goedkoop, W., Goethals, P., Liess, M., Dyer, S. (2011). Traits-based approaches in bioassessment and ecological risk assessment: strengths, weaknesses, opportunities and threats. Integrated Environmental Assessment and Management 7, 198-208.
Which two traits may determine the sensitivity of invertebrates to insecticides?
Will two daphnids which are equal except in their size be equally sensitive to a chemical?
What is the difference between a response and an effect trait?
Which traits are of importance for the recovery of impacted populations?
How will the insecticide-induced death of water fleas propagate to the ecosystem level under eutrophic circumstances, and what are these types of effects called?