10: Removal of Lead
- Page ID
- 40259
\( \newcommand{\vecs}[1]{\overset { \scriptstyle \rightharpoonup} {\mathbf{#1}} } \)
\( \newcommand{\vecd}[1]{\overset{-\!-\!\rightharpoonup}{\vphantom{a}\smash {#1}}} \)
\( \newcommand{\id}{\mathrm{id}}\) \( \newcommand{\Span}{\mathrm{span}}\)
( \newcommand{\kernel}{\mathrm{null}\,}\) \( \newcommand{\range}{\mathrm{range}\,}\)
\( \newcommand{\RealPart}{\mathrm{Re}}\) \( \newcommand{\ImaginaryPart}{\mathrm{Im}}\)
\( \newcommand{\Argument}{\mathrm{Arg}}\) \( \newcommand{\norm}[1]{\| #1 \|}\)
\( \newcommand{\inner}[2]{\langle #1, #2 \rangle}\)
\( \newcommand{\Span}{\mathrm{span}}\)
\( \newcommand{\id}{\mathrm{id}}\)
\( \newcommand{\Span}{\mathrm{span}}\)
\( \newcommand{\kernel}{\mathrm{null}\,}\)
\( \newcommand{\range}{\mathrm{range}\,}\)
\( \newcommand{\RealPart}{\mathrm{Re}}\)
\( \newcommand{\ImaginaryPart}{\mathrm{Im}}\)
\( \newcommand{\Argument}{\mathrm{Arg}}\)
\( \newcommand{\norm}[1]{\| #1 \|}\)
\( \newcommand{\inner}[2]{\langle #1, #2 \rangle}\)
\( \newcommand{\Span}{\mathrm{span}}\) \( \newcommand{\AA}{\unicode[.8,0]{x212B}}\)
\( \newcommand{\vectorA}[1]{\vec{#1}} % arrow\)
\( \newcommand{\vectorAt}[1]{\vec{\text{#1}}} % arrow\)
\( \newcommand{\vectorB}[1]{\overset { \scriptstyle \rightharpoonup} {\mathbf{#1}} } \)
\( \newcommand{\vectorC}[1]{\textbf{#1}} \)
\( \newcommand{\vectorD}[1]{\overrightarrow{#1}} \)
\( \newcommand{\vectorDt}[1]{\overrightarrow{\text{#1}}} \)
\( \newcommand{\vectE}[1]{\overset{-\!-\!\rightharpoonup}{\vphantom{a}\smash{\mathbf {#1}}}} \)
\( \newcommand{\vecs}[1]{\overset { \scriptstyle \rightharpoonup} {\mathbf{#1}} } \)
\( \newcommand{\vecd}[1]{\overset{-\!-\!\rightharpoonup}{\vphantom{a}\smash {#1}}} \)
\(\newcommand{\avec}{\mathbf a}\) \(\newcommand{\bvec}{\mathbf b}\) \(\newcommand{\cvec}{\mathbf c}\) \(\newcommand{\dvec}{\mathbf d}\) \(\newcommand{\dtil}{\widetilde{\mathbf d}}\) \(\newcommand{\evec}{\mathbf e}\) \(\newcommand{\fvec}{\mathbf f}\) \(\newcommand{\nvec}{\mathbf n}\) \(\newcommand{\pvec}{\mathbf p}\) \(\newcommand{\qvec}{\mathbf q}\) \(\newcommand{\svec}{\mathbf s}\) \(\newcommand{\tvec}{\mathbf t}\) \(\newcommand{\uvec}{\mathbf u}\) \(\newcommand{\vvec}{\mathbf v}\) \(\newcommand{\wvec}{\mathbf w}\) \(\newcommand{\xvec}{\mathbf x}\) \(\newcommand{\yvec}{\mathbf y}\) \(\newcommand{\zvec}{\mathbf z}\) \(\newcommand{\rvec}{\mathbf r}\) \(\newcommand{\mvec}{\mathbf m}\) \(\newcommand{\zerovec}{\mathbf 0}\) \(\newcommand{\onevec}{\mathbf 1}\) \(\newcommand{\real}{\mathbb R}\) \(\newcommand{\twovec}[2]{\left[\begin{array}{r}#1 \\ #2 \end{array}\right]}\) \(\newcommand{\ctwovec}[2]{\left[\begin{array}{c}#1 \\ #2 \end{array}\right]}\) \(\newcommand{\threevec}[3]{\left[\begin{array}{r}#1 \\ #2 \\ #3 \end{array}\right]}\) \(\newcommand{\cthreevec}[3]{\left[\begin{array}{c}#1 \\ #2 \\ #3 \end{array}\right]}\) \(\newcommand{\fourvec}[4]{\left[\begin{array}{r}#1 \\ #2 \\ #3 \\ #4 \end{array}\right]}\) \(\newcommand{\cfourvec}[4]{\left[\begin{array}{c}#1 \\ #2 \\ #3 \\ #4 \end{array}\right]}\) \(\newcommand{\fivevec}[5]{\left[\begin{array}{r}#1 \\ #2 \\ #3 \\ #4 \\ #5 \\ \end{array}\right]}\) \(\newcommand{\cfivevec}[5]{\left[\begin{array}{c}#1 \\ #2 \\ #3 \\ #4 \\ #5 \\ \end{array}\right]}\) \(\newcommand{\mattwo}[4]{\left[\begin{array}{rr}#1 \amp #2 \\ #3 \amp #4 \\ \end{array}\right]}\) \(\newcommand{\laspan}[1]{\text{Span}\{#1\}}\) \(\newcommand{\bcal}{\cal B}\) \(\newcommand{\ccal}{\cal C}\) \(\newcommand{\scal}{\cal S}\) \(\newcommand{\wcal}{\cal W}\) \(\newcommand{\ecal}{\cal E}\) \(\newcommand{\coords}[2]{\left\{#1\right\}_{#2}}\) \(\newcommand{\gray}[1]{\color{gray}{#1}}\) \(\newcommand{\lgray}[1]{\color{lightgray}{#1}}\) \(\newcommand{\rank}{\operatorname{rank}}\) \(\newcommand{\row}{\text{Row}}\) \(\newcommand{\col}{\text{Col}}\) \(\renewcommand{\row}{\text{Row}}\) \(\newcommand{\nul}{\text{Nul}}\) \(\newcommand{\var}{\text{Var}}\) \(\newcommand{\corr}{\text{corr}}\) \(\newcommand{\len}[1]{\left|#1\right|}\) \(\newcommand{\bbar}{\overline{\bvec}}\) \(\newcommand{\bhat}{\widehat{\bvec}}\) \(\newcommand{\bperp}{\bvec^\perp}\) \(\newcommand{\xhat}{\widehat{\xvec}}\) \(\newcommand{\vhat}{\widehat{\vvec}}\) \(\newcommand{\uhat}{\widehat{\uvec}}\) \(\newcommand{\what}{\widehat{\wvec}}\) \(\newcommand{\Sighat}{\widehat{\Sigma}}\) \(\newcommand{\lt}{<}\) \(\newcommand{\gt}{>}\) \(\newcommand{\amp}{&}\) \(\definecolor{fillinmathshade}{gray}{0.9}\)In chemistry we relate macroscopic, observable change to transformations at the molecular level that go unseen through the shorthand of symbolic representation. For the most part, we are comforted by the fact that we can at least observe the macroscopic. Here is a wonderful representation of the way chemical reactions take place that may help you understand chemical kinetics and thermodynamics.
Although tetraethyllead left its own toxic trail, the human toxicity of lead compounds was not the driving force for the removal of TEL from fuels. Eventually TEL was removed to make way for the catalytic converter in an effort to reduce the damage from nitrogen oxides and ozone. This is a discussion of the \(NO_x\) and Tropospheric Ozone (\(O_3\)) problem. To understand this section fully, you need to be familiar with the principles of chemical kinetics, chemical equilibrium, thermochemistry and thermodynamics described in your chemistry text. This section amplifies on those principles and applies them to the design, manufacture and use of fuels and engines.
Consider the chemical reactions:
\[ \ce{N_2 + O_2 \rightarrow 2NO} \nonumber \]
\[ \ce{ 3/2 O_2 \rightarrow O_3} \nonumber \]
Macroscopic Observations of the Air Around Us:
- Does the chemical reaction between nitrogen and oxygen to give nitric oxide (\(NO\)) take place at room temperature and pressure to any great extent?
- Does oxygen convert to ozone (\(O_3\))?
What is your intuitive answer? What is the evidence that it does not? Is it important, for example that the earth's atmosphere consists of 79% nitrogen and 20% oxygen and virtually no ozone and the two substances appear to coexist without undergoing any spontaneous chemical reaction?
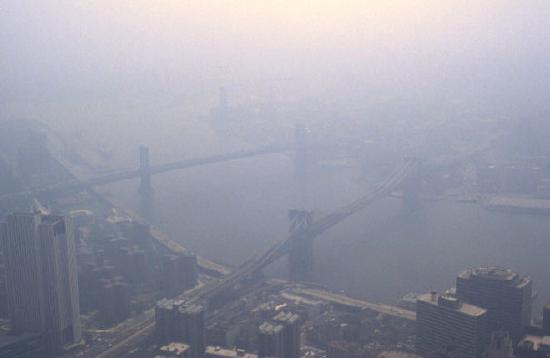
But we do see, hovering over our cities a brown haze we call photochemical smog. We can measure the gaseous impurities - we find ozone there. We also find nitrogen oxides (\(NO_x\)) and that they are at higher concentrations in the daytime than at night. It turns out this photochemical smog is a result of the production of nitric oxide in the confined and extraordinary conditions of the automobile cylinder or in power plants at a temperature above 2000 degrees Celsius. Under these conditions nitric oxide (\(NO\)) is formed and is emitted to the atmosphere, there to begin the process of smog formation through the production of ozone.
Microscopic Evaluation
Nitric oxide molecules form a colorless gas, but the substance is highly reactive in the presence of oxygen. In the atmosphere, \(NO\) molecules react with oxygen rapidly to form \(NO_2\). \(NO_2\) production starts a cascade of chemical reactions that produces the oxygen allotrope, ozone (\(O_3\)). Ozone is harmful in the lower atmosphere. More complex reactions of ozone cause photochemical smog.
Look at this sequence of chemical reactions:
\[\ce{NO} + \ce{1/2O_2} \rightarrow \underbrace{\ce{1/2} NO_2}_{\text{brown gas}} \nonumber \]
\[\ce{ 1/2 NO_2 ->[ h\nu] NO + O} \nonumber \]
\[\ce{O + O_2 \rightarrow O_3} \nonumber \]
The brown gas, nitrogen dioxide decays back to \(NO\) and an oxygen atom and the oxygen atom reacts with an oxygen molecule to form ozone. Take a moment to sum the chemical reactions just as you would partial algebraic equations:
\[\ce{3/2 O_2 \rightarrow O_3} \nonumber \]
Note that:
- NO, NO2 , and the oxygen atom do not appear in the final summation. This means there is not net increase or decrease in their concentrations - they are produced in one step and disappear in another.
- This sequence is called a chain reaction and it results in the conversion of oxygen to ozone.
- The conversion of oxygen to ozone doesn't occur in normal atmospheric conditions
Thermodynamics and the Production of NO
As in the cracking of hexane to propane and propene that we discussed earlier, thermodynamic principle explain to us the production of NO.
delta H0 kcal.mol | S0 cal/mol*K | |
NO | 22 | +50 |
N2 | 0 | +46 |
O2 | 0 | +49 |
\[N_2 + O_2 \rightarrow 2NO \nonumber \]
And for the reaction at room temperature - 298 degrees Celsius:
\[\Delta G = \Delta H - T \Delta S \nonumber \]
at standard temperature:
\[\Delta G^o = 44\,kcal - (298\,K)(5\,cal/K) = +42\,kcal \nonumber \]
The process is not spontaneous. Even if we look at a temperature of 2400 degrees Celsius, delta G0 is = 32kcal so the process is not spontaneous.
So how does NO occur?
Let us look at the equation relationg G,H and S. It derives the properties at standard state, that is, for gases, a pressure of 1 atmosphere for each component and a temperature of 298 degrees Celsius. But we are far from standard state. How can the equation be modified to reflect deviations from standard, the real condition of generation of small concentrations of products in a process that is non spontaneous at standard conditions?
At equilibrium, that is at the point where forward and reverse reactions are taking place at the same rate:
\[\Delta G = \Delta G^o + RT \ln (Q) \nonumber \]
in which
- T = temperature Celsius
- R = gas constant
- Q = ratio of concentrations of products to starting materials
In this expression, if the system is at equilibrium, delta G = 0 and the ratio Q = Keq the equilibrium constant. This equation implies that in ANY chemical process that is at equilibrium, SOME of all species will be present.
But in the case of the production of NO, the equilibrium constant determined by the ratios at equilibrium is 10-15 at 298 degrees Celsius. This implies a tiny concentration of NO, but remember,each process must be activated to the transition state to proceed to products. And at the low temperature, the reaction rate to give NO is negligible because of high activation energy required.
At 2400 degrees Celsius, however, the Keq = 0.05 and coupled with the ease of reaching the transition state, NO is formed. The gas mixture quickly cools and the composition is frozen and an engine exhausts as much as 4g/mile of NO to the atmosphere.
Chemical Kinetics, Catalysis and the Production of Ozone:
If we look at the sequence of ozone production again:
NO + 1/2O2 --> 1/2NO2(brown gas)
sunlight
1/2NO2 -------------> NO + O
O + O2 -----> O3
we see a chain reaction in which the presence of three components are necessary to convert oxygen to ozone. Both NO and NO2 are formed and destroyed in the process. Their concentration does not change at equilibrium yet they are essential to the process. They are each catalystss - substances that effectively reduce the transition state requirements for a process to go forward.
Sunlight is a catalyst also - and a catalyst without which the process does not go forward. The decomposition of NO2 to NO and O requires sunlight and indeed the smog forming processes abate at night as determineed by measurements of the airborne pollutants.
1. You will recognize that ozone in the upper atmosphere is necessary. The destruction of the ozone layer by refrigerant gases has been slowed by development of new refrigerants stemming from application of chemical concepts.