Objectives
After completing this section, you should be able to
- draw the structure of furan, pyrrole and imidazole.
- use the Hückel 2n + 4 rule to explain the aromaticity of pyrrole.
- predict the product formed when pyrrole is subjected to an aromatic electrophilic substitution, such as nitration, etc.
- write the detailed mechanism for the electrophilic aromatic substitution of pyrrole to account for the fact that substitution takes place at C2 rather than C3.
- describe the geometry of the pyridine molecule.
- account for the difference in basicity between pyridine, pyrrole and other amines.
- explain why pyridine undergoes electrophilic substitution much less readily than does benzene.
- identify the presence of a fused‑ring heterocycle in a given structure.
- make predictions about the chemical behaviour of the fused‑ring heterocycle based on what you have learned about pyrrole, imidazole, pyridine and pyrimidine.
Heterocyclic structures containing nitrogen are found in many natural products. Examples of some nitrogen compounds, known as alkaloids because of their basic properties, were given in the amine chapter.
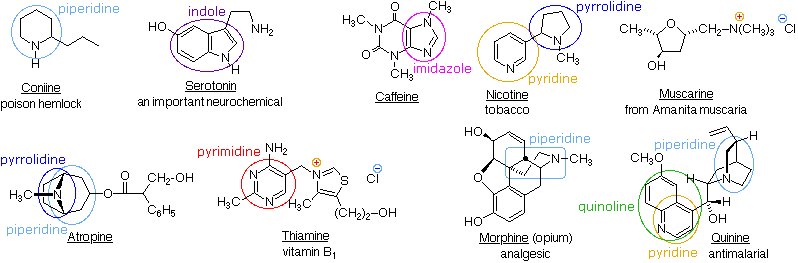
The porphyrin aromatic heterocycle contains multiple pyrrole ring strictures. The porphyrines, heme and chlorophyll b play vital parts in the metabolism of plants and animals.
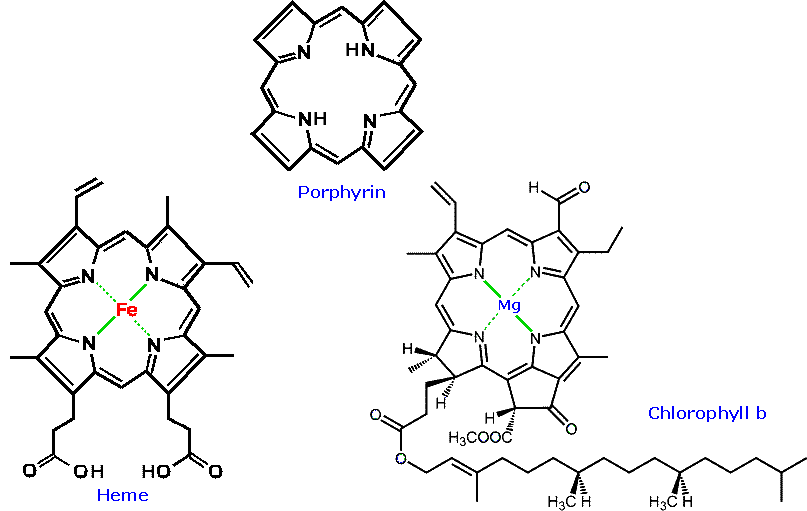
Pyrrole
Pyrrole is obtained commercially by the reaction of furan with ammonia .

As discussed in Section 15-5, pyrrole has six pi electrons contributing to its aromaticity. Each carbon in pyrrole contributes one pi electron. The nitrogen in pyrrole contributes two pi electron by becoming sp2 hybridized and placing its lone pair electrons into a p orbital. Because the nitrogen lone pair is part of the aromatic sextet, the electrons are very stable and are much less available for bonding to a proton (and if they do pick up a proton, the aromatic system is destroyed). For these reasons, pyrrole nitrogens are not strongly basic. Pyrrole is a very weak base: the conjugate acid is a strong acid with a pKa of 0.4.
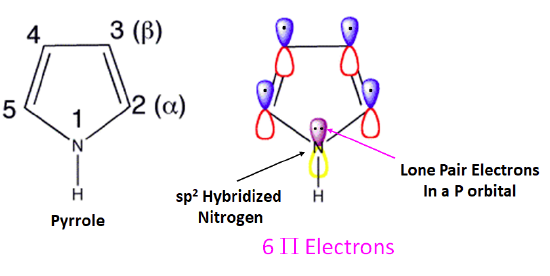
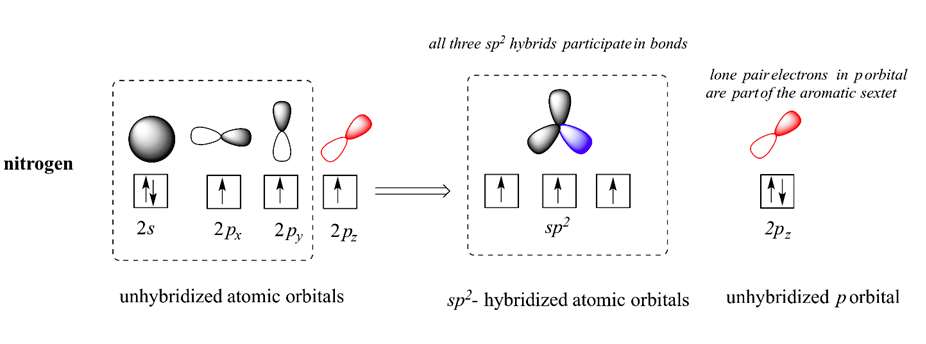

The involvement of the nitrogen lone pair electrons in pyrrole's aromatic conjugation creates charge separated structures not normally found for benzene. The combination of these resonance forms creates an overall separation of charge which increases the electron density around the ring carbons and decreases the electron density on the nitrogen. This effect has a number of ramifications. First is that pyrrole has a large dipole moment (1.8 D) pointing away from the ring nitrogen. This is in sharp contrast to the non-aromatic heterocycle pyrrolidine where the dipole moment (1.5 D) points towards the ring nitrogen.

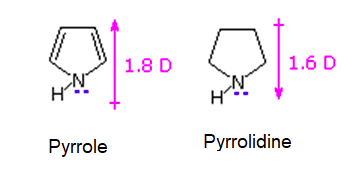
Due to the resonance forms, pyrrole has less electron density around it than the nitrogen in a typical alkyl amine. This agrees with fact that that pyrrole is a much weaker base than pyrrolidine. The electrostatic potential map of pyrrole (Shown Below) shows less electron density (shown as a red color) on the ring nitrogen when compared to its aliphatic equivalent, pyrrolidine. The map of pyrrole also shows that the resonance forms increase the electron density of pyrrole's ring carbons when compared to the ring carbons of benzene. The involvement of nitrogen's lone pair electrons in the aromaticity of pyrrole makes the ring activated towards electrophilic aromatic substitution.
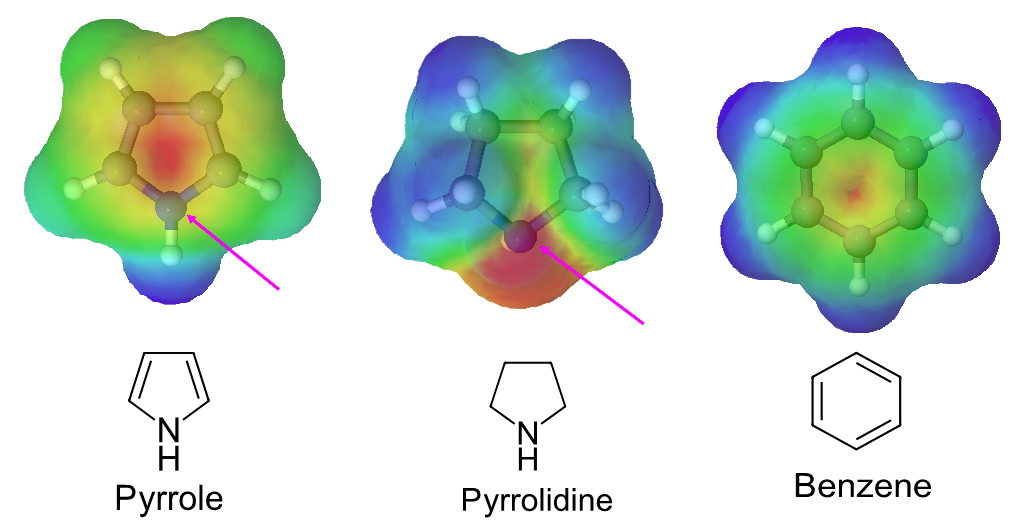
Pyrrole can undergo many of the same electrophilic aromatic substitution reactions as benzene. Because of the activation of pyrrole's aromatic ring, many of these reactions are performed under a reduced temperature compared to a similar reaction with benzene. There is a clear preference for substitution at the 2-position (α) of the pyrrole ring. An explanation for the general α-selectivity of these substitution reactions is apparent from the mechanism outlined below. The intermediate formed by electrophile attack at C-2 is stabilized by charge delocalization to a greater degree than the intermediate from C-3 attack. From the Hammond postulate we may then infer that the activation energy for substitution at the former position is less than the latter substitution.
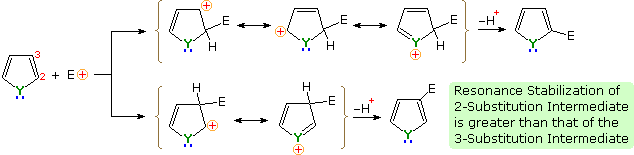
Example

Pyridine
Pyridine is an example of a six-membered aromatic heterocycle. In the bonding picture for pyridine, the nitrogen is sp2-hybridized, with two of the three sp2 orbitals forming sigma overlaps with the sp2 orbitals of neighboring carbon atoms, and the third nitrogen sp2 orbital containing the lone pair. The unhybridized p orbital contains a single electron, which is part of the 6 pi-electron aromatic system delocalized around the ring.
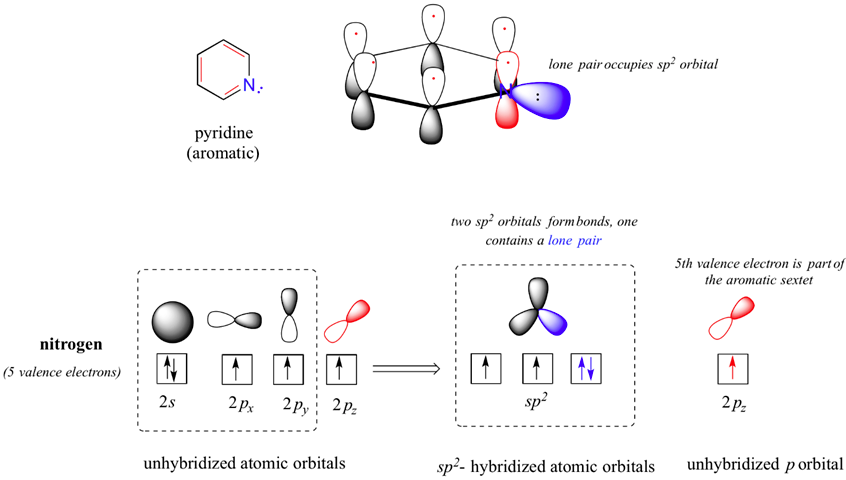
Pyridine's nitrogen lone pair occupies an sp2-hybrid orbital, and is not part of the aromatic sextet. Thus, its electron pair is available for forming a bond to a proton, making pyridine's nitrogen atom (conjugate acid pKa = 5.25) more basic than pyrrole's (pKa = 0.4). However, pyridine is is less basic than a typical alkylamine (pKa ~ 10-11). The difference is due to nitrogen hybridization. The lone pair electrons on a pyridine nitrogen occupy an sp2 hybrid orbital, while the lone pair electrons on an amine nitrogen occupy an sp3 hybrid orbital. sp2 orbitals are composed of one part s and two parts p atomic orbitals, meaning that they have about 33% s character. sp3 orbitals, conversely, are only 25% s character (one part s, three parts p) . An s atomic orbital holds electrons closer to the nucleus than a p orbital, thus s orbitals are more electronegative than p orbitals. Therefore, sp2 hybrid orbitals, with their higher s-character, are more electronegative than sp3 hybrid orbitals. Lone pair electrons in the more electronegative sp2 hybrid orbitals of pyridine are held more tightly to the nitrogen nucleus, and are therefore less 'free' to break away and form a bond to a proton - in other words, they are less basic. sp3

The lack of involvement of pyrrole's nitrogen lone pair electrons in the aromatic conjugation creates charge separated structures not normally found for benzene. The combination of the resonance forms creates an overall charge separation which increases the electron density around the ring's nitrogen and decreases the electron density on the ring carbons. This causes pyridine to have a larger dipole moment (2.26 D) than its non-aromatic equivalent piperidine where the dipole moment (1.17 D). In both cases the dipole moment points towards the ring nitrogen.

Due to the resonance forms, pyridine has more electron density around its nitrogen than in a typical alkyl amine. The electrostatic potential map of pyridine (Shown Below) shows more electron density (shown as a red color) on the ring nitrogen of pyridine when compared to pyrrole. The map of pyridine also shows that the resonance forms decrease the electron density of pyridine's ring carbons (Show as a blue/green color) when compared to those of benzene. The involvement of the nitrogen's lone pair electrons in the aromaticity of pyridine makes the ring deactivated towards electrophilic aromatic substitution.
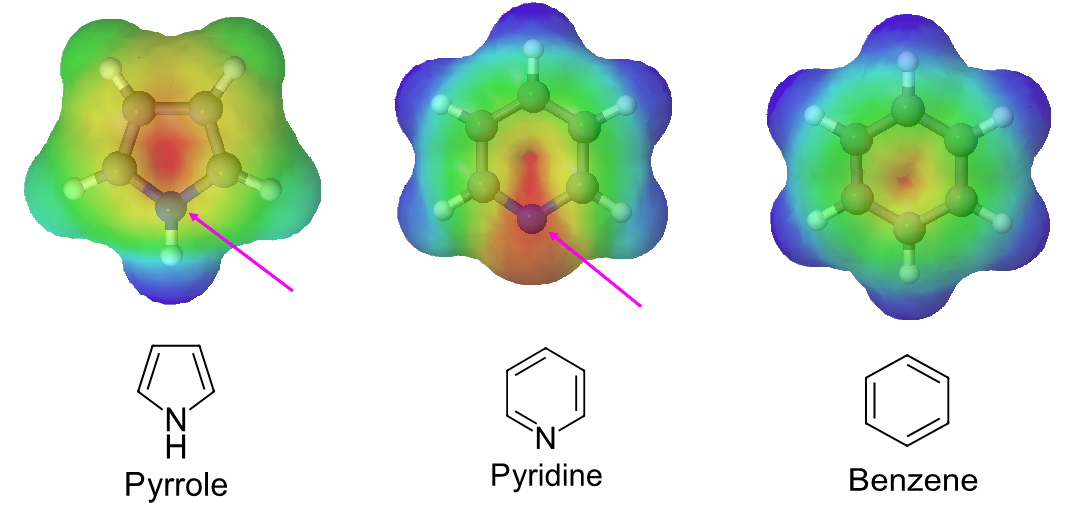
From the previous resonance description of pyridine, we expect this aromatic amine to undergo electrophilic substitution reactions far less easily than does benzene. Furthermore, the electrophilic reagents and catalysts employed in these reactions coordinate with the nitrogen electron pair, exacerbating the positive charge at positions 2,4 & 6 of the pyridine ring. When these reactions do occur then tend to produce the 3-substituted product. Three examples of the extreme conditions required for electrophilic substitution are shown below.
Example

Friedel-Crafts reactions are not feasible because alkyl halides and acid halides prefer to react with pyridine nucleophilic ring nitrogen to provide a N-substituted product. As shown below, N-alkylation and N-acylation products may be prepared as stable crystalline solids in the absence of water or other reactive nucleophiles. Pyridine is a modest base (pKa=5.2). Since the lone pair electrons on pyridine's nitrogen are not part of the aromatic sextet, the pyridinium species produced by N-substitution retains the aromaticity of pyridine.
Example
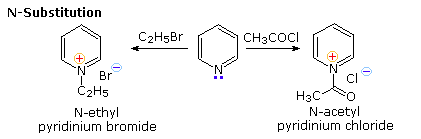
Other six-membered nitrogen contain aromatic heterocycles include pyrazine, pyrimidine, and pyridazine. The inductive effect of a second nitrogen makes all three of these heterocycles less basic than pyridine.
Imidazole
Imidazole is another important example of an aromatic heterocycle found in biomolecules - the side chain of the amino acid histidine contains an imidazole ring. The two nitrogens in imidazole are quite different. One nitrogen is pyrrole-like and donates its lone pair electrons, like pyrrole, to make imidazole aromatic. The other nitrogen is pyridine like and its lone pair electrons are contained is a sp2 hybridized orbital. These lone pair electrons are readily available for bonding which imidazole (pKa = 6.95) much more basic than pyrrole (pKa = 0.4).
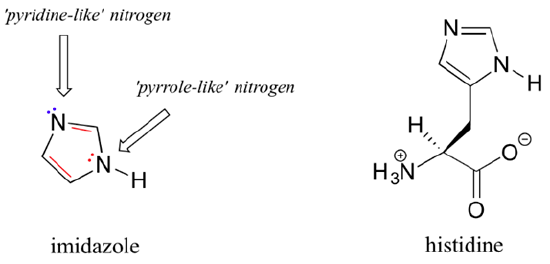
Thiazole
Thiazole is a five-membered sulfur containing aromatic ring system which is found in biological systems, such as thiamine diphosphate. Thiamine diphosphate (ThDP, sometimes also abbreviated TPP or ThPP) is a coenzyme which, like PLP, acts as an electron sink to stabilize key carbanion intermediates. The most important part of the ThDP molecule from a catalytic standpoint is its thiazole ring. The presence of sulfur in thiazole's aromatic ring makes its nitrogen less basic (pKa = 2.44) than imidazole.
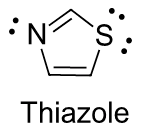

Exercise \(\PageIndex{1}\)
1) Describe how thiazole is aromatic. Use an orbital picture and include the lone pair electrons on sulfur. Assume the sulfur is sp2 hybridized.
2) Would you expect a thiazole ring to be protonated at the physiological pH of 7.3 (Section 24-5)?
- Answer
-
1)
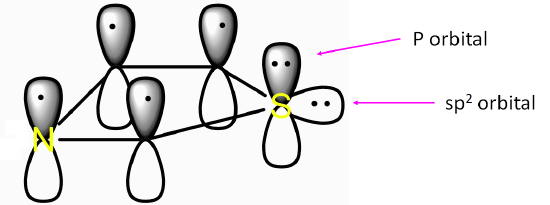
2)
7.3 = 2.44 + log ([RNH2] / [RNH3+])
([RNH2] / [RNH3+]) = 7.2 x 104
. . . so, [RNH2] >> [RNH3+] so thiazole would be almost completely unprotonated at pH = 7.3.
Polycyclic Heterocycles
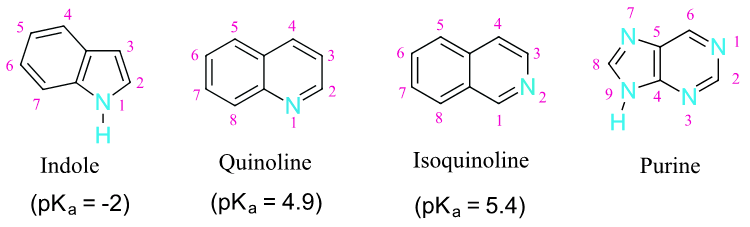
Indole, quinoline, isoquinoline, and purine are all polycyclic aromatic heterocycles commonly found in nature. Indole, quinoline, and isoquinoline all contain a hetrocyclic ring fused to benzene. Purine is made up to two heterocyclic rings, imidazole and pyrimidine, fused together. Quinoline is found in the antimalarial drug quinine. Indole is found in the neurotransmitter serotonin. The purine ring structure is found in adenine and guanine, two important parts of DNA and in the stimulant caffeine.
The pyridine-like nitrogen atom in quinoline and isoquinoline withdraws electrons making them both less reactive to electrophilic substitution than benzene. Likewise, quinoline (pKa = 4.9) and isoquinoline (pKa = 5.4) are less basic that a typical amine (pKa ~10-11). Quinoline and isoquinoline can both undergo electrophilic aromatic substitution but substitution on the pyridine-like ring is avoided. Quinoline usually makes a roughly equal mixture of 5 and 8 substituted products. Isoquinoline favors making the 5 substituted product with a small amount of an 8 substituted side product.
Example
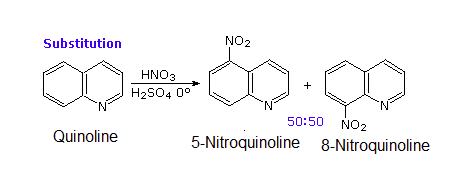
Indole has a ring nitrogen similar to to pyrrole. The lone pair electrons for this nitrogen are contained in a p orbital and are part of indole's 10 pi aromaticity. This makes indole relatively non-basic (pKa = -2) and activated toward electrophilic substitution. Indole undergo electrophilic substitution more easily than benzene and substitution typically occurs at the 3 position on the pyrrole ring.
Example
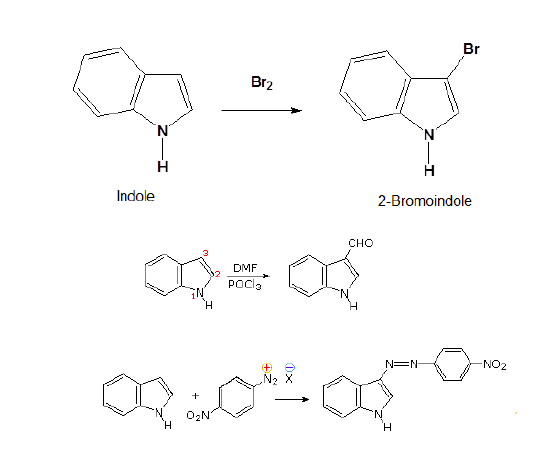
The purine ring system contain three pyridine-like nitrogens. This lone pair electrons of theses nitrogen are contained in sp2 hybrid orbitals, making them not not part of purine's 10 pi aromaticity, allowing them to retain base-like characteristics. The lone pair electrons for the remaining, pyrrole-like, nitrogen are contained in a p orbital and are part of purine's 10 pi aromaticity. This makes the remaining nitrogen relatively non-basic.
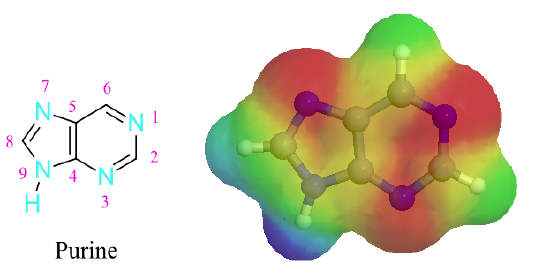
Exercise \(\PageIndex{1}\)
1) Which nitrogen atom in the neurotransmitter serotonin is expected to be the most basic. Please explain your answer.
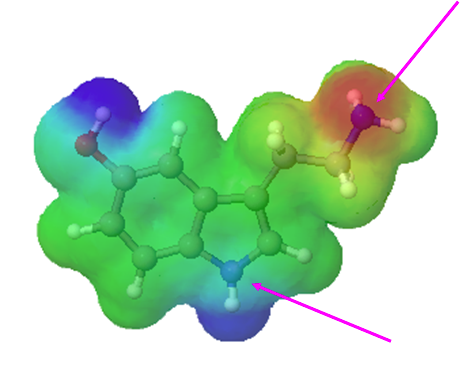
2) Pyridine reacts with electrophiles to product a 3-substituted product rather than a 2-substituted product. Write a series of resonance forms for the cation intermediate formed during the reaction. Use these structures to explain the experimental result.
- Answer
-
1) The
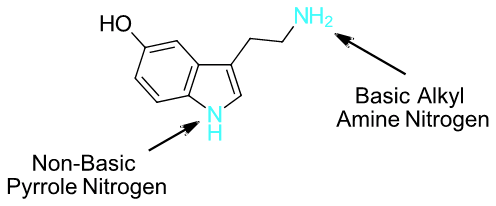
2) The resonance forms of 2-substitution places a positive charge on the ring nitrogen. The resonance forms of 3-substitution do not place a positive charge on the ring nitrogen. Having a positive charge on a nitrogen is less stable than on a carbon. Nitrogen is more electronegative than carbon making it less able to stabilize the positive charge. The 3-substituted product is preferred because it causes resonance forms which are more stable.
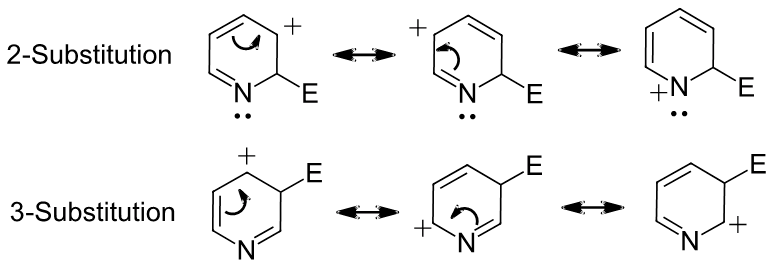