12.5: Nuclear Power
- Page ID
- 393956
\( \newcommand{\vecs}[1]{\overset { \scriptstyle \rightharpoonup} {\mathbf{#1}} } \)
\( \newcommand{\vecd}[1]{\overset{-\!-\!\rightharpoonup}{\vphantom{a}\smash {#1}}} \)
\( \newcommand{\id}{\mathrm{id}}\) \( \newcommand{\Span}{\mathrm{span}}\)
( \newcommand{\kernel}{\mathrm{null}\,}\) \( \newcommand{\range}{\mathrm{range}\,}\)
\( \newcommand{\RealPart}{\mathrm{Re}}\) \( \newcommand{\ImaginaryPart}{\mathrm{Im}}\)
\( \newcommand{\Argument}{\mathrm{Arg}}\) \( \newcommand{\norm}[1]{\| #1 \|}\)
\( \newcommand{\inner}[2]{\langle #1, #2 \rangle}\)
\( \newcommand{\Span}{\mathrm{span}}\)
\( \newcommand{\id}{\mathrm{id}}\)
\( \newcommand{\Span}{\mathrm{span}}\)
\( \newcommand{\kernel}{\mathrm{null}\,}\)
\( \newcommand{\range}{\mathrm{range}\,}\)
\( \newcommand{\RealPart}{\mathrm{Re}}\)
\( \newcommand{\ImaginaryPart}{\mathrm{Im}}\)
\( \newcommand{\Argument}{\mathrm{Arg}}\)
\( \newcommand{\norm}[1]{\| #1 \|}\)
\( \newcommand{\inner}[2]{\langle #1, #2 \rangle}\)
\( \newcommand{\Span}{\mathrm{span}}\) \( \newcommand{\AA}{\unicode[.8,0]{x212B}}\)
\( \newcommand{\vectorA}[1]{\vec{#1}} % arrow\)
\( \newcommand{\vectorAt}[1]{\vec{\text{#1}}} % arrow\)
\( \newcommand{\vectorB}[1]{\overset { \scriptstyle \rightharpoonup} {\mathbf{#1}} } \)
\( \newcommand{\vectorC}[1]{\textbf{#1}} \)
\( \newcommand{\vectorD}[1]{\overrightarrow{#1}} \)
\( \newcommand{\vectorDt}[1]{\overrightarrow{\text{#1}}} \)
\( \newcommand{\vectE}[1]{\overset{-\!-\!\rightharpoonup}{\vphantom{a}\smash{\mathbf {#1}}}} \)
\( \newcommand{\vecs}[1]{\overset { \scriptstyle \rightharpoonup} {\mathbf{#1}} } \)
\( \newcommand{\vecd}[1]{\overset{-\!-\!\rightharpoonup}{\vphantom{a}\smash {#1}}} \)
\(\newcommand{\avec}{\mathbf a}\) \(\newcommand{\bvec}{\mathbf b}\) \(\newcommand{\cvec}{\mathbf c}\) \(\newcommand{\dvec}{\mathbf d}\) \(\newcommand{\dtil}{\widetilde{\mathbf d}}\) \(\newcommand{\evec}{\mathbf e}\) \(\newcommand{\fvec}{\mathbf f}\) \(\newcommand{\nvec}{\mathbf n}\) \(\newcommand{\pvec}{\mathbf p}\) \(\newcommand{\qvec}{\mathbf q}\) \(\newcommand{\svec}{\mathbf s}\) \(\newcommand{\tvec}{\mathbf t}\) \(\newcommand{\uvec}{\mathbf u}\) \(\newcommand{\vvec}{\mathbf v}\) \(\newcommand{\wvec}{\mathbf w}\) \(\newcommand{\xvec}{\mathbf x}\) \(\newcommand{\yvec}{\mathbf y}\) \(\newcommand{\zvec}{\mathbf z}\) \(\newcommand{\rvec}{\mathbf r}\) \(\newcommand{\mvec}{\mathbf m}\) \(\newcommand{\zerovec}{\mathbf 0}\) \(\newcommand{\onevec}{\mathbf 1}\) \(\newcommand{\real}{\mathbb R}\) \(\newcommand{\twovec}[2]{\left[\begin{array}{r}#1 \\ #2 \end{array}\right]}\) \(\newcommand{\ctwovec}[2]{\left[\begin{array}{c}#1 \\ #2 \end{array}\right]}\) \(\newcommand{\threevec}[3]{\left[\begin{array}{r}#1 \\ #2 \\ #3 \end{array}\right]}\) \(\newcommand{\cthreevec}[3]{\left[\begin{array}{c}#1 \\ #2 \\ #3 \end{array}\right]}\) \(\newcommand{\fourvec}[4]{\left[\begin{array}{r}#1 \\ #2 \\ #3 \\ #4 \end{array}\right]}\) \(\newcommand{\cfourvec}[4]{\left[\begin{array}{c}#1 \\ #2 \\ #3 \\ #4 \end{array}\right]}\) \(\newcommand{\fivevec}[5]{\left[\begin{array}{r}#1 \\ #2 \\ #3 \\ #4 \\ #5 \\ \end{array}\right]}\) \(\newcommand{\cfivevec}[5]{\left[\begin{array}{c}#1 \\ #2 \\ #3 \\ #4 \\ #5 \\ \end{array}\right]}\) \(\newcommand{\mattwo}[4]{\left[\begin{array}{rr}#1 \amp #2 \\ #3 \amp #4 \\ \end{array}\right]}\) \(\newcommand{\laspan}[1]{\text{Span}\{#1\}}\) \(\newcommand{\bcal}{\cal B}\) \(\newcommand{\ccal}{\cal C}\) \(\newcommand{\scal}{\cal S}\) \(\newcommand{\wcal}{\cal W}\) \(\newcommand{\ecal}{\cal E}\) \(\newcommand{\coords}[2]{\left\{#1\right\}_{#2}}\) \(\newcommand{\gray}[1]{\color{gray}{#1}}\) \(\newcommand{\lgray}[1]{\color{lightgray}{#1}}\) \(\newcommand{\rank}{\operatorname{rank}}\) \(\newcommand{\row}{\text{Row}}\) \(\newcommand{\col}{\text{Col}}\) \(\renewcommand{\row}{\text{Row}}\) \(\newcommand{\nul}{\text{Nul}}\) \(\newcommand{\var}{\text{Var}}\) \(\newcommand{\corr}{\text{corr}}\) \(\newcommand{\len}[1]{\left|#1\right|}\) \(\newcommand{\bbar}{\overline{\bvec}}\) \(\newcommand{\bhat}{\widehat{\bvec}}\) \(\newcommand{\bperp}{\bvec^\perp}\) \(\newcommand{\xhat}{\widehat{\xvec}}\) \(\newcommand{\vhat}{\widehat{\vvec}}\) \(\newcommand{\uhat}{\widehat{\uvec}}\) \(\newcommand{\what}{\widehat{\wvec}}\) \(\newcommand{\Sighat}{\widehat{\Sigma}}\) \(\newcommand{\lt}{<}\) \(\newcommand{\gt}{>}\) \(\newcommand{\amp}{&}\) \(\definecolor{fillinmathshade}{gray}{0.9}\)- Describe how nuclear fission and fusion generate energy.
Nuclear Fusion- The Power of the Sun
In addition to fission, a second possible method for obtaining energy from nuclear reactions lies in the fusing together of two light nuclei to form a heavier nucleus. As we see when discussing Figure 1 from Mass-Energy Relationships, such a process results in nucleons which are more firmly bonded to each other, and hence lower in potential energy. This is particularly true if \({}_{\text{2}}^{\text{4}}\text{He}\) is formed, because this nucleus is very stable. Such a reaction occurs between the nuclei of the two heavy isotopes of hydrogen, deuterium and tritium:
\[{}_{\text{1}}^{\text{2}}\text{D + }{}_{\text{1}}^{\text{3}}\text{T }\to \text{ }{}_{\text{2}}^{\text{4}}\text{He + }{}_{\text{0}}^{\text{1}}n \label{1} \]
For this reaction, Δm = – 0.018 88 g mol–1 so that ΔHm = – 1700 GJ mol–1. Although very large quantities of energy are released by a reaction like Equation \(\ref{1}\), such a reaction is very difficult to achieve in practice. This is because of the very high activation energy, about 30 GJ mol–1, which must be overcome to bring the nuclei close enough to fuse together. This barrier is created by coulombic repulsion between the positively charged nuclei. The only place where scientists have succeeded in producing fusion reactions on a large scale is in a hydrogen bomb. Here, the necessary activation energy is achieved by exploding a fission bomb to heat the reactants to a temperature of about 108 K. Attempts to carry out fusion in a more controlled way have met only limited success. At the very high temperatures required, all molecules dissociate and most atoms ionize. A new state of matter called a plasma is formed. It is neither solid, liquid, nor gas. Plasma behaves much like the universal solvent of the alchemists by converting any solid material that it contacts into vapor.
Two techniques for producing a controlled fusion reaction are currently being explored. The first is to restrict the plasma by means of a strong magnetic field, rather than the walls of a container. This has met some success, but has not yet been able to contain a plasma long enough for usable energy to be obtained. The second technique involves the sudden compression and heating of pellets of deuterium and tritium by means of a sharply focused laser beam. Again, only limited success has been obtained.
Though these attempts at a controlled fusion reaction have so far been only partially successful, they are nevertheless worth pursuing. Because of the much readier availability of lighter isotopes necessary for fusion, as opposed to the much rarer heavier isotopes required for fission, controlled nuclear fusion would offer the human race an essentially limitless supply of energy. There would still be some environmental difficulties with the production of isotopes such as tritium, but these would be nowhere near the seriousness of the problem caused by the production of the witches brew of radioactive isotopes in a fission reactor. It must be confessed, though, that at the present rate of progress, the prospect of limitless clean energy from fusion seems unlikely in the next decade or two.
Nuclear Fission Reactors
Fission reactions can be used in the production of electricity if we control the rate at which the fission occurs. The great majority of all electrical generating systems (whether coal burning power plants, hydroelectric plants, or nuclear power plants) is that they follow a reasonably simple design. The electricity is produced by spinning a coil of wire inside a magnetic field. When a fluid (air, steam, water) is forced through the pipe, it spins the fan blades, which in turn spin the axle. To generate electricity, the axle of a turbine is attached to the loop of wire in a generator. When a fluid is forced through the turbine, the fan blades turn, the turbine axle turns, and the loop of wire inside the generator turns—thus generating electricity.
The essential difference in various kinds of electrical generating systems is the method used to spin the turbine. For a wind generator, the turbine is a windmill. In a geothermal generator, steam from a geyser is forced through the turbine. In hydroelectric generating plants, water falling over a dam passes through the turbine and spins it. In fossil fuel (coal, oil, natural gas) generating plants, the fossil fuel is burned and the heat is used to boil water into steam, and then the steam passes through the turbine to make it spin. In a fission reactor generating plant, a fission reaction is used to boil the water into steam, and the steam passes through the turbine to make it spin. Once the steam is generated by the fission reaction, a nuclear power plant is essentially the same as a fossil fuel plant.
Naturally occurring uranium is composed almost totally of two uranium isotopes. It contains more than \(99\%\) uranium-238 and less than \(1\%\) uranium-235. It is the uranium-235, however, that is fissionable (will undergo fission). In order for uranium to be used as fuel in a fission reactor, the percentage of uranium-235 must be increased, usually to about \(3\%\). (Uranium in which the \(\ce{U}\)-235 content is more than \(1\%\) is called enriched uranium.)
Once the supply of \(\ce{U}\)-235 is acquired, it is placed in a series of long cylindrical tubes called fuel rods. These fuel cylinders are bundled together with control rods made of neutron-absorbing material. The amount of \(\ce{U}\)-235 in all the fuel rods taken together is adequate to carry on a chain reaction, but is less than the critical mass. (In the United States, all public nuclear power plants contain less than a critical mass of \(\ce{U}\)-235 and therefore, could never produce a nuclear explosion.) The amount of heat generated by the chain reaction is controlled by the rate at which the nuclear reaction occurs. The rate of the nuclear reaction is dependent on how many neutrons are emitted by one \(\ce{U}\)-235 nuclear disintegration and strike a new \(\ce{U}\)-235 nucleus to cause another disintegration. The purpose of the control rods is to absorb some of the neutrons and thus stop them from causing further disintegration. The control rods can be raised or lowered into the fuel rod bundle. When the control rods are lowered all the way into the fuel rod bundle, they absorb so many neutrons that the chain reaction essentially stops. When more heat is desired, the control rods are raised so that they catch fewer neutrons, the chain reaction speeds up, and more heat is generated. The control rods are operated in a fail-safe system, so that power is necessary to hold them up; during a power failure, gravity will pull the control rods down into the shut off position.
\(\ce{U}\)-235 nuclei can capture neutrons and disintegrate more efficiently if the neutrons are moving slower than the speed at which they are released. Fission reactors use a moderator surrounding the fuel rods to slow down the neutrons. Water is not only a good coolant, but also a good moderator. A common type of fission reactor has the fuel core submerged in a huge pool of water.
You can follow the operation of an electricity-generating fission reactor in the figure below. The reactor core is submerged in a pool of water. The heat from the fission reaction heats the water and the water is pumped into a heat exchanger container where the heated water boils the water in the heat exchanger. The steam from there is forced through a turbine which spins a generator and produces electricity. After the water passes through the turbine, it is condensed back to liquid water and pumped back to the heat exchanger.
In the United States, heavy opposition to the use of nuclear energy was mounted in the late 1960's and early 1970's. Every environmentalist organization in the US opposed the use of nuclear energy; the constant pressure from environmentalist groups caused an increase of public fear and, therefore, opposition to nuclear energy. This is not true today; at least one environmental leader has published a paper in favor of nuclear-powered electricity generation.
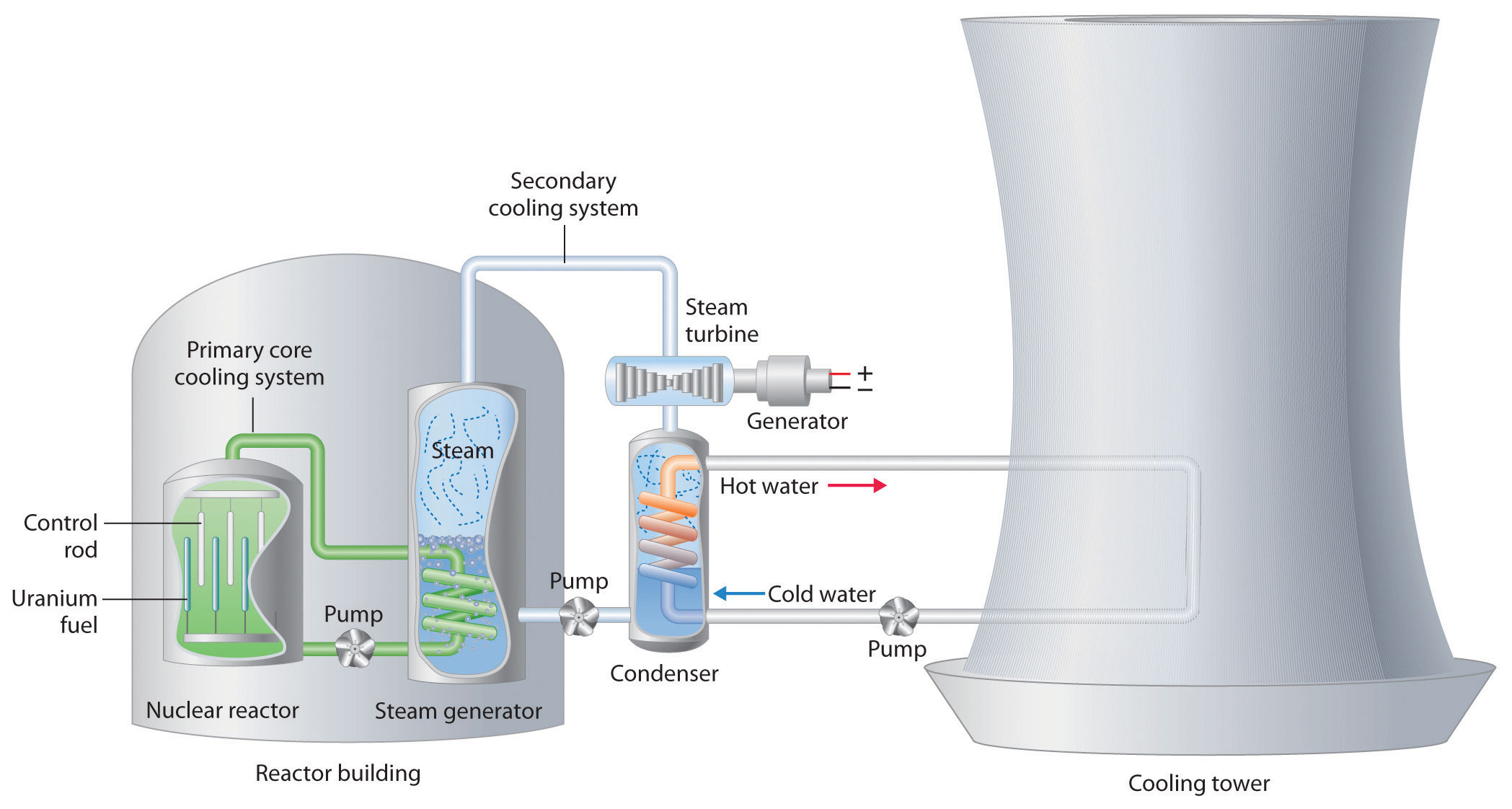
In 1979, a reactor core meltdown at Pennsylvania's Three Mile Island nuclear power plant reminded the entire country of the dangers of nuclear radiation. The concrete containment structure (six feet thick walls of reinforced concrete), however, did what it was designed to do—prevent radiation from escaping into the environment. Although the reactor was shut down for years, there were no injuries or deaths among nuclear workers or nearby residents. Three Mile Island was the only serious accident in the entire history of 103 civilian power plants operating for 40 years in the United States. There has never been a single injury or death due to radiation in any public nuclear power plant in the U.S. The accident at Three Mile Island did, however, frighten the public so that there has not been a nuclear power plant built in the U.S. since the accident.
The 103 nuclear power plants operating in the U.S. deliver approximately \(19.4\%\) of American electricity with zero greenhouse gas emission. There are 600 coal-burning electric plants in the US delivering \(48.5\%\) of American electricity and producing 2 billion tons of \(\ce{CO_2}\) annually, accounting for \(40\%\) of U.S. \(\ce{CO_2}\) emissions and \(10\%\) of global emissions. These coal burning plants also produce \(64\%\) of the sulfur dioxide emissions, \(26\%\) of the nitrous oxide emissions, and \(33\%\) of mercury emissions.