12.6: Energy and Phase Transitions
- Page ID
- 476618
\( \newcommand{\vecs}[1]{\overset { \scriptstyle \rightharpoonup} {\mathbf{#1}} } \)
\( \newcommand{\vecd}[1]{\overset{-\!-\!\rightharpoonup}{\vphantom{a}\smash {#1}}} \)
\( \newcommand{\id}{\mathrm{id}}\) \( \newcommand{\Span}{\mathrm{span}}\)
( \newcommand{\kernel}{\mathrm{null}\,}\) \( \newcommand{\range}{\mathrm{range}\,}\)
\( \newcommand{\RealPart}{\mathrm{Re}}\) \( \newcommand{\ImaginaryPart}{\mathrm{Im}}\)
\( \newcommand{\Argument}{\mathrm{Arg}}\) \( \newcommand{\norm}[1]{\| #1 \|}\)
\( \newcommand{\inner}[2]{\langle #1, #2 \rangle}\)
\( \newcommand{\Span}{\mathrm{span}}\)
\( \newcommand{\id}{\mathrm{id}}\)
\( \newcommand{\Span}{\mathrm{span}}\)
\( \newcommand{\kernel}{\mathrm{null}\,}\)
\( \newcommand{\range}{\mathrm{range}\,}\)
\( \newcommand{\RealPart}{\mathrm{Re}}\)
\( \newcommand{\ImaginaryPart}{\mathrm{Im}}\)
\( \newcommand{\Argument}{\mathrm{Arg}}\)
\( \newcommand{\norm}[1]{\| #1 \|}\)
\( \newcommand{\inner}[2]{\langle #1, #2 \rangle}\)
\( \newcommand{\Span}{\mathrm{span}}\) \( \newcommand{\AA}{\unicode[.8,0]{x212B}}\)
\( \newcommand{\vectorA}[1]{\vec{#1}} % arrow\)
\( \newcommand{\vectorAt}[1]{\vec{\text{#1}}} % arrow\)
\( \newcommand{\vectorB}[1]{\overset { \scriptstyle \rightharpoonup} {\mathbf{#1}} } \)
\( \newcommand{\vectorC}[1]{\textbf{#1}} \)
\( \newcommand{\vectorD}[1]{\overrightarrow{#1}} \)
\( \newcommand{\vectorDt}[1]{\overrightarrow{\text{#1}}} \)
\( \newcommand{\vectE}[1]{\overset{-\!-\!\rightharpoonup}{\vphantom{a}\smash{\mathbf {#1}}}} \)
\( \newcommand{\vecs}[1]{\overset { \scriptstyle \rightharpoonup} {\mathbf{#1}} } \)
\( \newcommand{\vecd}[1]{\overset{-\!-\!\rightharpoonup}{\vphantom{a}\smash {#1}}} \)
\(\newcommand{\avec}{\mathbf a}\) \(\newcommand{\bvec}{\mathbf b}\) \(\newcommand{\cvec}{\mathbf c}\) \(\newcommand{\dvec}{\mathbf d}\) \(\newcommand{\dtil}{\widetilde{\mathbf d}}\) \(\newcommand{\evec}{\mathbf e}\) \(\newcommand{\fvec}{\mathbf f}\) \(\newcommand{\nvec}{\mathbf n}\) \(\newcommand{\pvec}{\mathbf p}\) \(\newcommand{\qvec}{\mathbf q}\) \(\newcommand{\svec}{\mathbf s}\) \(\newcommand{\tvec}{\mathbf t}\) \(\newcommand{\uvec}{\mathbf u}\) \(\newcommand{\vvec}{\mathbf v}\) \(\newcommand{\wvec}{\mathbf w}\) \(\newcommand{\xvec}{\mathbf x}\) \(\newcommand{\yvec}{\mathbf y}\) \(\newcommand{\zvec}{\mathbf z}\) \(\newcommand{\rvec}{\mathbf r}\) \(\newcommand{\mvec}{\mathbf m}\) \(\newcommand{\zerovec}{\mathbf 0}\) \(\newcommand{\onevec}{\mathbf 1}\) \(\newcommand{\real}{\mathbb R}\) \(\newcommand{\twovec}[2]{\left[\begin{array}{r}#1 \\ #2 \end{array}\right]}\) \(\newcommand{\ctwovec}[2]{\left[\begin{array}{c}#1 \\ #2 \end{array}\right]}\) \(\newcommand{\threevec}[3]{\left[\begin{array}{r}#1 \\ #2 \\ #3 \end{array}\right]}\) \(\newcommand{\cthreevec}[3]{\left[\begin{array}{c}#1 \\ #2 \\ #3 \end{array}\right]}\) \(\newcommand{\fourvec}[4]{\left[\begin{array}{r}#1 \\ #2 \\ #3 \\ #4 \end{array}\right]}\) \(\newcommand{\cfourvec}[4]{\left[\begin{array}{c}#1 \\ #2 \\ #3 \\ #4 \end{array}\right]}\) \(\newcommand{\fivevec}[5]{\left[\begin{array}{r}#1 \\ #2 \\ #3 \\ #4 \\ #5 \\ \end{array}\right]}\) \(\newcommand{\cfivevec}[5]{\left[\begin{array}{c}#1 \\ #2 \\ #3 \\ #4 \\ #5 \\ \end{array}\right]}\) \(\newcommand{\mattwo}[4]{\left[\begin{array}{rr}#1 \amp #2 \\ #3 \amp #4 \\ \end{array}\right]}\) \(\newcommand{\laspan}[1]{\text{Span}\{#1\}}\) \(\newcommand{\bcal}{\cal B}\) \(\newcommand{\ccal}{\cal C}\) \(\newcommand{\scal}{\cal S}\) \(\newcommand{\wcal}{\cal W}\) \(\newcommand{\ecal}{\cal E}\) \(\newcommand{\coords}[2]{\left\{#1\right\}_{#2}}\) \(\newcommand{\gray}[1]{\color{gray}{#1}}\) \(\newcommand{\lgray}[1]{\color{lightgray}{#1}}\) \(\newcommand{\rank}{\operatorname{rank}}\) \(\newcommand{\row}{\text{Row}}\) \(\newcommand{\col}{\text{Col}}\) \(\renewcommand{\row}{\text{Row}}\) \(\newcommand{\nul}{\text{Nul}}\) \(\newcommand{\var}{\text{Var}}\) \(\newcommand{\corr}{\text{corr}}\) \(\newcommand{\len}[1]{\left|#1\right|}\) \(\newcommand{\bbar}{\overline{\bvec}}\) \(\newcommand{\bhat}{\widehat{\bvec}}\) \(\newcommand{\bperp}{\bvec^\perp}\) \(\newcommand{\xhat}{\widehat{\xvec}}\) \(\newcommand{\vhat}{\widehat{\vvec}}\) \(\newcommand{\uhat}{\widehat{\uvec}}\) \(\newcommand{\what}{\widehat{\wvec}}\) \(\newcommand{\Sighat}{\widehat{\Sigma}}\) \(\newcommand{\lt}{<}\) \(\newcommand{\gt}{>}\) \(\newcommand{\amp}{&}\) \(\definecolor{fillinmathshade}{gray}{0.9}\)- Predict the direction of energy flow associated with each phase change.
- Interpret a heating curve.
In the previous subsection we discussed transitions between different physical states. But why do these transitions occur? You might associate something that freezes with a low temperature and something that melts with a high temperature. We have seen frozen water and melted wax that are consistent with this perception. And based on what we already know from our study of energy earlier in this text, we might assume that heat transfers can lead to phase changes. This is correct, and we will explore this concept in this section.
Heat and Evaporation
In order for a liquid molecule to escape into the gas state, the molecule must have enough kinetic energy to overcome the intermolecular attractive forces in the liquid. Recall that a given liquid sample will have molecules with a wide range of kinetic energies. Liquid molecules that have this certain threshold kinetic energy escape the surface and become vapor. As a result, the liquid molecules that remain now have lower kinetic energy. As evaporation occurs, the temperature of the remaining liquid decreases. You have observed the effects of evaporative cooling. On a hot day, the water molecules in your perspiration absorb body heat and evaporate from the surface of your skin. The evaporation process leaves the remaining perspiration cooler, which in turn absorbs more heat from your body.
A given liquid will evaporate more quickly when it is heated. This is because the heating process results in a greater fraction of the liquid's molecules having the necessary kinetic energy to escape the surface of the liquid. The figure below shows the kinetic energy distribution of liquid molecules at two temperatures. The numbers of molecules that have the required kinetic energy to evaporate are shown in the shaded area under the curve at the right. The higher temperature liquid \(\left( T_2 \right)\) has more molecules that are capable of escaping into the vapor phase than the lower temperature liquid \(\left( T_1 \right)\).

Heating Curves
Imagine that you have a block of ice that is at a temperature of \(-30^\text{o} \text{C}\), well below its melting point. The ice is in a closed container. As heat is steadily added to the ice block, the water molecules will begin to vibrate faster and faster as they absorb kinetic energy. Eventually, when the ice has warmed to \(0^\text{o} \text{C}\), the added energy will start to break apart the hydrogen bonding that keeps the water molecules in place when it is in the solid form. As the ice melts, its temperature does not rise. All of the energy that is being put into the ice goes into the melting process and not into any increase in temperature. During the melting process, the two states—solid and liquid—are in equilibrium with one another. If the system was isolated at that point and no energy was allowed to enter or leave, the ice-water mixture at \(0^\text{o} \text{C}\) would remain. Temperature is always constant during a change of state.
Continued heating of the water after the ice has completely melted will now increase the kinetic energy of the liquid molecules and the temperature will rise. Assuming that the atmospheric pressure is standard, the temperature will rise steadily until it reaches \(100^\text{o} \text{C}\). At this point, the added energy from the heat will cause the liquid to begin to vaporize. As with the previous state change, the temperature will remain at \(100^\text{o} \text{C}\) while the water molecules are going from the liquid to the gas or vapor state. Once all the liquid has completely boiled away, continued heating of the steam (since the container is closed) will increase its temperature above \(100^\text{o} \text{C}\).
The experiment described above can be summarized in a graph called a heating curve (figure below).
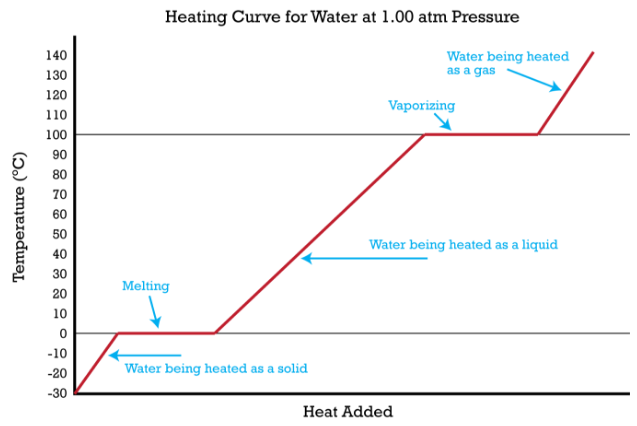
The change of state behavior of all substances can be represented with a heating curve of this type. The melting and boiling points of the substance can be determined by the horizontal lines or plateaus on the curve. Other substances have melting and boiling points that are different from those of water. An exception to this blueprint heating curve is for a substance such as carbon dioxide, which sublimes rather than melts at standard pressure. The heating curve for carbon dioxide would have only one plateau, at the sublimation temperature of \(\ce{CO_2}\).
The entire experiment could be run in reverse. Steam above \(100^\text{o} \text{C}\) could be steadily cooled down to \(100^\text{o} \text{C}\), at which point it would condense to liquid water. The water could then be cooled to \(0^\text{o} \text{C}\), at which point continued cooling would freeze the water to ice. The ice could then be cooled to a point below \(0^\text{o} \text{C}\). This could be diagrammed in a cooling curve that would be the reverse of the heating curve.
Summary of Energy and Phase Changes
Some physical changes of state require energy to be added for them to occur, while others require that energy be removed in order for them to occur. Changes of state that require energy to be added are evaporation, boiling, melting, and sublimation. Changes of state that require energy to be removed are condensation, freezing, and deposition. Whether energy is being added or removed, those changes of state will occur at a constant temperature. When a substance is not at the temperature of a phase change, any energy added to that substance will cause the temperature to increase. Likewise, any energy removed from it will cause the temperature to decrease.
Section Summary
- A change of state can be brought about by putting heat into a system or removing heat from the system.
- The temperature of a system will not change as long as the substance is undergoing a change from solid to liquid to gas, as well as the reverse.
- Freezing is the opposite of melting.
- Evaporation occurs when a liquid turns to a gas.
- Condensation is the opposite of vaporization.
- Deposition is the opposite of sublimation.