9.4: Citric acid cycle
- Page ID
- 433999
\( \newcommand{\vecs}[1]{\overset { \scriptstyle \rightharpoonup} {\mathbf{#1}} } \)
\( \newcommand{\vecd}[1]{\overset{-\!-\!\rightharpoonup}{\vphantom{a}\smash {#1}}} \)
\( \newcommand{\id}{\mathrm{id}}\) \( \newcommand{\Span}{\mathrm{span}}\)
( \newcommand{\kernel}{\mathrm{null}\,}\) \( \newcommand{\range}{\mathrm{range}\,}\)
\( \newcommand{\RealPart}{\mathrm{Re}}\) \( \newcommand{\ImaginaryPart}{\mathrm{Im}}\)
\( \newcommand{\Argument}{\mathrm{Arg}}\) \( \newcommand{\norm}[1]{\| #1 \|}\)
\( \newcommand{\inner}[2]{\langle #1, #2 \rangle}\)
\( \newcommand{\Span}{\mathrm{span}}\)
\( \newcommand{\id}{\mathrm{id}}\)
\( \newcommand{\Span}{\mathrm{span}}\)
\( \newcommand{\kernel}{\mathrm{null}\,}\)
\( \newcommand{\range}{\mathrm{range}\,}\)
\( \newcommand{\RealPart}{\mathrm{Re}}\)
\( \newcommand{\ImaginaryPart}{\mathrm{Im}}\)
\( \newcommand{\Argument}{\mathrm{Arg}}\)
\( \newcommand{\norm}[1]{\| #1 \|}\)
\( \newcommand{\inner}[2]{\langle #1, #2 \rangle}\)
\( \newcommand{\Span}{\mathrm{span}}\) \( \newcommand{\AA}{\unicode[.8,0]{x212B}}\)
\( \newcommand{\vectorA}[1]{\vec{#1}} % arrow\)
\( \newcommand{\vectorAt}[1]{\vec{\text{#1}}} % arrow\)
\( \newcommand{\vectorB}[1]{\overset { \scriptstyle \rightharpoonup} {\mathbf{#1}} } \)
\( \newcommand{\vectorC}[1]{\textbf{#1}} \)
\( \newcommand{\vectorD}[1]{\overrightarrow{#1}} \)
\( \newcommand{\vectorDt}[1]{\overrightarrow{\text{#1}}} \)
\( \newcommand{\vectE}[1]{\overset{-\!-\!\rightharpoonup}{\vphantom{a}\smash{\mathbf {#1}}}} \)
\( \newcommand{\vecs}[1]{\overset { \scriptstyle \rightharpoonup} {\mathbf{#1}} } \)
\( \newcommand{\vecd}[1]{\overset{-\!-\!\rightharpoonup}{\vphantom{a}\smash {#1}}} \)
\(\newcommand{\avec}{\mathbf a}\) \(\newcommand{\bvec}{\mathbf b}\) \(\newcommand{\cvec}{\mathbf c}\) \(\newcommand{\dvec}{\mathbf d}\) \(\newcommand{\dtil}{\widetilde{\mathbf d}}\) \(\newcommand{\evec}{\mathbf e}\) \(\newcommand{\fvec}{\mathbf f}\) \(\newcommand{\nvec}{\mathbf n}\) \(\newcommand{\pvec}{\mathbf p}\) \(\newcommand{\qvec}{\mathbf q}\) \(\newcommand{\svec}{\mathbf s}\) \(\newcommand{\tvec}{\mathbf t}\) \(\newcommand{\uvec}{\mathbf u}\) \(\newcommand{\vvec}{\mathbf v}\) \(\newcommand{\wvec}{\mathbf w}\) \(\newcommand{\xvec}{\mathbf x}\) \(\newcommand{\yvec}{\mathbf y}\) \(\newcommand{\zvec}{\mathbf z}\) \(\newcommand{\rvec}{\mathbf r}\) \(\newcommand{\mvec}{\mathbf m}\) \(\newcommand{\zerovec}{\mathbf 0}\) \(\newcommand{\onevec}{\mathbf 1}\) \(\newcommand{\real}{\mathbb R}\) \(\newcommand{\twovec}[2]{\left[\begin{array}{r}#1 \\ #2 \end{array}\right]}\) \(\newcommand{\ctwovec}[2]{\left[\begin{array}{c}#1 \\ #2 \end{array}\right]}\) \(\newcommand{\threevec}[3]{\left[\begin{array}{r}#1 \\ #2 \\ #3 \end{array}\right]}\) \(\newcommand{\cthreevec}[3]{\left[\begin{array}{c}#1 \\ #2 \\ #3 \end{array}\right]}\) \(\newcommand{\fourvec}[4]{\left[\begin{array}{r}#1 \\ #2 \\ #3 \\ #4 \end{array}\right]}\) \(\newcommand{\cfourvec}[4]{\left[\begin{array}{c}#1 \\ #2 \\ #3 \\ #4 \end{array}\right]}\) \(\newcommand{\fivevec}[5]{\left[\begin{array}{r}#1 \\ #2 \\ #3 \\ #4 \\ #5 \\ \end{array}\right]}\) \(\newcommand{\cfivevec}[5]{\left[\begin{array}{c}#1 \\ #2 \\ #3 \\ #4 \\ #5 \\ \end{array}\right]}\) \(\newcommand{\mattwo}[4]{\left[\begin{array}{rr}#1 \amp #2 \\ #3 \amp #4 \\ \end{array}\right]}\) \(\newcommand{\laspan}[1]{\text{Span}\{#1\}}\) \(\newcommand{\bcal}{\cal B}\) \(\newcommand{\ccal}{\cal C}\) \(\newcommand{\scal}{\cal S}\) \(\newcommand{\wcal}{\cal W}\) \(\newcommand{\ecal}{\cal E}\) \(\newcommand{\coords}[2]{\left\{#1\right\}_{#2}}\) \(\newcommand{\gray}[1]{\color{gray}{#1}}\) \(\newcommand{\lgray}[1]{\color{lightgray}{#1}}\) \(\newcommand{\rank}{\operatorname{rank}}\) \(\newcommand{\row}{\text{Row}}\) \(\newcommand{\col}{\text{Col}}\) \(\renewcommand{\row}{\text{Row}}\) \(\newcommand{\nul}{\text{Nul}}\) \(\newcommand{\var}{\text{Var}}\) \(\newcommand{\corr}{\text{corr}}\) \(\newcommand{\len}[1]{\left|#1\right|}\) \(\newcommand{\bbar}{\overline{\bvec}}\) \(\newcommand{\bhat}{\widehat{\bvec}}\) \(\newcommand{\bperp}{\bvec^\perp}\) \(\newcommand{\xhat}{\widehat{\xvec}}\) \(\newcommand{\vhat}{\widehat{\vvec}}\) \(\newcommand{\uhat}{\widehat{\uvec}}\) \(\newcommand{\what}{\widehat{\wvec}}\) \(\newcommand{\Sighat}{\widehat{\Sigma}}\) \(\newcommand{\lt}{<}\) \(\newcommand{\gt}{>}\) \(\newcommand{\amp}{&}\) \(\definecolor{fillinmathshade}{gray}{0.9}\)- Understand the citric acid cycle, its reactions, and the yield of energetic molecules.
What is the citric acid cycle?
The citric acid cycle is the first part of the third stage of food catabolism, including carbohydrates, fats, and proteins. It is called the citric acid cycle because a \(\ce{2C's}\) acetyl-\(\ce{CoA}\) produced in the second stage of catabolism of foods reacts with a \(\ce{4C's}\) oxaloacetate and produces \(\ce{6C's}\)-citrate in the first reaction. The citrate is oxidized through a series of eight reactions producing two carbon dioxide (\(\ce{CO2}\)) and re-generates the oxaloacetate to repeat the next round of the cycle, as shown in Figure \(\PageIndex{1}\). It is also called the tricarboxylic acid cycle because citrate has three carboxylates (\(\ce{-COO^{-}}\)) groups, i.e., triacid. Another name for it is the Krebs cycle in honor of Hans Krebs, who discovered this metabolic pathway.
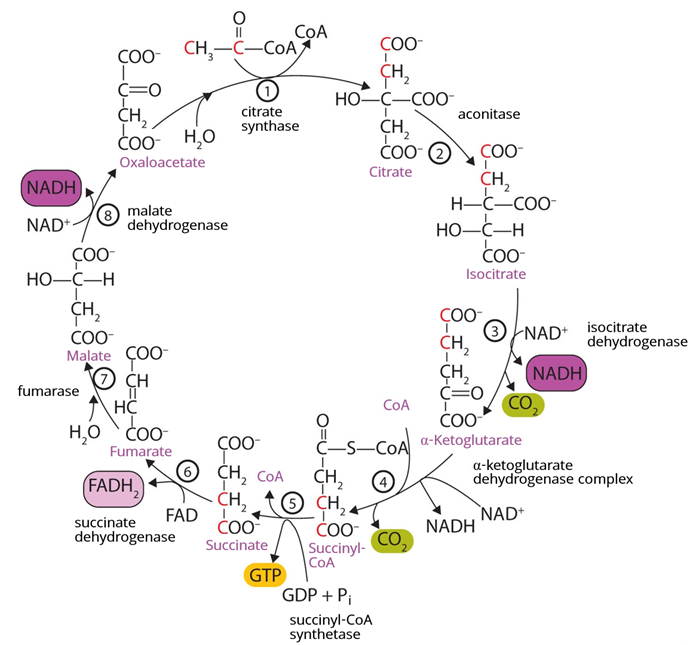
The oxidation reactions in the citric acid cycle release energy, which is coupled to the reduction of \(\ce{NAD^{+}}\) to \(\ce{NADH}\), \(\ce{FAD}\) to \(\ce{FADH}\), or conversions of \(\ce{ADP}\) to high-energy \(\ce{ATP}\). Guanosine triphosphate (\(\ce{GTP}\)) -another high-energy molecule is also produced from guanosine diphosphate (\(\ce{GDP}\)), but this reaction ultimately reverses to transfer its energy to make \(\ce{ATP}\).
Reactions of the citric acid cycle
The following equations of the reactions are modified from a public domain at Wikipedia.
1. To understand the first reaction, recall that the \(\ce{-CH3}\) group of acetyl-\(\ce{CoA}\) has relatively acidic protons as it is \(\alpha\) to a carbonyl (\(\ce{C=O}\)) group. An enzyme, acting as a base, removes a proton from the \(\ce{-CH3}\) group making it a carbanion which, being a strong nucleophile, attacks the ketone-\(\ce{C}\) of oxaloacetate, which is an electrophile. This nucleophilic addition reaction converts the \(\ce{C=O}\) into an \(\ce{-OH}\) group. It is followed by the hydrolysis of the thioester by \(\ce{H2O}\) through nucleophilic acyl substitution mechanism producing citrate and \(\ce{HS-CoA}\), as shown in the following overall reaction.
2. To understand the second reaction, recall that tertiary \(\ce{-OH}\) do not oxidize but are easily eliminated through E2-dehydration mechanics. The tertiary \(\ce{-OH}\) of citrate is eliminated, and then \(\ce{H2O}\) adds to the alkene intermediate, but it installs a secondary \(\ce{-OH}\) group in isocitrate product, as shown below.
3. The third reaction has three steps. In the first step, the secondary \(\ce{-OH}\) in isocitrate is oxidized to a ketone group at the expense of reduction of a \(\ce{NAD^{+}}\) into \(\ce{NADH}\). In this step, one \(\ce{-H}\) is picked up by an enzyme (\(\ce{A^{-}}\)) and the second by the nicotinamide ring (shown in blue color) of the coenzyme \(\ce{NAD^{+}}\). The \(\ce{COO^{-}}\) group in the intermediate-I, being \(\beta\) to the \(\ce{C=O}\) group, eliminates (decarboxylate) easily as \(\ce{CO2}\). The decarboxylation is facilitated by cofactor \(\ce{Mg^{2+}}\) that binds with and draws electrons from the \(\ce{O's}\). The enzyme \(\ce{H-A}\) protonates the enole \(\ce{C=C}\) bond of the intermediate-II that produces \(\alpha\)-ketoglutarate, as shown below.
4. The fourth reaction resembles the previous link reaction's oxidative decarboxylation of pyruvate to acetyl-\(\ce{CoA}\). In this reaction, \(\ce{NAD^{+}}\) is reduced into \(\ce{NADH}\) at the expense of oxidative decarboxylation of \(\alpha\)-ketoglutarate and the resulting acyl-group is transferred to \(\ce{HS-CoA}\) resulting in succinyl-\(\ce{CoA}\) product as shown in the reaction below.
5. The fifth reaction has two steps. In the first step, a phosphate (\(\ce{Pi}\)) group substitutes \(\ce{-S-CoA}\) group from succinyl-\(\ce{CoA}\). In the second step, the phosphate group is transferred to \(\ce{GDP}\) that converts to a \(\ce{GTP}\) by the same mechanism as in steps #7 and step#10 of glycolysis. \(\ce{GTP}\) reverts back to \(\ce{GDP}\) and transfers its phosphate group to \(\ce{ADP}\) that converts to one \(\ce{ATP}\). So, production of one \(\ce{GTP}\) is equal to production of one \(\ce{ATP}\). The overall reaction five is shown below.
6. In the sixth reaction, one \(\ce{FAD}\) is reduced into \(\ce{FADH_{2}}\) at the expense of oxidation of succinate to fumarate by the following overall reaction.
7. In the seventh reaction \(\ce{H2O}\) hydrates the \(\ce{C=C}\) of fumarate producing malate, as shown below.
Recall that the enzymes are stereospecific. In this case, only fumarate (not its cis-isomer) reacts with the enzyme, and only (S)-malate (not its enantiomers (R)-malate) is produced.
8. In the eighth step, one more \(\ce{NAD^{+}}\) is reduced into \(\ce{NADH}\) at the expense of oxidation of malate into oxaloacetate, as shown in the reaction below.
The oxaloacetate is the reactant of step#1 and begins the cycle again by reacting with another acetyl-. One citric acid cycle has the following overall reaction with one acetyl-\(\ce{CoA}\).
\(\ce{ \underbrace{CH3-\!\!-\!\!{\overset{\overset{\huge\enspace\!{O}}|\!\!|\enspace}{C}}\!\!-S-CoA}_{Acetyl\;CoA} + 3NAD^{+} + FAD + GDP + P_{i} + 2H2O -> HS-CoA + 3NADH + 3H^{+} + FADH_{2} + GTP + 2CO2}\)
Recall that the catabolism of one glucose molecule produces two pyruvates that, intern, produce two acetyl-\(\ce{CoA}\) in the oxidative decarboxylation of pyruvate under aerobic conditions. So, the citric acid cycle runs twice for the catabolism of one glucose molecule. One \(\ce{GTP}\) ultimately converts into one \(\ce{ATP}\).
Two citric acids from one glucose molecule yield ten energetic molecules from two turns citric acid cycles: \(\ce{6NADH}\), \(\ce{2FADH_{2}}\), and \(\ce{2ATP}\)
The information in this note is meant to help predict which energetic molecule will be produced or consumed during food catabolism reactions. Recall that oxidation generally release energy (exothermic), and reduction consumes energy (endothermic). The energy released from oxidation reactions in food catabolism is utilized to produce one of the two energetic coenzymes, i.e., either \(\ce{NADH}\) from the reduction of \(\ce{NAD^{+}}\) or \(\ce{FADH2}\) from the reduction of \(\ce{FAD}\). Recall that \(\ce{C=O}\) is stronger bond (~800 kJ/mol bond dissociation energy) compared to \(\ce{C=C}\) bond (~600 kJ/mole bond dissociation energy). \(\ce{NADH}\) is more energetic than \(\ce{FADH2}\). Therefore, any oxidation reaction involving carbonyl (\(\ce{C=O}\)) group produces \(\ce{NADH}\) and any oxidation involving \(\ce{C=C}\) bond produces \(\ce{FADH2}\). There is only one reaction described in food catabolism in this book, i.e., reaction#6 in the citric acid cycle that involved oxidation of \(\ce{C-C}\) to \(\ce{C=C}\) bond and produced \(\ce{FADH2}\). All other oxidation reactions in the citric acid cycle and glycolysis involve \(\ce{C=O}\) group, i.e., either alcohol (\(\ce{CH-OH}\)) to carbonyl (\(\ce{C=O}\)) conversion, or ketone (\(\ce{R-\!\!-\!\!{\overset{\overset{\huge\enspace\!{O}}|\!\!|\enspace}{C}}\!\!-R'}\) to thioester (\(\ce{R-\!\!-\!\!{\overset{\overset{\huge\enspace\!{O}}|\!\!|\enspace}{C}}\!\!-S-R"}\)) conversions produce \(\ce{NAD}\). Conversionerisons of pyruvate to lactate and ethanol under anaerobic conditions are reduction reactions involving \(\ce{C=O}\) group and consume \(\ce{NADH}\).
Reactions that are not redox reactions, e.g., acyl (\(\ce{ R-\!\!-\!\!{\overset{\overset{\huge\enspace\!{O}}|\!\!|\enspace}{C}}\!\!-}\)) transfer, hydration (addition of \(\ce{H2O}\)) , dehydration (elimination of \(\ce{H2O}\)), and isomerization, involve a small amount of energy. They are usually not coupled with the formation or consumption of energetic molecules like \(\ce{NADH}\), \(\ce{FADH2}\), or \(\ce{ATP}\).
Exceptions include phosphate ester or thioester group, which are high-energy groups. The addition or removal of phosphate ester or thioester groups without redox reaction is usually coupled with \(\ce{ATP}\)/ \(\ce{ADP}\) conversion. Two reactions, i.e., reaction#1 and reaction#3 in glycolysis (Figure 9.3.1) add phosphate ester bond and consume \(\ce{ATP}\), and two reactions, i.e., reaction#& and reaction#10, remove phosphate ester and produce \(\ce{ATP}\). One reaction, i.e., reaction#5 in the citric acid cycle (Figure 9.4.1), involves the replacing a thioester group with a carboxylate group without redox. It is coupled with the production of \(\ce{GTP}\), which, in turn, is associated with the output of \(\ce{ATP}\).
In summary:
- redox reactions involving \(\ce{C=O}\) group relate to \(\ce{NADH}\),
- redox reactions involving \(\ce{C=C}\) group relate to \(\ce{FADH2}\), and
- all other reactions that involve energetic groups like phosphate esters or thioesters relate to \(\ce{ATP}\) in food catabolism.
\(\ce{NADH}\) and \(\ce{FADH}\) are high-energy molecules that are oxidized by \(\ce{O2}\) in part two of the third stage of catabolism of food. The energy released from the oxidation of \(\ce{NADH}\) and \(\ce{FADH}\) is used to produce more \(\ce{ATPs}\), which is described in the next section.
The following video summarizes the citric acid cycle, also called the Krebs cycle.