8.4: Corrosion and Explosion
- Page ID
- 152185
\( \newcommand{\vecs}[1]{\overset { \scriptstyle \rightharpoonup} {\mathbf{#1}} } \)
\( \newcommand{\vecd}[1]{\overset{-\!-\!\rightharpoonup}{\vphantom{a}\smash {#1}}} \)
\( \newcommand{\id}{\mathrm{id}}\) \( \newcommand{\Span}{\mathrm{span}}\)
( \newcommand{\kernel}{\mathrm{null}\,}\) \( \newcommand{\range}{\mathrm{range}\,}\)
\( \newcommand{\RealPart}{\mathrm{Re}}\) \( \newcommand{\ImaginaryPart}{\mathrm{Im}}\)
\( \newcommand{\Argument}{\mathrm{Arg}}\) \( \newcommand{\norm}[1]{\| #1 \|}\)
\( \newcommand{\inner}[2]{\langle #1, #2 \rangle}\)
\( \newcommand{\Span}{\mathrm{span}}\)
\( \newcommand{\id}{\mathrm{id}}\)
\( \newcommand{\Span}{\mathrm{span}}\)
\( \newcommand{\kernel}{\mathrm{null}\,}\)
\( \newcommand{\range}{\mathrm{range}\,}\)
\( \newcommand{\RealPart}{\mathrm{Re}}\)
\( \newcommand{\ImaginaryPart}{\mathrm{Im}}\)
\( \newcommand{\Argument}{\mathrm{Arg}}\)
\( \newcommand{\norm}[1]{\| #1 \|}\)
\( \newcommand{\inner}[2]{\langle #1, #2 \rangle}\)
\( \newcommand{\Span}{\mathrm{span}}\) \( \newcommand{\AA}{\unicode[.8,0]{x212B}}\)
\( \newcommand{\vectorA}[1]{\vec{#1}} % arrow\)
\( \newcommand{\vectorAt}[1]{\vec{\text{#1}}} % arrow\)
\( \newcommand{\vectorB}[1]{\overset { \scriptstyle \rightharpoonup} {\mathbf{#1}} } \)
\( \newcommand{\vectorC}[1]{\textbf{#1}} \)
\( \newcommand{\vectorD}[1]{\overrightarrow{#1}} \)
\( \newcommand{\vectorDt}[1]{\overrightarrow{\text{#1}}} \)
\( \newcommand{\vectE}[1]{\overset{-\!-\!\rightharpoonup}{\vphantom{a}\smash{\mathbf {#1}}}} \)
\( \newcommand{\vecs}[1]{\overset { \scriptstyle \rightharpoonup} {\mathbf{#1}} } \)
\( \newcommand{\vecd}[1]{\overset{-\!-\!\rightharpoonup}{\vphantom{a}\smash {#1}}} \)
\(\newcommand{\avec}{\mathbf a}\) \(\newcommand{\bvec}{\mathbf b}\) \(\newcommand{\cvec}{\mathbf c}\) \(\newcommand{\dvec}{\mathbf d}\) \(\newcommand{\dtil}{\widetilde{\mathbf d}}\) \(\newcommand{\evec}{\mathbf e}\) \(\newcommand{\fvec}{\mathbf f}\) \(\newcommand{\nvec}{\mathbf n}\) \(\newcommand{\pvec}{\mathbf p}\) \(\newcommand{\qvec}{\mathbf q}\) \(\newcommand{\svec}{\mathbf s}\) \(\newcommand{\tvec}{\mathbf t}\) \(\newcommand{\uvec}{\mathbf u}\) \(\newcommand{\vvec}{\mathbf v}\) \(\newcommand{\wvec}{\mathbf w}\) \(\newcommand{\xvec}{\mathbf x}\) \(\newcommand{\yvec}{\mathbf y}\) \(\newcommand{\zvec}{\mathbf z}\) \(\newcommand{\rvec}{\mathbf r}\) \(\newcommand{\mvec}{\mathbf m}\) \(\newcommand{\zerovec}{\mathbf 0}\) \(\newcommand{\onevec}{\mathbf 1}\) \(\newcommand{\real}{\mathbb R}\) \(\newcommand{\twovec}[2]{\left[\begin{array}{r}#1 \\ #2 \end{array}\right]}\) \(\newcommand{\ctwovec}[2]{\left[\begin{array}{c}#1 \\ #2 \end{array}\right]}\) \(\newcommand{\threevec}[3]{\left[\begin{array}{r}#1 \\ #2 \\ #3 \end{array}\right]}\) \(\newcommand{\cthreevec}[3]{\left[\begin{array}{c}#1 \\ #2 \\ #3 \end{array}\right]}\) \(\newcommand{\fourvec}[4]{\left[\begin{array}{r}#1 \\ #2 \\ #3 \\ #4 \end{array}\right]}\) \(\newcommand{\cfourvec}[4]{\left[\begin{array}{c}#1 \\ #2 \\ #3 \\ #4 \end{array}\right]}\) \(\newcommand{\fivevec}[5]{\left[\begin{array}{r}#1 \\ #2 \\ #3 \\ #4 \\ #5 \\ \end{array}\right]}\) \(\newcommand{\cfivevec}[5]{\left[\begin{array}{c}#1 \\ #2 \\ #3 \\ #4 \\ #5 \\ \end{array}\right]}\) \(\newcommand{\mattwo}[4]{\left[\begin{array}{rr}#1 \amp #2 \\ #3 \amp #4 \\ \end{array}\right]}\) \(\newcommand{\laspan}[1]{\text{Span}\{#1\}}\) \(\newcommand{\bcal}{\cal B}\) \(\newcommand{\ccal}{\cal C}\) \(\newcommand{\scal}{\cal S}\) \(\newcommand{\wcal}{\cal W}\) \(\newcommand{\ecal}{\cal E}\) \(\newcommand{\coords}[2]{\left\{#1\right\}_{#2}}\) \(\newcommand{\gray}[1]{\color{gray}{#1}}\) \(\newcommand{\lgray}[1]{\color{lightgray}{#1}}\) \(\newcommand{\rank}{\operatorname{rank}}\) \(\newcommand{\row}{\text{Row}}\) \(\newcommand{\col}{\text{Col}}\) \(\renewcommand{\row}{\text{Row}}\) \(\newcommand{\nul}{\text{Nul}}\) \(\newcommand{\var}{\text{Var}}\) \(\newcommand{\corr}{\text{corr}}\) \(\newcommand{\len}[1]{\left|#1\right|}\) \(\newcommand{\bbar}{\overline{\bvec}}\) \(\newcommand{\bhat}{\widehat{\bvec}}\) \(\newcommand{\bperp}{\bvec^\perp}\) \(\newcommand{\xhat}{\widehat{\xvec}}\) \(\newcommand{\vhat}{\widehat{\vvec}}\) \(\newcommand{\uhat}{\widehat{\uvec}}\) \(\newcommand{\what}{\widehat{\wvec}}\) \(\newcommand{\Sighat}{\widehat{\Sigma}}\) \(\newcommand{\lt}{<}\) \(\newcommand{\gt}{>}\) \(\newcommand{\amp}{&}\) \(\definecolor{fillinmathshade}{gray}{0.9}\)- Understand the process of corrosion.
- Understand the process of explosion.
Corrosion is a galvanic process by which metals deteriorate through oxidation—usually but not always to their oxides. For example, when exposed to air, iron rusts, silver tarnishes, and copper and brass acquire a bluish-green surface called a patina. Of the various metals subject to corrosion, iron is by far the most important commercially. An estimated $100 billion per year is spent in the United States alone to replace iron-containing objects destroyed by corrosion.
Explosions can occur in nature due to a large influx of energy. Most natural explosions arise from volcanic processes of various sorts. Chemical explosions, usually involve a rapid and violent oxidation reaction that produces large amounts of hot gas. Gunpowder was the first explosive to be discovered and put to use. Other notable early developments in chemical explosive technology were Frederick Augustus Abel's development of nitrocellulose in 1865 and Alfred Nobel's invention of dynamite in 1866. Chemical explosions (both intentional and accidental) are often initiated by an electric spark or flame in the presence of oxygen. Accidental explosions may occur in fuel tanks, rocket engines, etc.
The Rusting of Iron
Perhaps the most familiar example of corrosion is the formation of rust on iron. Iron will rust when it is exposed to oxygen and water. The main steps in the rusting of iron appear to involve the following. Once exposed to the atmosphere, iron rapidly oxidizes.
\[\ce{Fe(s) -> Fe^{2+}(aq) + 2e^{-}} \tag{cathode} \]
The electrons reduce oxygen in the air in acidic solutions.
\[\ce{O2(g) + 4H^{+}(aq) + 4e^{-} -> 2H_2O(l)} \tag{anode} \]
\[\ce{2Fe(s) + O2(g) + 4H^{+}(aq) -> 2Fe^{2+}(aq) + 2H2O(l)} \tag{over all} \]
What we call rust is hydrated iron(III) oxide, which forms when iron(II) ions react further with oxygen.
\[\ce{4Fe^{2+}(aq) + O2(g) + (4+2x)H2O(l) -> 2Fe2O3 \cdot x H2O(s) + 8H^{+}(aq)} \nonumber \]
The number of water molecules is variable, so it is represented by x. Unlike the patina on copper, the formation of rust does not create a protective layer and so corrosion of the iron continues as the rust flakes off and exposes fresh iron to the atmosphere.
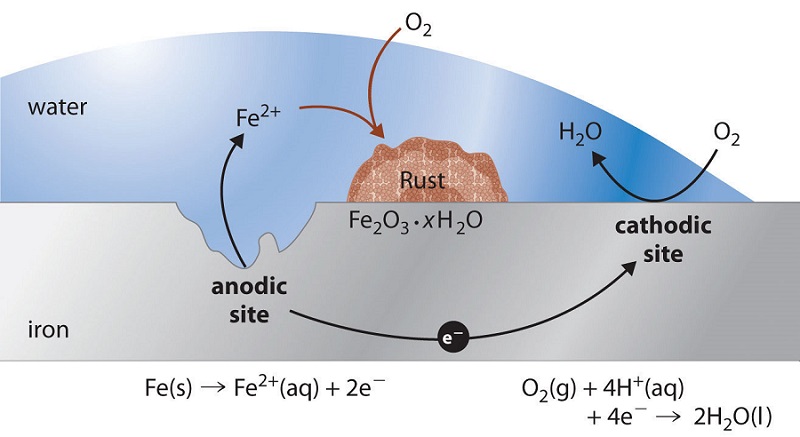
One way to keep iron from corroding is to keep it painted. Other strategies include alloying the iron with other metals. For example, stainless steel is mostly iron with a bit of chromium. Zinc-plated or galvanized iron uses a different strategy. Zinc is more easily oxidized than iron because zinc has a lower reduction potential. Since zinc has a lower reduction potential, it is a more active metal. Thus, even if the zinc coating is scratched, the zinc will still oxidize before the iron. This suggests that this approach should work with other active metals.
The Statue of Liberty is a landmark every American recognizes. The Statue of Liberty is easily identified by its height, stance, and unique blue-green color. When this statue was first delivered from France, its appearance was not green. It was brown, the color of its copper “skin.” So how did the Statue of Liberty change colors? The change in appearance was a direct result of corrosion. The copper that is the primary component of the statue slowly underwent oxidation from the air. The oxidation-reduction reactions of copper metal in the environment occur in several steps. Copper metal is oxidized to copper(I) oxide (\(\ce{Cu_2O}\)), which is red, and then to copper(II) oxide, which is black.
\[\ce{2Cu(s)} +\ce{1/2 O2(g)} \rightarrow \underset{\text{red}}{\ce{Cu2O(s)} } \nonumber \]
\[\ce{Cu2O(s)} +\ce{1/2 O2(g)}\rightarrow \underset{\text{black}}{\ce{2CuO(s)} } \nonumber \]
Coal, which was often high in sulfur, was burned extensively in the early part of the last century. As a result, sulfur trioxide, carbon dioxide, and water all reacted with the \(\ce{CuO}\).
\[\ce{2CuO(s)} + \ce{CO2(g)} + \ce{H2O(l)} \rightarrow \underset{\text{green}}{\ce{Cu2CO3(OH)2(s)} } \nonumber \]
\[\ce{3CuO(s)} + \ce{2CO2(g)}+\ce{H2O(l)} \rightarrow \underset{\text{blue}}{\ce{Cu_2(CO_3)2(OH)2(s)} } \nonumber \]
\[\ce{4CuO(s)} + \ce{SO3(g)}+\ce{3H2O(l)} \rightarrow \underset{\text{green}}{\ce{Cu_4SO4(OH)6(s)} } \nonumber \]
These three compounds are responsible for the characteristic blue-green patina seen today. Fortunately, formation of the patina created a protective layer on the surface, preventing further corrosion of the copper skin. The formation of the protective layer is a form of passivation, which is discussed elsewhere.
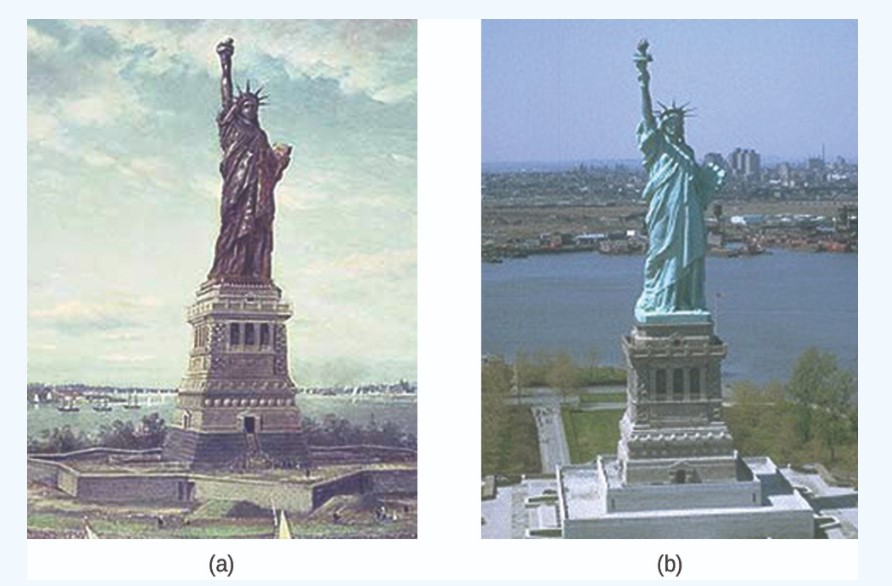
Silver Tarnishes
Even though coinage metals are known to be resistant to oxygen in air, silver will tarnish due to \(\ce{H2S(g)}\)
\[\ce{2Ag(s) + H_2S(g) -> Ag2S(s) + H2(g)} \nonumber \]
Explosive Reactions
TNT is one of the most commonly used explosives for military, industrial, and mining applications. TNT has been used in conjunction with hydraulic fracturing, a process used to recover oil and gas from shale formations. The technique involves displacing and detonating nitroglycerin in hydraulically induced fractures followed by wellbore shots using pelletized TNT.
TNT is valued partly because of its insensitivity to shock and friction, with reduced risk of accidental detonation compared to more sensitive explosives such as nitroglycerin. TNT melts at 80 °C (176 °F), far below the temperature at which it will spontaneously detonate, allowing it to be poured or safely combined with other explosives. TNT neither absorbs nor dissolves in water, which allows it to be used effectively in wet environments. To detonate, TNT must be triggered by a pressure wave from a starter explosive, called an explosive booster.
Although blocks of TNT are available in various sizes (e.g. 250 g, 500 g, 1,000 g), it is more commonly encountered in synergistic explosive blends comprising a variable percentage of TNT plus other ingredients
Upon detonation, TNT decomposes as follows:
\[\ce{2 C7H5N3O6 → 3 N2 + 5 H2O + 7 CO + 7 C} \nonumber \]
\[\ce{2 C7H5N3O6 → 3 N2 + 5 H2 + 12 CO + 2 C} \nonumber \]
The reaction is exothermic but has a high activation energy in the gas phase (~62 kcal/mol). The condensed phases (solid or liquid) show markedly lower activation energies of roughly 35 kcal/mol due to unique bimolecular decomposition routes at elevated densities. Because of the production of carbon, TNT explosions have a sooty appearance. Because TNT has an excess of carbon, explosive mixtures with oxygen-rich compounds can yield more energy per kilogram than TNT alone. During the 20th century, amatol, a mixture of TNT with ammonium nitrate was a widely used military explosive.
TNT can be detonated with a high velocity initiator or by efficient concussion. For many years, TNT used to be the reference point for the Figure of Insensitivity. TNT had a rating of exactly 100 on the "F of I" scale. The reference has since been changed to a more sensitive explosive called RDX, which has an F of I rating of 80.
ANFO (or AN/FO, for ammonium nitrate/fuel oil) is a widely used bulk industrial explosive. Its name is commonly pronounced as "an-fo". It consists of 94% porous prilled ammonium nitrate (\(\ce{NH4NO3}\)) (AN), which acts as the oxidizing agent and absorbent for the fuel, and 6% number 2 fuel oil (FO). The fuel component of ANFO is typically diesel, but kerosene, coal dust, racing fuel, or even molasses have been used instead. Finely powdered aluminium in the mixture will sensitise it to detonate more readily.
The chemistry of ANFO detonation is the reaction of ammonium nitrate with a long-chain alkane (\(\ce{C_nH_{$2n+2$}}\)) to form nitrogen, carbon dioxide, and water.
\[\ce{NH4NO3 + C_nH2_{$n+2$} → xN2 + yH2O + zCO2} \nonumber \]
In an ideal stoichiometrically balanced reaction, ANFO is composed of about 94.3% AN and 5.7% FO by \(\ce{weight. In practice, a slight excess of fuel oil is added, as underdosing results in reduced performance while overdosing merely results in more post-blast fumes. When detonation conditions are optimal, the aforementioned gases are the only products. In practical use, such conditions are impossible to attain, and blasts produce moderate amounts of toxic gases such as carbon monoxide and nitrogen oxides (NOx).
\[\ce{NH4NO3 + C_nH_{$2n+2$} → vCO + wNOx + yH2O + zCO2} \nonumber \]
ANFO has found wide use in coal mining, quarrying, metal mining, and civil construction in applications where its low cost and ease of use may outweigh the benefits of other explosives, such as water resistance, oxygen balance, higher detonation velocity, or performance in small-diameter columns. ANFO is also widely used in avalanche hazard mitigation.
Summary
- Corrosion and explosion are two major redox processes.
- Examples of corrosion (metal deterioration) and explosion (using TNT and ANFO) are given.
Contributors and Attributions
Paul Flowers (University of North Carolina - Pembroke), Klaus Theopold (University of Delaware) and Richard Langley (Stephen F. Austin State University) with contributing authors. Textbook content produced by OpenStax College is licensed under a Creative Commons Attribution License 4.0 license. Download for free at http://cnx.org/contents/85abf193-2bd...a7ac8df6@9.110).
- TextMap: Chemistry-A Central Science (Brown et al.)
- Wikipedia