4.3: Oxygen and oxides (Part 1)
- Page ID
- 125394
\( \newcommand{\vecs}[1]{\overset { \scriptstyle \rightharpoonup} {\mathbf{#1}} } \)
\( \newcommand{\vecd}[1]{\overset{-\!-\!\rightharpoonup}{\vphantom{a}\smash {#1}}} \)
\( \newcommand{\id}{\mathrm{id}}\) \( \newcommand{\Span}{\mathrm{span}}\)
( \newcommand{\kernel}{\mathrm{null}\,}\) \( \newcommand{\range}{\mathrm{range}\,}\)
\( \newcommand{\RealPart}{\mathrm{Re}}\) \( \newcommand{\ImaginaryPart}{\mathrm{Im}}\)
\( \newcommand{\Argument}{\mathrm{Arg}}\) \( \newcommand{\norm}[1]{\| #1 \|}\)
\( \newcommand{\inner}[2]{\langle #1, #2 \rangle}\)
\( \newcommand{\Span}{\mathrm{span}}\)
\( \newcommand{\id}{\mathrm{id}}\)
\( \newcommand{\Span}{\mathrm{span}}\)
\( \newcommand{\kernel}{\mathrm{null}\,}\)
\( \newcommand{\range}{\mathrm{range}\,}\)
\( \newcommand{\RealPart}{\mathrm{Re}}\)
\( \newcommand{\ImaginaryPart}{\mathrm{Im}}\)
\( \newcommand{\Argument}{\mathrm{Arg}}\)
\( \newcommand{\norm}[1]{\| #1 \|}\)
\( \newcommand{\inner}[2]{\langle #1, #2 \rangle}\)
\( \newcommand{\Span}{\mathrm{span}}\) \( \newcommand{\AA}{\unicode[.8,0]{x212B}}\)
\( \newcommand{\vectorA}[1]{\vec{#1}} % arrow\)
\( \newcommand{\vectorAt}[1]{\vec{\text{#1}}} % arrow\)
\( \newcommand{\vectorB}[1]{\overset { \scriptstyle \rightharpoonup} {\mathbf{#1}} } \)
\( \newcommand{\vectorC}[1]{\textbf{#1}} \)
\( \newcommand{\vectorD}[1]{\overrightarrow{#1}} \)
\( \newcommand{\vectorDt}[1]{\overrightarrow{\text{#1}}} \)
\( \newcommand{\vectE}[1]{\overset{-\!-\!\rightharpoonup}{\vphantom{a}\smash{\mathbf {#1}}}} \)
\( \newcommand{\vecs}[1]{\overset { \scriptstyle \rightharpoonup} {\mathbf{#1}} } \)
\( \newcommand{\vecd}[1]{\overset{-\!-\!\rightharpoonup}{\vphantom{a}\smash {#1}}} \)
\(\newcommand{\avec}{\mathbf a}\) \(\newcommand{\bvec}{\mathbf b}\) \(\newcommand{\cvec}{\mathbf c}\) \(\newcommand{\dvec}{\mathbf d}\) \(\newcommand{\dtil}{\widetilde{\mathbf d}}\) \(\newcommand{\evec}{\mathbf e}\) \(\newcommand{\fvec}{\mathbf f}\) \(\newcommand{\nvec}{\mathbf n}\) \(\newcommand{\pvec}{\mathbf p}\) \(\newcommand{\qvec}{\mathbf q}\) \(\newcommand{\svec}{\mathbf s}\) \(\newcommand{\tvec}{\mathbf t}\) \(\newcommand{\uvec}{\mathbf u}\) \(\newcommand{\vvec}{\mathbf v}\) \(\newcommand{\wvec}{\mathbf w}\) \(\newcommand{\xvec}{\mathbf x}\) \(\newcommand{\yvec}{\mathbf y}\) \(\newcommand{\zvec}{\mathbf z}\) \(\newcommand{\rvec}{\mathbf r}\) \(\newcommand{\mvec}{\mathbf m}\) \(\newcommand{\zerovec}{\mathbf 0}\) \(\newcommand{\onevec}{\mathbf 1}\) \(\newcommand{\real}{\mathbb R}\) \(\newcommand{\twovec}[2]{\left[\begin{array}{r}#1 \\ #2 \end{array}\right]}\) \(\newcommand{\ctwovec}[2]{\left[\begin{array}{c}#1 \\ #2 \end{array}\right]}\) \(\newcommand{\threevec}[3]{\left[\begin{array}{r}#1 \\ #2 \\ #3 \end{array}\right]}\) \(\newcommand{\cthreevec}[3]{\left[\begin{array}{c}#1 \\ #2 \\ #3 \end{array}\right]}\) \(\newcommand{\fourvec}[4]{\left[\begin{array}{r}#1 \\ #2 \\ #3 \\ #4 \end{array}\right]}\) \(\newcommand{\cfourvec}[4]{\left[\begin{array}{c}#1 \\ #2 \\ #3 \\ #4 \end{array}\right]}\) \(\newcommand{\fivevec}[5]{\left[\begin{array}{r}#1 \\ #2 \\ #3 \\ #4 \\ #5 \\ \end{array}\right]}\) \(\newcommand{\cfivevec}[5]{\left[\begin{array}{c}#1 \\ #2 \\ #3 \\ #4 \\ #5 \\ \end{array}\right]}\) \(\newcommand{\mattwo}[4]{\left[\begin{array}{rr}#1 \amp #2 \\ #3 \amp #4 \\ \end{array}\right]}\) \(\newcommand{\laspan}[1]{\text{Span}\{#1\}}\) \(\newcommand{\bcal}{\cal B}\) \(\newcommand{\ccal}{\cal C}\) \(\newcommand{\scal}{\cal S}\) \(\newcommand{\wcal}{\cal W}\) \(\newcommand{\ecal}{\cal E}\) \(\newcommand{\coords}[2]{\left\{#1\right\}_{#2}}\) \(\newcommand{\gray}[1]{\color{gray}{#1}}\) \(\newcommand{\lgray}[1]{\color{lightgray}{#1}}\) \(\newcommand{\rank}{\operatorname{rank}}\) \(\newcommand{\row}{\text{Row}}\) \(\newcommand{\col}{\text{Col}}\) \(\renewcommand{\row}{\text{Row}}\) \(\newcommand{\nul}{\text{Nul}}\) \(\newcommand{\var}{\text{Var}}\) \(\newcommand{\corr}{\text{corr}}\) \(\newcommand{\len}[1]{\left|#1\right|}\) \(\newcommand{\bbar}{\overline{\bvec}}\) \(\newcommand{\bhat}{\widehat{\bvec}}\) \(\newcommand{\bperp}{\bvec^\perp}\) \(\newcommand{\xhat}{\widehat{\xvec}}\) \(\newcommand{\vhat}{\widehat{\vvec}}\) \(\newcommand{\uhat}{\widehat{\uvec}}\) \(\newcommand{\what}{\widehat{\wvec}}\) \(\newcommand{\Sighat}{\widehat{\Sigma}}\) \(\newcommand{\lt}{<}\) \(\newcommand{\gt}{>}\) \(\newcommand{\amp}{&}\) \(\definecolor{fillinmathshade}{gray}{0.9}\)(a) Oxygen
Dioxygen, O2, is a colorless and odorless gas (bp -183.0 °C) that occupies 21% of air (volume ratio). Since oxygen atoms are also the major components of water and rocks, oxygen is the most abundant element on the Earth’s surface. Despite its abundance, it was established as an element as late as the 18th century. Since an immense quantity of oxygen gas is consumed for steel production now, it is separated in large quantities from liquified air.
The isotopes of oxygen are 16O (99.762% abundance), 17O (0.038%), and 18O (0.200%). 17O has nuclear spin I = 5/2 and is an important nuclide for NMR measurements. 18O is used as a tracer for tracking reagents or for the study of reaction mechanisms. It is also useful for the assignment of absorption lines in infrared or Raman spectra by means of isotope effects.
As already described in section 2.3 (e), dioxygen, O2, in the ground state has two unpaired spins in its molecular orbitals, shows paramagnetism and is called triplet dioxygen. In the excited state, the spins are paired and dioxygen becomes diamagnetic, which is called singlet dioxygen. Singlet dioxygen is important in synthetic chemistry, because it has characteristic oxidation reactivity. Singlet dioxygen is generated in a solution by an energy transfer reaction from a photo-activated complex or by the pyrolysis of ozonides (O3 compounds).
Superoxide ion, O2-, and peroxide ion, O22-, are the anions of dioxygen (Table \(\PageIndex{3}\)). They can be isolated as alkali metal salts. There is another state, O2+, called the dioxygen (1+) cation, and it can be isolated as a salt with suitable anions.
Bond order | Compound | O-O distance (Å) | ν(O-O) (cm-1) | |
---|---|---|---|---|
O2+ | 2.5 | O2[AsF6] | 1.123 | 1858 |
O2 | 2.0 | 1.207 | 1554 | |
O2- | 1.5 | K[O2] | 1.28 | 1145 |
O22- | 1.0 | Na2[O2] | 1.49 | 842 |
Ozone, O3, is an allotrope of oxygen that is an unstable gas with an irritating odor. Ozone is a bent three-atom molecule (117°) and has unique reactivities. In recent years it has been discovered that ozone plays an important role in intercepting the detrimental ultraviolet radiation from the sun in the upper atmospheric zone, and in protecting life on the Earth from photochemical damage. It is now clear that chlorofluorocarbons, frequently used as refrigerants or as cleaners of electronic components, destroy the ozone layer, and measures are being taken on a global scale to cope with this serious environmental problem.
(b) Oxides of hydrogen
Oxygen is highly reactive, and direct reactions with many elements form oxides. Water is an oxide of hydrogen and is crucially important for the global environment and life in general.
Water H2O
Ninety-seven percent of water on the Earth is present as sea water, 2% as ice of the polar zone, and fresh water represents only the small remaining fraction. Fundamental chemical and physical properties of water are very significant to chemistry. The main physical properties are shown in Table \(\PageIndex{1}\). Most of the unusual properties of water are caused by its strong hydrogen bonds. Physical properties of water differ considerably with the presence of isotopes of hydrogen. At least nine polymorphs of ice are known and their crystal structures depend on the freezing conditions of the ice.
Water has a bond angle of 104.5° and a bond distance of 95.7 pm as a free molecule. It is described in Section 3.4 (b) that self-dissociation of water generates oxonium ion, H3O+. Further water molecules add to H3O+ to form [H(OH2)n]+ (H5O2+, H7O3+, H9O4+, and H13O6+), and the structures of the various species have been determined.
Hydrogen peroxide H2O2
Hydrogen peroxide is an almost colorless liquid (mp -0.89 °C and bp (extrapolated) 151.4 °C) that is highly explosive and dangerous in high concentrations. Usually it is used as a dilute solution but occasionally 90% aqueous solutions are used. Since it is consumed in large quantities as a bleaching agent for fiber and paper, large-scale industrial synthetic process has been established. This process applies very subtle catalytic reactions to produce a dilute solution of hydrogen peroxide from air and hydrogen using a substituted anthraquinone. This dilute solution is then concentrated.
When deuterium peroxide is prepared in a laboratory, the following reaction is applied.
\[K_{2}S_{2}O_{8} + 2 D_{2} O \rightarrow D_{2} O + 2 KDSO_{4} \nonumber \]
Hydrogen peroxide is decomposed into oxygen and water in the presence of catalysts such as manganese dioxide, MnO2. Hydrogen peroxide may be either an oxidant or a reductant depending on its co-reactants. Its reduction potential in an acidic solution expressed in a Latimer diagram (refer to Section 3.3 (c)) is
\[O_{2} \xrightarrow{+0.70} H_{2}O_{2} \xrightarrow{+1.76} H_{2} O \nonumber \]
(c) Silicon oxides
Silicon oxides are formed by taking SiO4 tetrahedra as structural units and sharing the corner oxygen atoms. They are classified by the number of corner-sharing oxygen atoms in the SiO4 tetrahedra, as this determines their composition and structure. When the SiO4 tetrahedra connect by corner sharing, the structures of the polymeric compounds become a chain, a ring, a layer, or 3-dimensional depending on the connection modes of adjacent units. Fractional expression is adopted in order to show the bridging modes. Namely, the numerator in the fraction is the number of bridging oxygens and the denominator is 2, meaning that one oxygen atom is shared by two tetrahedra. The empirical formulae are as follows and each structure is illustrated in Figure \(\PageIndex{9}\) in coordination-polyhedron form.
- A bridge is constructed with one oxygen atom. (SiO3O1/2)3- = Si2O76-
- Bridges are constructed with two oxygen atoms. (SiO2O2/2)n2n- = (SiO3)n2n-
- Bridges are constructed with three oxygen atoms. (SiOO3/2)nn- = (Si2O5)n2n-
- Amalgamation of bridging modes with three oxygen and two oxygen atoms. [(Si2O5)(SiO2O2/2)2]n6- = (Si4O11)n6-
- Bridges are constructed with four oxygen atoms. (SiO4/2)n = (SiO2)n
Silicates with various cross linkage structures are contained in natural rocks, sand, clay, soil, etc.
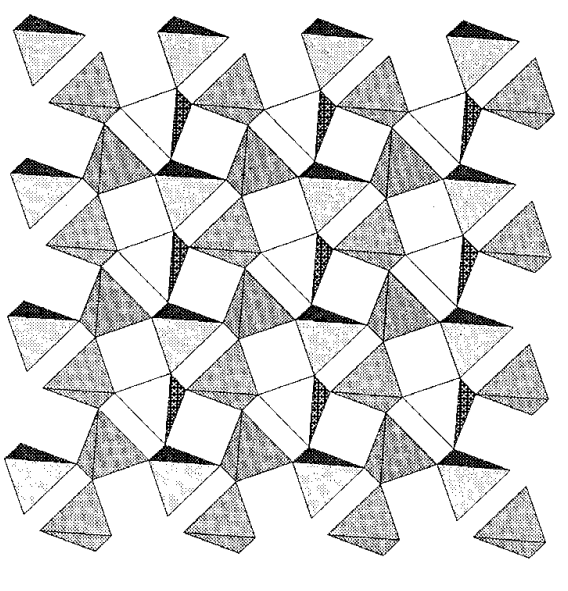
Aluminosilicates
There are many minerals in which some silicon atoms of silicate minerals are replaced by aluminum atoms. They are called aluminosilicates. Aluminum atoms replace the silicon atoms in the tetrahedral sites or occupy the octahedral cavities of oxygen atoms, making the structures more complicated. The substitution of a tetravalent silicon by a trivalent aluminum causes a shortage of charge which is compensated by occlusion of extra cations such as H+, Na+, Ca2+, etc. Feldspars are a typical aluminosilicate mineral, and KAlSi3O8 (orthoclase) and NaAlSi3O8 (albite) are also known well. Feldspars take 3-dimensional structures in which all the corners of the SiO4 and AlO4 tetrahedra are shared.
On the other hand, 2-dimensional layers are formed if [AlSiO5]3- units are lined, and stratified minerals like mica are constructed if 6-coordinate ions are inserted between layers. If the number of oxygen atoms in the layers is not enough to form regular octahedra between layers, hydroxide groups bond to the interstitial Al3+ ions. Muscovite, KAl2(OH)2Si3AlO10, is a type of mica with such a structure and can be eas ily peeled into layers.
Zeolite
One of the important aluminosilicates is zeolite. Zeolites are present as natural minerals and also many kinds of zeolites are prepared synthetically in large quantities. The SiO4 and AlO4 tetrahedra are bonded by oxygen bridges, and form holes and tunnels of various sizes. The structures are composites of the basic structural units of tetrahedral MO4. As shown in Figure \(\PageIndex{10}\), the basic units are cubes with 8 condensed MO4, hexagonal prisms with 12 condensed MO4, and truncated octahedra with 24 condensed MO4.
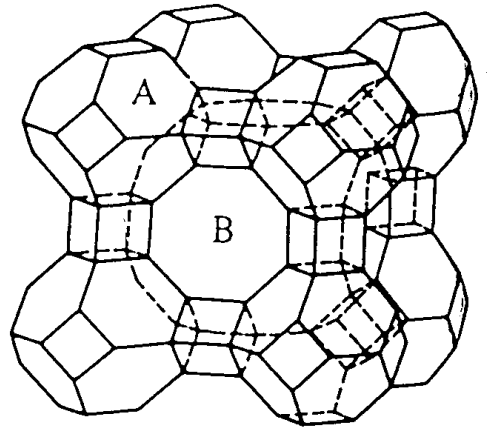
Silicon or aluminum atoms are located on the corners of the polyhedra and the bridging oxygen atoms on the middle of each edge (it should be noted that this expression is different from the polyhedron model of oxides).
When these polyhdra are bonded, various kinds of zeolite structures are formed. For example, the truncated octahedra called \(\beta\) cages are the basic frames of synthetic zeolite A, Na12(Al12Si12O48)] • 27H2O, and the quadrangle portions are connected through cubes. It can be seen that an octagonal tunnel B forms when eight truncated octahedra bind in this way. The structure in which the hexagon portions connect through hexagonal prisms is faujasite, NaCa0.5(Al2Si5O14)] • 10H2O.
Alkali metal or alkaline earth metal cations exist in the holes, and the number of these cations increases with the content of aluminum to compensate for the charge deficiency. The structures of zeolites have many crevices in which cations and water are contained. Utilizing this cation-exchange property, zeolites are used in large quantities as softeners of hard water. As zeolites dehydrated by heating absorb water efficiently, they are also used as desiccants of solvents or gases. Zeolites are sometimes called molecular sieves, since the sizes of holes and tunnels change with the kinds of zeolites and it is possible to segregate organic molecules according to their sizes. Zeolites can fix the directions of more than two molecules in their cavities and can be used as catalysts for selective reactions.
For example, synthetic zeolite ZSM-5 is useful as a catalyst to convert methanol to gasoline. This zeolite is prepared hydrothermally in an autoclave (high-pressure reaction vessel) at ca. 100 °C using meta-sodium luminate, NaAlO2, as the source of aluminum oxide and silica sol as the source of silicon oxide with tetrapropylammonium bromide, Pr4NBr, present in the reaction. The role of this ammonium salt is a kind of mold to form zeolite holes of a fixed size. When the ammonium salt is removed by calcination at 500 °C, the zeolite structure remains.
(d) Nitrogen oxides
A variety of nitrogen oxides will be described sequentially from lower to higher oxidation numbers (Table \(\PageIndex{4}\)).
1 | 2 | 12 | 13 | 14 | 15 | 16 | 17 | 18 | |
---|---|---|---|---|---|---|---|---|---|
2 | Li2O | BeO | B2O3 | CO CO2 |
N2O NO NO2 |
||||
3 | Na2O Na2O2 NaO2 |
MgO | Al2O3 | SiO2 | P4O6 P4O10 |
SO2 SO3 |
Cl2O ClO2 |
||
4 | K2O K2O2 KO2 |
CaO | ZnO | Ga2O3 | GeO2 | As4O6 As4O10 |
SeO2 SeO3 |
||
5 | Rb2O Rb2O2 Rb9O2 |
SrO | CdO | In2O3 | SnO2 | Sb4O6 Sb4O10 |
TeO2 TeO3 |
I2O5 | XeO3 XeO4 |
6 | Cs2O Cs11O3 |
BaO | HgO | Tl2O Tl2O3 |
PbO PbO2 |
Bi2O3 |
Dinitrogen monoxide, N2O
Oxide of monovalent nitrogen. Pyrolysis of ammonium nitrate generates this oxide as follows.
\[NH_{4}NO_{3} \xrightarrow{250 \;^{o} C} N_{2} O + 2 H_{2} O \nonumber \]
Although the oxidation number is a formality, it is an interesting and symbolic aspect of the versatility of the oxidation number of nitrogen that NH4NO3 forms a monovalent nitrogen oxide (+1 is a half of the average of -3 and +5 for NH4 and NO3, respectively). The N-N-O bond distances of the straight N2O are 112 pm (N-N) and 118 pm (N-O), corresponding to 2.5th and 1.5th bond order, respectively. N2O (16e) is isoelectronic with carbon dioxide CO2 (16e). This compound is also called laughing gas and is widely used for analgesia.
Nitric oxide, NO
An oxide of divalent nitrogen. This is obtained by reduction of nitrite as follows.
\[KNO_{2} + KI + H_{2}SO_{4} \rightarrow NO + K_{2}SO_{4} + H_{2} O + \frac{1}{2} I_{2} \nonumber \]
Having an odd number of valence electrons (11 electrons), it is paramagnetic. The N-O distance is 115 pm and the bond has double bond character. The unpaired electron in the highest antibonding \(\pi^{*}\) orbital is easily removed, and NO becomes NO+ (nitrosonium), which is isoelectronic with CO. Since an electron is lost from the antibonding orbital, the N-O bond becomes stronger. The compounds NOBF4 and NOHSO4 containing this cation are used as 1 electron oxidants.
Although NO is paramagnetic as a monomer in the gas phase, dimerization in the ondensed phase leads to diamagnetism. It is unique as a ligand of transition metal complexes and forms complexes like [Fe(CO)2(NO)2], in which NO is a neutral 3-electron ligand. Although M-N-O is straight in these kind of complexes, the M-N-O angle bends to 120° ~ 140° in [Co(NH3)5NO] Br2, in which NO- coordinates as a 4-electron ligand. It has become clear recently that nitric oxide has various biological control functions, such as blood-pressure depressing action, and it attracts attention as the second inorganic material after Ca2+ to play a role in signal transduction.
Dinitrogen trioxide, N2O3
The oxidation number of nitrogen is +3, and this is an unstable compound decomposing into NO and NO2 at room temperature. It is generated when equivalent quantities of NO and NO2 are condensed at low temperatures. It is light blue in the solid state and dark blue in the liquid state but the color fades at higher temperatures.
Nitrogen dioxide, NO2
A nitrogen compound with oxidation number +4. It is an odd electron compound with an unpaired electron, and is dark reddish brown in color. It is in equilibrium with the colorless dimer dinitrogen tetroxide, N2O4. The proportion of NO2 is 0.01% at -11 °C, and it increases gradually to 15.9% at its boiling point (21.2 °C), and becomes 100% at 140 °C.
N2O4 can be generated by the pyrolysis of lead nitrate as follows.
\[2 Pb(NO_{3})_{2} \xrightarrow{400 \;^{o} C} 4 NO_{2} + 2 PbO + O_{2} \nonumber \]
When NO2 is dissolved in water, nitric acid and nitrous acid are formed.
\[2 NO_{2} + H_{2}O \rightarrow HNO_{3} + HNO_{2} \nonumber \]
By one electron oxidation, NO2+ (nitroyl) forms and the O-N-O angle changes from 134° to 180° in the neutral NO2. On the other hand, by one electron reduction, NO2- (nitrito) forms and the angle bends to 115°.
Dinitrogen pentoxide, N2O5, is obtained when concentrated nitric acid is carefully dehydrated with phosphorus pentoxide at low temperatures. It sublimes at 32.4 °C. As it forms nitric acid by dissolving in water, it may also be called a nitric anhydride.
\[N_{2} O_{5} + H_{2} O \rightarrow 2 HNO_{3} \nonumber \]
Although it assumes an ion-pair structure NO2NO3 and straight NO2+ and planar NO3- ions are located alternately in the solid phase, it is molecular in the gas phase.
Oxoacids
Oxyacids of nitrogen include nitric acid, HNO3, nitrous acid, HNO2, and hyponitrous acid, H2N2O2. Nitric acid, HNO3, is one of the most important acids in the chemical industry, along with sulfuric acid and hydrochloric acid. Nitric acid is produced industrially by the Ostwald process, which is the oxidation reaction of ammonia in which the oxidation number of nitrogen increases from -3 to +5. Because the Gibbs energy of the direct conversion of dinitrogen to the intermediate NO2 is positive, and therefore the reaction is unfavorable thermodynamically, dinitrogen is firstly reduced to ammonia, and this is then oxidized to NO2.
\[\stackrel{0}{N_{2}} \rightarrow \stackrel{-3}{NH_{3}} \rightarrow \stackrel{+4}{NO_{2}} \rightarrow \stackrel{+5}{HNO_{3}} \nonumber \]
Nitric acid, HNO3
Commercial nitric acid is a ca.70% aqueous solution and vacuum distillation of it in the presence of phosphorus pentoxide gives pure nitric acid. As it is a srong oxidizing agent while also being a strong acid, it can dissolve metals (copper, silver, lead, etc.) which do not dissolve in other acids. Gold and platinum can even be dissolved in a mixture of nitric acid and hydrochloric acid (aqua regia). The nitrate ion, NO3-, and nitrite ion, NO2-, take various coordination forms when they coordinate as ligands in transition metal complexes.
Nitrous acid, HNO2
Although not isolated as a pure compound, aqueous solutions are weak acids (pKa = 3.15 at 25 °C) and important reagents. Since NaNO2 is used industrially for hydroxylamine (NH2OH) production and also used for diazotidation of aromatic amines, it is important for the manufacture of azo dyes and drugs. Among the various coordination forms of NO2- isomers now known, monodentate nitro (N-coordination) and nitrito (O-coordination) ligands had already been discovered in the 19th century.
(e) Phosphorus oxides
The structures of the phosphorus oxides P4O10, P4O9, P4O7, and P4O6 have been determined.
Phosphorus pentoxide, P4O10, is a white crystalline and sublimable solid that is formed when phosphorus is oxidized completely. Four phosphorus atoms form a tetrahedron and they are bridged by oxygen atoms (refer to Figure \(\PageIndex{12}\)). Since a terminal oxygen atom is bonded to each phosphorus atom, the coordination polyhedron of oxygen is also a tetrahedron. When the molecular P4O10 is heated, a vitrified isomer is formed. This is a polymer composed of similar tetrahedra of phosphorus oxide with the same composition that are connected to one another in sheets. Since it is very reactive with water, phosphorus pentoxide is a powerful dehydrating agent. It is used not only as a desiccant, but also it has remarkable dehydration properties, and N2O5 or SO3 can be formed by dehydration of HNO3 or H2SO4, respectively. Phosphorus pentoxide forms orthophosphoric acid, H3PO4, when reacted with sufficient water, but if insufficient water is used, various kinds of condensed phosphoric acids are produced depending on the quantity of reacting water.
Phosphorus trioxide, P4O6, is a molecular oxide, and its tetrahedral structure results from the removal of only the terminal oxygen atoms from phosphorus pentoxide. Each phosphorus is tri-coordinate. This compound is formed when white phosphorus is oxidized at low temperatures in insufficient oxygen. The oxides with compositions intermediate between phosphorus pentoxide and trioxide have 3 to 1 terminal oxygen atoms and their structures have been analyzed.
Although arsenic and antimony give molecular oxides As4O6 and Sb4O6 that have similar structures to P4O6, bismuth forms a polymeric oxide of composition Bi2O3.
Phosphoric acid
Orthophosphoric acid, H3PO4
It is one of the major acids used in chemical industry, and is produced by the hydration reaction of phosphorus pentoxide, P4O10. Commercial phosphoric acid is usually of 75-85% purity. The pure acid is a crystalline compound (mp 42.35 °C). One terminal oxygen atom and three OH groups are bonded to the phosphorus atom in the center of a tetrahedron. The three OH groups release protons making the acid tribasic (pK1 = 2.15). When two orthophosphoric acid molecules condense by the removal of an H2O molecule, pyrophosphoric acid, H4P2O7, is formed.
Phosphonic acid, H3PO3
This acid is also called phosphorous acid and has H in place of one of the OH groups of orthophosphoric acid. Since there are only two OH groups, it is a dibasic acid.
Phosphinic acid, H3PO2
It is also called hypophosphorous acid, and two of the OH groups in orthophosphoric acid are replaced by H atoms. The remaining one OH group shows monobasic acidity. If the PO4 tetrahedra in the above phosphorus acids bind by O bridges, many condensed phosphoric acids form. Adenosine triphosphate (ATP), deoxyribonucleic acid (DNA), etc., in which the triphosphorus acid moieties are combined with adenosine are phosphorus compounds that are fundamentally important for living organisms.
(f) Sulfur oxides
Sulfur dioxide, SO2
This is formed by the combustion of sulfur or sulfur compounds. This is a colorless and poisonous gas (bp -10.0 °C) and as an industrial emission is one of the greatest causes of environmental problems. However, it is very important industrially as a source material of sulfur. Sulfur dioxide is an angular molecule, and recently it has been demonstrated that it takes various coordination modes as a ligand to transition metals. It is a nonaqueous solvent similar to liquid ammonia, and is used for special reactions or as a solvent for special NMR measurements.
Sulfur trioxide, SO3
It is produced by catalytic oxidation of sulfur dioxide and used for manufacturing sulfuric acid. The usual commercial reagent is a liquid (bp 44.6 °C). The gaseous phase monomer is a planar molecule. It is in equilibrium with a ring trimer (\(\gamma\)-SO3 = S3O9) in the gaseous or liquid phase. In the presence of a minute amount of water SO3 changes to \(\beta\)-SO3, which is a crystalline high polymer with a helical structure. \(\alpha\)-SO3 is also known as a solid of still more complicated lamellar structure. All react violently with water to form sulfuric acid.
Sulfur acids
Although there are many oxy acids of sulfur, most of them are unstable and cannot be isolated. They are composed of a combination of S=O, S-OH, S-O-S, and S-S bonds with a central sulfur atom. As the oxidation number of sulfur atoms varies widely, various redox equilibria are involved.
Sulfuric acid, H2SO4
It is an important basic compound produced in the largest quantity of all inorganic compounds. Pure sulfuric acid is a viscous liquid (mp 10.37 °C), and dissolves in water with the generation of a large amount of heat to give strongly acidic solutions.
Thiosulfuric acid, H2S2O3
Although it is generated if thiosulfate is acidified, the free acid is unstable. The S2O32- ion is derived from the replacement of one of the oxygen atoms of SO42- by sulfur, and is mildly reducing.
Sulfurous acid, H2SO3
The salt is very stable although the free acid has not been isolated. The SO32- ion that has pyramidal C3v symmetry is a reducing agent. In dithionic acid, H2S2O6, and the dithionite ion S2O62-, the oxidation number of sulfer is +5, and one S-S bond is formed. This is a very strong reducing agent.
(g) Metal oxides
Oxides of all the metallic elements are known and they show a wide range of properties in terms of structures, acidity and basicity, and conductivity. Namely, an oxide can exhibit molecular, 1-dimensional chain, 2-dimensional layer, or 3-dimensional structures. There are basic, amphoteric, and acidic oxides depending on the identity of the metallic element. Moreover, the range of physical properties displayed is also broad, from insulators, to semicondutors, metallic conductors, and superconductors. The compositions of metallic oxide can be simply stoichiometric, stoichiometric but not simple, or sometimes non-stoichiometric. Therefore, it is better to classify oxides according to each property. However, since structures give the most useful information to understand physical and chemical properties, typical oxides are classified first according to the dimensionality of their structures (Table \(\PageIndex{4}\), Table \(\PageIndex{5}\)).
Oxidation number | 3 | 4 | 5 | 6 | 7 | 8 | 9 | 10 | 11 |
---|---|---|---|---|---|---|---|---|---|
+1 | Ti2Ol | Cu2O Ag2O |
|||||||
+2 | TiO | VO NbO |
MnO | FeO | CoO | NiO | CuO Ag2O2 |
||
+3 | Sc2O3 Y2O3 |
Ti2O3 | V2O3 | Cr2O3 | Fe2O3 | Rh2O3 | |||
+4 | TiO2 ZrO2 HfO2 |
VO2 NbO2 TaO2 |
CrO2 MoO2 WO2 |
MnO2 TcO2 ReO2 |
RuO2 OsO2 |
RhO2 IrO2 |
PtO2 | ||
+5 | V2O5i Nb2O5 Ta2O5 |
||||||||
+6 | CrO3c MoO3l WO3 |
ReO3 | |||||||
+7 | Re2O7l | ||||||||
+8 | RuO4 m OsO4m |
m molecular, c chain, l layer, others 3-demensional.