12.9.1: Metal-catalyzed Hydrolysis
- Page ID
- 385559
Transition metal ions (but not alkali metal ions) act as Lewis acids in metal-ligand interactions. As a consequence of the metal ion interacting with the ligand, the electronic structure of the ligand is altered. In some cases, the metal-ligand interaction causes changes in the strength and reactivity of other bonds within the ligand itself. For example, when a ligand has an acidic proton, interactions with a metal ion will make that acidic proton more acidic. In other words, interaction with the metal ion can cause a decrease in the ligand's \(pK_a\). The metal-ligand complex itself can act as a stronger Bronsted acid (giving off a proton) compared to the "free" ligand. We can see this type of alteration in the \(pK_a\) of water when it is "free" compared to when it is bound to a metal ion.
Example: Water meets a transition metal ion1
In biology, the solvent is water. Water is also a ligand that binds to metal ions, according to the equation shown below.
\[\ce{nH2O + M^{x+} <=>> [M(H2O)_{n}]^{x+}} \nonumber \]
There is a LOT of water around in biological systems. Consequently, the reaction shown above is shifted far to the right (toward the aquo metal complex, \(\ce{[M(H2O)_{n}]^{x+}}\)) in aqueous solution. Consequently, when metals are dissolved in aqueous solution, the "free" ion does not exist, and rather the metal is in complex with water. This is generally true unless there are other ligands present that have stronger affinity for the metal ion.
Consider the two equilibrium reactions shown below. The autoionization of water is shown on the left. This reaction has a very small equilibrium constant ( \(K_W = 10^{-14}\)) and thus the \(pK_a\) for dissociation of a proton from water is \(pK_a = 14\). On the left is the dissociation of a proton from a water molecule bound to \(\ce{Zn^2+}\) ion (other water ligands are not drawn for simplicity). The bond between \(\ce{H2O}\) and \(\ce{Zn}\) is considered to be covalent.
\[\ce{H2O <=> H^+ + OH^-} \quad \quad \text{vs.} \quad \quad \ce{[Zn(H2O)]^2+ <=> H^+ + [Zn(OH)]^+} \nonumber \]
Predict which reaction above is more favorable. In other words, in which case is water a better acid; when water is "free", or when it is bound to a transition metal ion like Zn2+? Defend your prediction.1
- Hint
-
Consider these two things:
(1) In which case is the hydroxide ion more stable: when it is a free ion or when it is bound to Zn? How will this effect one case relative to the other?
(2) Consider separation of charge in each equilibrium. Is the localization of positive and negative charge more energetically favorable in the reactands or in the products in each case? How will this effect one case relative to the other?
- Answer
-
Water is a better acid when it has a covalent bond to a metal ion. The metal-ligand interaction weakens the H-O bond and stabilizes the conjugate base that forms after deprotonation.
Water has a \(pK_a\) of 14. When water is bound to a Zn2+ ion in aqueous solution, its \(pK_a\) is lowered to 10. Calculate the pH of pure water and the pH of a solution of 0.1 M ZnSO4.
- Hint
-
You will need the chemical equations above and you will need to recall the definitions of \(K_a\) and \(pK_a\), as well as \(pH\) to complete this task. Recall that \(pK_a = - \log K_a\) and \(pH = - \log [H^+]\). The \(K_a\) is the equilibrium constant for the chemical equation. Use an I.C.E. table to calculate the amount of hydrogen ion at equilibrium.
- Answer
-
The pH of pure water is 7. The pH of 0.1 M \(\ce{ZnSO4}\) depends only on the concentration of the Zn aquo complex that forms after dissociation of \(\ce{ZnSO4}\) to \(\ce{Zn^2+}\) and \(\ce{SO4^2-}\). We can use the I.C.E method to determine that the concentration of hydrogen ion at equilibrium is approximately \(3.2 \times 10^{-6}\) after 0.1M \(\ce{Zn^2+}\) is initially added to water. This gives approximately \(pH = 5.5\).
Transition metals dramatically decrease solution pH compared to pure water. On the other hand, alkali metals do not significantly affect pH.
a) Based on this information, what can you conclude about the relative covalent nature of water’s interactions with transition metals compared to alkali metals?
b) Rationalize this based on the element's position on the periodic table and their consequent electron configurations.
- Answer
-
a) transition metals form more covalent bonds with water, while alkali metal's interactions are more electrostatic in character.
b) transition metals have partially-filled d subshells, while alkali metals have the electron configurations of the nobel gases. Perhaps the nobel gas electron configuration makes covalent bonding unfavorable, while partially filled d-orbitals enables productive bonding interactions with ligands.
When a transition metal binds to a Cys side chain, it usually binds to the thiolate anion form of this side chain. Explain why the Cys loses its proton when a metal ion binds. Use drawings to describe what happens to the \(pK_a\) of the thiol hydrogen.
- Hint
-
A cystein side chain has a thiol (R-SH) group. Recall that thiols behave similarly to alcohols, but thiols are more acidic than alcohols.
- Answer
-
Bonding of a cystein S to a metal ion would weaken the S-H bond and increase the acidity of that proton (it's pKa would decrease). In bilogical conditions, near neutral pH, this bonding could decrease the pKa of the thiol group so much that the H is lost to give the conjugate base thiolate bound to the metal.
Biological hydrolysis2
Above you learned how metals can act as Lewis Acids to increase the acidity of a bound water molecule (or other ligand). This modulation of water's \(pK_a\) can be really useful to catalyze reactions in which hydroxide ion is a reactant. The enzyme carbonic anhydrase (CA) is a prime example of how biology uses this property to catalyze an important biological acid/base reaction. CA is the enzyme that converts \(\ce{CO2}\) into bicarbonate (\(\ce{HCO3^-}\)) very quickly. This is important in the blood to get to \(\ce{CO2}\) to the lungs to be exhaled. Conversion of \(\ce{CO2}\) to bicarbonate is also important in production of the aqueous fluid of eye and other secretions; and CA is an important drug target for treatment of glaucoma.
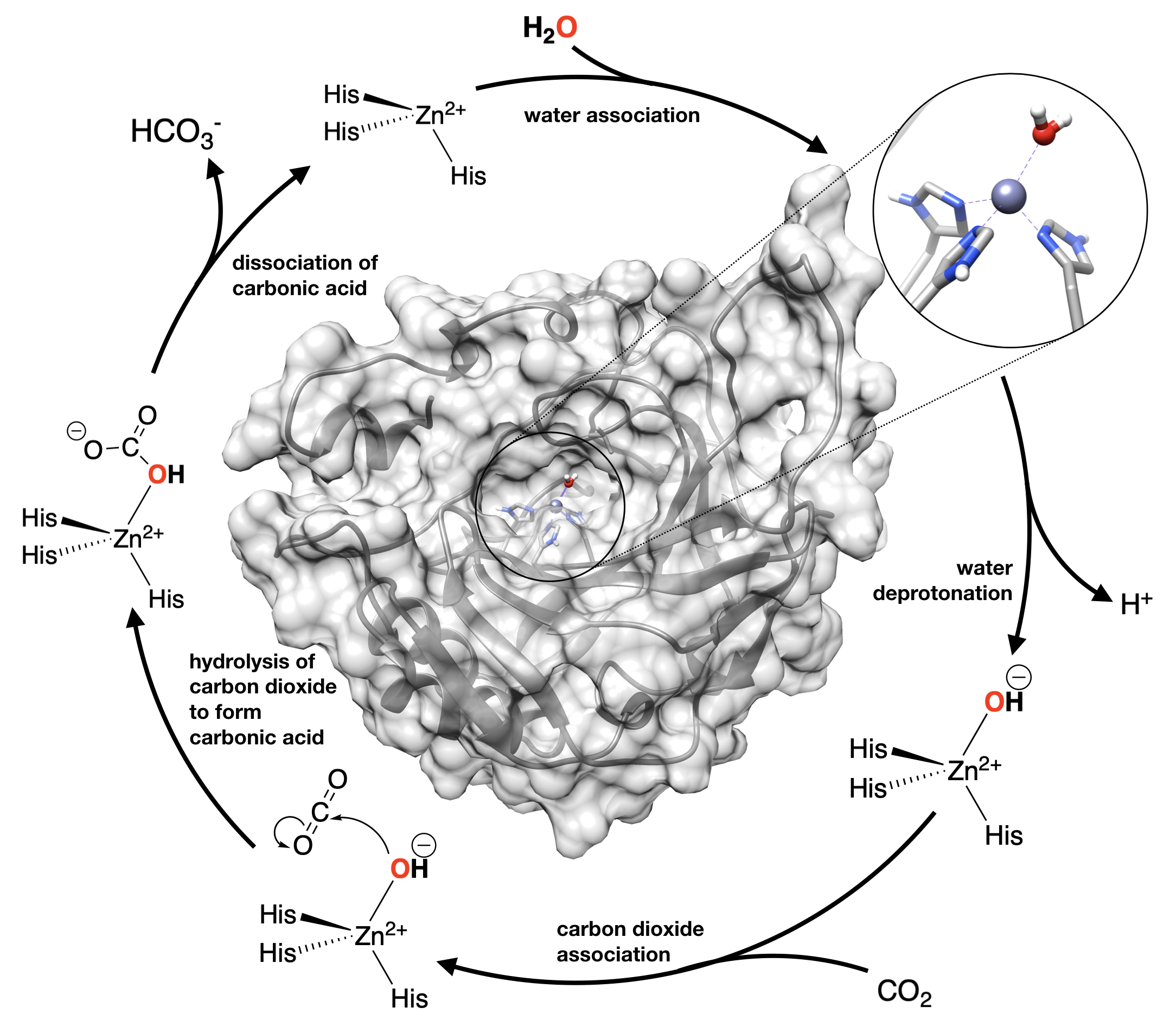
The figure shows the structure of human carbonic anhydrase IV (from PDB 1znc), with a blow up of the Zn active site where Zn is bound to 3 histidine side chains (His). The mechanism for this enzyme is shown in the chemical equations circling the structure in Figure \(\PageIndex{4}\). As we discused above, binding water to a metal ion can change its \(pK_a\), and make its protons more acidic by stabilizing the hydroxide ion. When hydroxide is needed to carry out hydrolysis reactions, acidic metal ions, like Zn2+ are often used to stabilize the hydroxide nucleophile in order to catalyze the reaction (lower the activation energy). The \(pK_a\) of “free” water is 14. For water in [Zn(H2O)6]2+ in aqueous solution, the \(pK_a\) is 10. But, in the active site of carbonic anhydrase, the \(pK_a\) of water is lowered even more, to 7, by the protein environment around the Zn active site. The active site of this protein has the "power" to lower the pKa of water even more than in the case of aqueous Zn as a virtue of the hydrophobic pocket of the active site that makes charge separation more unstable compared to bulk water where charges can be better solubilize by bulk water.
Look carefully at the catalytic cycle shown in Figure \(\PageIndex{4}\) and identify the step in which water looses a proton (the step labeled "water deprotonation". This step would be nearly impossible under biological conditions at pH 7.4. In other words, it would happen too infrequently to be useful in biological systems. But, when the pKa is lowered to 7, which is below the pH, that step becomes so favorable, that it would happen spontaneously in solution.
Carbonic anhydrase is just one example of how metal ions can be useful for catalyzing hydrolysis reactions. There are several more examples of catalytic enzymes and small molecule metal complexes that use metals (Cu, Co, Ni, Mn, Ca, and Mg) to catalyze hydrolysis of esters (eg hydrolysis of fats), amides (eg hydrolysis of peptide bonds with water), phosphate esters (eg hydrolysis of DNA and RNA) using mechanisms simlar to that shown above for hydrolysis of carbon dioxide.
Sources
1. Inspired by or taken directly from Metals in Acid Base Chemistry, an in-class activity created by Sheila Smith, University of Michigan- Dearborn (sheilars@umd.umich.edu) and posted on VIPEr (www.ionicviper.org) on October 17, 2009. Copyright Sheila Smith 2009. This work is licensed under the Creative Commons Attribution Non-commercial Share Alike License. To view a copy of this license visit http://creativecommons.org/about/license/.
2. From Metals in Biological Systems - Who? How? and Why?, a 5 slides about learning object created by Adam R. Johnson, Harvey Mudd College (adam_johnson@hmc.edu), Hilary Eppley, DePauw Univeristy (), and Sheila Smith, University of Michigan- Dearborn (sheilars@umd.umich.edu) and posted on VIPEr (www.ionicviper.org) on January 20, 2010. Copyright Sheila Smith 2009. This work is licensed under the Creative Commons Attribution Non-commercial Share Alike License. To view a copy of this license visit http://creativecommons.org/about/license/.