11.3.8: Applications of Charge-Transfer
- Page ID
- 377935
\( \newcommand{\vecs}[1]{\overset { \scriptstyle \rightharpoonup} {\mathbf{#1}} } \)
\( \newcommand{\vecd}[1]{\overset{-\!-\!\rightharpoonup}{\vphantom{a}\smash {#1}}} \)
\( \newcommand{\id}{\mathrm{id}}\) \( \newcommand{\Span}{\mathrm{span}}\)
( \newcommand{\kernel}{\mathrm{null}\,}\) \( \newcommand{\range}{\mathrm{range}\,}\)
\( \newcommand{\RealPart}{\mathrm{Re}}\) \( \newcommand{\ImaginaryPart}{\mathrm{Im}}\)
\( \newcommand{\Argument}{\mathrm{Arg}}\) \( \newcommand{\norm}[1]{\| #1 \|}\)
\( \newcommand{\inner}[2]{\langle #1, #2 \rangle}\)
\( \newcommand{\Span}{\mathrm{span}}\)
\( \newcommand{\id}{\mathrm{id}}\)
\( \newcommand{\Span}{\mathrm{span}}\)
\( \newcommand{\kernel}{\mathrm{null}\,}\)
\( \newcommand{\range}{\mathrm{range}\,}\)
\( \newcommand{\RealPart}{\mathrm{Re}}\)
\( \newcommand{\ImaginaryPart}{\mathrm{Im}}\)
\( \newcommand{\Argument}{\mathrm{Arg}}\)
\( \newcommand{\norm}[1]{\| #1 \|}\)
\( \newcommand{\inner}[2]{\langle #1, #2 \rangle}\)
\( \newcommand{\Span}{\mathrm{span}}\) \( \newcommand{\AA}{\unicode[.8,0]{x212B}}\)
\( \newcommand{\vectorA}[1]{\vec{#1}} % arrow\)
\( \newcommand{\vectorAt}[1]{\vec{\text{#1}}} % arrow\)
\( \newcommand{\vectorB}[1]{\overset { \scriptstyle \rightharpoonup} {\mathbf{#1}} } \)
\( \newcommand{\vectorC}[1]{\textbf{#1}} \)
\( \newcommand{\vectorD}[1]{\overrightarrow{#1}} \)
\( \newcommand{\vectorDt}[1]{\overrightarrow{\text{#1}}} \)
\( \newcommand{\vectE}[1]{\overset{-\!-\!\rightharpoonup}{\vphantom{a}\smash{\mathbf {#1}}}} \)
\( \newcommand{\vecs}[1]{\overset { \scriptstyle \rightharpoonup} {\mathbf{#1}} } \)
\( \newcommand{\vecd}[1]{\overset{-\!-\!\rightharpoonup}{\vphantom{a}\smash {#1}}} \)
\(\newcommand{\avec}{\mathbf a}\) \(\newcommand{\bvec}{\mathbf b}\) \(\newcommand{\cvec}{\mathbf c}\) \(\newcommand{\dvec}{\mathbf d}\) \(\newcommand{\dtil}{\widetilde{\mathbf d}}\) \(\newcommand{\evec}{\mathbf e}\) \(\newcommand{\fvec}{\mathbf f}\) \(\newcommand{\nvec}{\mathbf n}\) \(\newcommand{\pvec}{\mathbf p}\) \(\newcommand{\qvec}{\mathbf q}\) \(\newcommand{\svec}{\mathbf s}\) \(\newcommand{\tvec}{\mathbf t}\) \(\newcommand{\uvec}{\mathbf u}\) \(\newcommand{\vvec}{\mathbf v}\) \(\newcommand{\wvec}{\mathbf w}\) \(\newcommand{\xvec}{\mathbf x}\) \(\newcommand{\yvec}{\mathbf y}\) \(\newcommand{\zvec}{\mathbf z}\) \(\newcommand{\rvec}{\mathbf r}\) \(\newcommand{\mvec}{\mathbf m}\) \(\newcommand{\zerovec}{\mathbf 0}\) \(\newcommand{\onevec}{\mathbf 1}\) \(\newcommand{\real}{\mathbb R}\) \(\newcommand{\twovec}[2]{\left[\begin{array}{r}#1 \\ #2 \end{array}\right]}\) \(\newcommand{\ctwovec}[2]{\left[\begin{array}{c}#1 \\ #2 \end{array}\right]}\) \(\newcommand{\threevec}[3]{\left[\begin{array}{r}#1 \\ #2 \\ #3 \end{array}\right]}\) \(\newcommand{\cthreevec}[3]{\left[\begin{array}{c}#1 \\ #2 \\ #3 \end{array}\right]}\) \(\newcommand{\fourvec}[4]{\left[\begin{array}{r}#1 \\ #2 \\ #3 \\ #4 \end{array}\right]}\) \(\newcommand{\cfourvec}[4]{\left[\begin{array}{c}#1 \\ #2 \\ #3 \\ #4 \end{array}\right]}\) \(\newcommand{\fivevec}[5]{\left[\begin{array}{r}#1 \\ #2 \\ #3 \\ #4 \\ #5 \\ \end{array}\right]}\) \(\newcommand{\cfivevec}[5]{\left[\begin{array}{c}#1 \\ #2 \\ #3 \\ #4 \\ #5 \\ \end{array}\right]}\) \(\newcommand{\mattwo}[4]{\left[\begin{array}{rr}#1 \amp #2 \\ #3 \amp #4 \\ \end{array}\right]}\) \(\newcommand{\laspan}[1]{\text{Span}\{#1\}}\) \(\newcommand{\bcal}{\cal B}\) \(\newcommand{\ccal}{\cal C}\) \(\newcommand{\scal}{\cal S}\) \(\newcommand{\wcal}{\cal W}\) \(\newcommand{\ecal}{\cal E}\) \(\newcommand{\coords}[2]{\left\{#1\right\}_{#2}}\) \(\newcommand{\gray}[1]{\color{gray}{#1}}\) \(\newcommand{\lgray}[1]{\color{lightgray}{#1}}\) \(\newcommand{\rank}{\operatorname{rank}}\) \(\newcommand{\row}{\text{Row}}\) \(\newcommand{\col}{\text{Col}}\) \(\renewcommand{\row}{\text{Row}}\) \(\newcommand{\nul}{\text{Nul}}\) \(\newcommand{\var}{\text{Var}}\) \(\newcommand{\corr}{\text{corr}}\) \(\newcommand{\len}[1]{\left|#1\right|}\) \(\newcommand{\bbar}{\overline{\bvec}}\) \(\newcommand{\bhat}{\widehat{\bvec}}\) \(\newcommand{\bperp}{\bvec^\perp}\) \(\newcommand{\xhat}{\widehat{\xvec}}\) \(\newcommand{\vhat}{\widehat{\vvec}}\) \(\newcommand{\uhat}{\widehat{\uvec}}\) \(\newcommand{\what}{\widehat{\wvec}}\) \(\newcommand{\Sighat}{\widehat{\Sigma}}\) \(\newcommand{\lt}{<}\) \(\newcommand{\gt}{>}\) \(\newcommand{\amp}{&}\) \(\definecolor{fillinmathshade}{gray}{0.9}\)Transition metal complexes with strong \(\pi\)-accepting ligands have proven quite useful across many applications in industry, materials science, and medicine due to their intense absorption of light, photostability, and unique geometric, redox, and photodynamic properties. These complexes usually have low-valent metal ions (low oxidation state) with \(\pi\)-accepting ligands constructed of conjugated poly-aromatic rings. Intense light absorption occurs through metal-to-ligand charge transfer (MLCT), causing a formal oxidation of the metal ion and reduction of the ligand. These compounds are used as sensors, and their excited states can be used as indispensable reactants. Some examples of their applications are discussed briefly below.
Ruthenium bypyridine derivatives
Let's use the example of a simple and interesting metal complex: tris(bypyridine)ruthenium(II), or \(\ce{[Ru(bpy)_3]^2+}\). This molecule is the most generic form of a family of derivatives that have this core structure in common. \(\ce{[Ru(bpy)_3]^2+}\) absorbs ultraviolet light at 452 nm due to an intense MLCT. It also absorbs light at shorter wavelenghts due to ligand-centered \(\pi * \leftarrow \pi\), metal-centered \(d \leftarrow d\), and LMCT transitions. The ground state is a singlet state (all paired electrons), and the spin-selection rule allows formation of a singlet excited state. However, in the case of high-atomic-number \(d^6\) metal ions, like Ru, there is intense spin-orbit coupling that favors intersystem crossing. Intersystem crossing is the phenomenon by which a system's spin multiplicity can change by the transition of an electron's spin without energy cost. In the case of \(\ce{[Ru(bpy)_3]^2+}\), the excited singlet state transitions to an isoenergetic (or degenerate) triplet excited state. \(\ce{[Ru(bpy)_3]^2+}\) has a long-lived excited state due to the fact that the transition between the triplet excited state and the singlet ground state is spin-forbidden. The excited state can relax to the singlet ground state radiatively (releasing a photon) or non-radiatively (as phonons/heat).
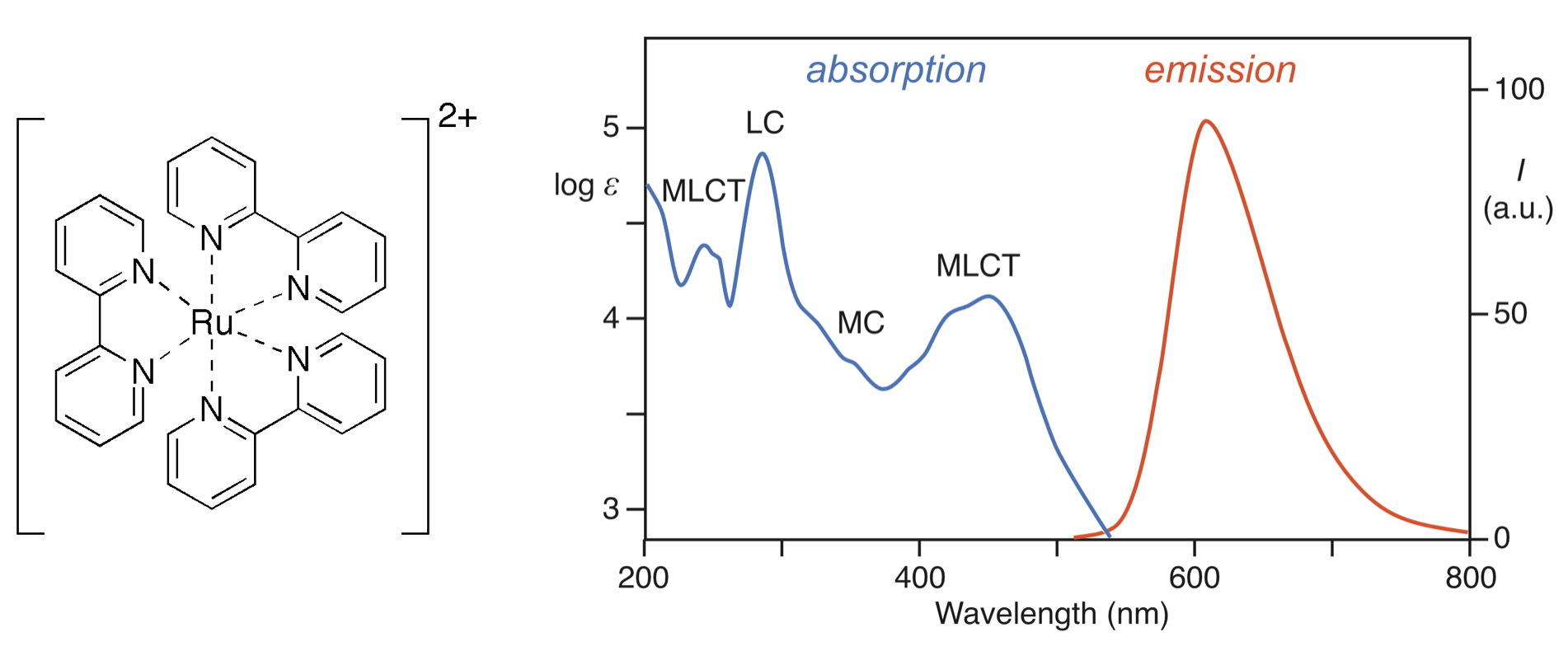
Bioimaging
The \(\ce{[Ru(bpy)_3]^2+}\) complex is fluorescent, with a quantum yeld of approximately 2%. Derivatives of this core structure offer a variety of interesting properties, including a tunable color of emission. Other modifications have been made to make Ru bipyridine derivatives more functional for cellular imaging. For example, these metal complexes have been modified to bind to carbohydrates, specific organelles, or specific organs for targeted biological imaging applications.
Energy and catalysis
Due to the long lifetime of the \(\ce{[Ru(bpy)_3]^2+}*\) excited triplet state, this molecule has potential as a photosensitizer for the oxidation and reduction of water (water splitting) for energy production. The MLCT excitation is essentially a removal of an electron from Ru (an oxidation of Ru) and a placement of an electron onto a bpy ligand (a reduction of the ligand). The resultant \(\ce{[Ru^3+]}\) center is a powerful oxidant, capable of oxidizing water, while the unpaired electron on reduced bpy is a powerful reductant. The power of this complex for catalyzing the splitting of water has been demonstrated using an electron mediator, methyl viologin, a Pt catalyst, and a sacrificial reductant. Once the excited \(\ce{[Ru(bpy)_3]^2+}*\) has formed, the excited electron can be captured by methyl viologen and passed to a Pt catalyst which reduces hydrogen ion (\(\ce{H^+}\)) to \(\ce{H_2}\). The oxidized \(\ce{[Ru(bpy)_3]^3+}\) complex is then reduced by reaction with a sacrificial reductant like EDTA or triethanolamine to re-form \(\ce{[Ru(bpy)_3]^2+}\).
\(\ce{[Ru(bpy)_3]^2+}\) is also used as a photosensitizer for organic synthesis. Many analogues of \(\ce{[Ru(bpy)_3]^2+}\) are employed as well. These transformations exploit the redox properties of excited \(\ce{[Ru(bpy)_3]^2+}*\) and its reductively quenched derivative \(\ce{[Ru(bpy)_3]^+}\). (from Wikipedia)
Other applications
Derivatives of \(\ce{[Ru(bpy)_3]^2+}\) are numerous. Such complexes are widely discussed for applications in biodiagnostics, photovoltaics, and organic light-emitting diodes, but no derivative has been commercialized. Application of \(\ce{[Ru(bpy)_3]^2+}\) and its derivatives to fabrication of optical chemical sensors is arguably one of the most successful areas so far. (from Wikipedia)