16.9: Acid-Base Properties of Salt Solutions
- Page ID
- 25233
\( \newcommand{\vecs}[1]{\overset { \scriptstyle \rightharpoonup} {\mathbf{#1}} } \)
\( \newcommand{\vecd}[1]{\overset{-\!-\!\rightharpoonup}{\vphantom{a}\smash {#1}}} \)
\( \newcommand{\id}{\mathrm{id}}\) \( \newcommand{\Span}{\mathrm{span}}\)
( \newcommand{\kernel}{\mathrm{null}\,}\) \( \newcommand{\range}{\mathrm{range}\,}\)
\( \newcommand{\RealPart}{\mathrm{Re}}\) \( \newcommand{\ImaginaryPart}{\mathrm{Im}}\)
\( \newcommand{\Argument}{\mathrm{Arg}}\) \( \newcommand{\norm}[1]{\| #1 \|}\)
\( \newcommand{\inner}[2]{\langle #1, #2 \rangle}\)
\( \newcommand{\Span}{\mathrm{span}}\)
\( \newcommand{\id}{\mathrm{id}}\)
\( \newcommand{\Span}{\mathrm{span}}\)
\( \newcommand{\kernel}{\mathrm{null}\,}\)
\( \newcommand{\range}{\mathrm{range}\,}\)
\( \newcommand{\RealPart}{\mathrm{Re}}\)
\( \newcommand{\ImaginaryPart}{\mathrm{Im}}\)
\( \newcommand{\Argument}{\mathrm{Arg}}\)
\( \newcommand{\norm}[1]{\| #1 \|}\)
\( \newcommand{\inner}[2]{\langle #1, #2 \rangle}\)
\( \newcommand{\Span}{\mathrm{span}}\) \( \newcommand{\AA}{\unicode[.8,0]{x212B}}\)
\( \newcommand{\vectorA}[1]{\vec{#1}} % arrow\)
\( \newcommand{\vectorAt}[1]{\vec{\text{#1}}} % arrow\)
\( \newcommand{\vectorB}[1]{\overset { \scriptstyle \rightharpoonup} {\mathbf{#1}} } \)
\( \newcommand{\vectorC}[1]{\textbf{#1}} \)
\( \newcommand{\vectorD}[1]{\overrightarrow{#1}} \)
\( \newcommand{\vectorDt}[1]{\overrightarrow{\text{#1}}} \)
\( \newcommand{\vectE}[1]{\overset{-\!-\!\rightharpoonup}{\vphantom{a}\smash{\mathbf {#1}}}} \)
\( \newcommand{\vecs}[1]{\overset { \scriptstyle \rightharpoonup} {\mathbf{#1}} } \)
\( \newcommand{\vecd}[1]{\overset{-\!-\!\rightharpoonup}{\vphantom{a}\smash {#1}}} \)
\(\newcommand{\avec}{\mathbf a}\) \(\newcommand{\bvec}{\mathbf b}\) \(\newcommand{\cvec}{\mathbf c}\) \(\newcommand{\dvec}{\mathbf d}\) \(\newcommand{\dtil}{\widetilde{\mathbf d}}\) \(\newcommand{\evec}{\mathbf e}\) \(\newcommand{\fvec}{\mathbf f}\) \(\newcommand{\nvec}{\mathbf n}\) \(\newcommand{\pvec}{\mathbf p}\) \(\newcommand{\qvec}{\mathbf q}\) \(\newcommand{\svec}{\mathbf s}\) \(\newcommand{\tvec}{\mathbf t}\) \(\newcommand{\uvec}{\mathbf u}\) \(\newcommand{\vvec}{\mathbf v}\) \(\newcommand{\wvec}{\mathbf w}\) \(\newcommand{\xvec}{\mathbf x}\) \(\newcommand{\yvec}{\mathbf y}\) \(\newcommand{\zvec}{\mathbf z}\) \(\newcommand{\rvec}{\mathbf r}\) \(\newcommand{\mvec}{\mathbf m}\) \(\newcommand{\zerovec}{\mathbf 0}\) \(\newcommand{\onevec}{\mathbf 1}\) \(\newcommand{\real}{\mathbb R}\) \(\newcommand{\twovec}[2]{\left[\begin{array}{r}#1 \\ #2 \end{array}\right]}\) \(\newcommand{\ctwovec}[2]{\left[\begin{array}{c}#1 \\ #2 \end{array}\right]}\) \(\newcommand{\threevec}[3]{\left[\begin{array}{r}#1 \\ #2 \\ #3 \end{array}\right]}\) \(\newcommand{\cthreevec}[3]{\left[\begin{array}{c}#1 \\ #2 \\ #3 \end{array}\right]}\) \(\newcommand{\fourvec}[4]{\left[\begin{array}{r}#1 \\ #2 \\ #3 \\ #4 \end{array}\right]}\) \(\newcommand{\cfourvec}[4]{\left[\begin{array}{c}#1 \\ #2 \\ #3 \\ #4 \end{array}\right]}\) \(\newcommand{\fivevec}[5]{\left[\begin{array}{r}#1 \\ #2 \\ #3 \\ #4 \\ #5 \\ \end{array}\right]}\) \(\newcommand{\cfivevec}[5]{\left[\begin{array}{c}#1 \\ #2 \\ #3 \\ #4 \\ #5 \\ \end{array}\right]}\) \(\newcommand{\mattwo}[4]{\left[\begin{array}{rr}#1 \amp #2 \\ #3 \amp #4 \\ \end{array}\right]}\) \(\newcommand{\laspan}[1]{\text{Span}\{#1\}}\) \(\newcommand{\bcal}{\cal B}\) \(\newcommand{\ccal}{\cal C}\) \(\newcommand{\scal}{\cal S}\) \(\newcommand{\wcal}{\cal W}\) \(\newcommand{\ecal}{\cal E}\) \(\newcommand{\coords}[2]{\left\{#1\right\}_{#2}}\) \(\newcommand{\gray}[1]{\color{gray}{#1}}\) \(\newcommand{\lgray}[1]{\color{lightgray}{#1}}\) \(\newcommand{\rank}{\operatorname{rank}}\) \(\newcommand{\row}{\text{Row}}\) \(\newcommand{\col}{\text{Col}}\) \(\renewcommand{\row}{\text{Row}}\) \(\newcommand{\nul}{\text{Nul}}\) \(\newcommand{\var}{\text{Var}}\) \(\newcommand{\corr}{\text{corr}}\) \(\newcommand{\len}[1]{\left|#1\right|}\) \(\newcommand{\bbar}{\overline{\bvec}}\) \(\newcommand{\bhat}{\widehat{\bvec}}\) \(\newcommand{\bperp}{\bvec^\perp}\) \(\newcommand{\xhat}{\widehat{\xvec}}\) \(\newcommand{\vhat}{\widehat{\vvec}}\) \(\newcommand{\uhat}{\widehat{\uvec}}\) \(\newcommand{\what}{\widehat{\wvec}}\) \(\newcommand{\Sighat}{\widehat{\Sigma}}\) \(\newcommand{\lt}{<}\) \(\newcommand{\gt}{>}\) \(\newcommand{\amp}{&}\) \(\definecolor{fillinmathshade}{gray}{0.9}\)- To recognize salts that will produce acidic, basic, or neutral solutions in water
- To understand the Lewis acidity of small, highly-charged metal ions in water
A neutralization reaction can be defined as the reaction of an acid and a base to produce a salt and water. That is, another cation, such as \(Na^+\), replaces the proton on the acid. An example is the reaction of \(CH_3CO_2H\), a weak acid, with \(NaOH\), a strong base:
\[\underset{acid}{CH_3CO_2H_{(l)}} +\underset{base}{NaOH_{(s)}} \overset{H_2O}{\longrightarrow} \underset{salt}{H_2OCH_3CO_2Na_{(aq)} }+\underset{water}{H_2O_{(l)}} \nonumber \]
Depending on the acid–base properties of its component ions, however, a salt can dissolve in water to produce a neutral solution, a basic solution, or an acidic solution.
When a salt such as \(NaCl\) dissolves in water, it produces \(Na^+_{(aq)}\) and \(Cl^−_{(aq)}\) ions. Using a Lewis approach, the \(Na^+\) ion can be viewed as an acid because it is an electron pair acceptor, although its low charge and relatively large radius make it a very weak acid. The \(Cl^−\) ion is the conjugate base of the strong acid \(HCl\), so it has essentially no basic character. Consequently, dissolving \(NaCl\) in water has no effect on the \(pH\) of a solution, and the solution remains neutral.
Now let's compare this behavior to the behavior of aqueous solutions of potassium cyanide and sodium acetate. Again, the cations (\(K^+\) and \(Na^+\)) have essentially no acidic character, but the anions (\(CN^−\) and \(CH_3CO_2^−\)) are weak bases that can react with water because they are the conjugate bases of the weak acids \(HCN\) and acetic acid, respectively.
\[ CN^-_{(aq)} + H_2O_{(l)} \ce{ <<=>} HCN_{(aq)} + OH^-_{(aq)} \nonumber \]
\[ CH_3CO^2_{2(aq)} + H_2O_{(l)} \ce{<<=>} CH_3CO_2H_{(aq)} + OH^-_{(aq)} \nonumber \]
Neither reaction proceeds very far to the right as written because the formation of the weaker acid–base pair is favored. Both \(HCN\) and acetic acid are stronger acids than water, and hydroxide is a stronger base than either acetate or cyanide, so in both cases, the equilibrium lies to the left. Nonetheless, each of these reactions generates enough hydroxide ions to produce a basic solution. For example, the \(pH\) of a 0.1 M solution of sodium acetate or potassium cyanide at 25°C is 8.8 or 11.1, respectively. From Table \(\PageIndex{1}\) and Figure \(\PageIndex{1}\), we can see that \(CN^−\) is a stronger base (\(pK_b = 4.79\)) than acetate (\(pK_b = 9.24\)), which is consistent with \(KCN\) producing a more basic solution than sodium acetate at the same concentration.
In contrast, the conjugate acid of a weak base should be a weak acid (Equation \(\ref{16.2}\)). For example, ammonium chloride and pyridinium chloride are salts produced by reacting ammonia and pyridine, respectively, with \(HCl\). As you already know, the chloride ion is such a weak base that it does not react with water. In contrast, the cations of the two salts are weak acids that react with water as follows:
\[ NH^+_{4(aq)} + H_2O_{(l)} \ce{ <<=>} HH_{3(aq)} + H_3O^+_{(aq)} \label{16.2} \]
\[ C_5H_5NH^+_{(aq)} + H_2O_{(l)} \ce{<<=>} C_5H_5NH_{(aq)} + H_3O^+_{(aq)} \label{16.3} \]
Equation \(\ref{16.2}\) indicates that \(H_3O^+\) is a stronger acid than either \(NH_4^+\) or \(C_5H_5NH^+\), and conversely, ammonia and pyridine are both stronger bases than water. The equilibrium will therefore lie far to the left in both cases, favoring the weaker acid–base pair. The \(H_3O^+\) concentration produced by the reactions is great enough, however, to decrease the \(pH\) of the solution significantly: the \(pH\) of a 0.10 M solution of ammonium chloride or pyridinium chloride at 25°C is 5.13 or 3.12, respectively. This is consistent with the information shown in Figure 16.2, indicating that the pyridinium ion is more acidic than the ammonium ion.
What happens with aqueous solutions of a salt such as ammonium acetate, where both the cation and the anion can react separately with water to produce an acid and a base, respectively? According to Figure 16.10, the ammonium ion will lower the \(pH\), while according to Equation \(\ref{16.3}\), the acetate ion will raise the \(pH\). This particular case is unusual, in that the cation is as strong an acid as the anion is a base (pKa ≈ pKb). Consequently, the two effects cancel, and the solution remains neutral. With salts in which the cation is a stronger acid than the anion is a base, the final solution has a \(pH\) < 7.00. Conversely, if the cation is a weaker acid than the anion is a base, the final solution has a \(pH\) > 7.00.
Solutions of simple salts of metal ions can also be acidic, even though a metal ion cannot donate a proton directly to water to produce \(H_3O^+\). Instead, a metal ion can act as a Lewis acid and interact with water, a Lewis base, by coordinating to a lone pair of electrons on the oxygen atom to form a hydrated metal ion (part (a) in Figure \(\PageIndex{1}\)). A water molecule coordinated to a metal ion is more acidic than a free water molecule for two reasons. First, repulsive electrostatic interactions between the positively charged metal ion and the partially positively charged hydrogen atoms of the coordinated water molecule make it easier for the coordinated water to lose a proton.
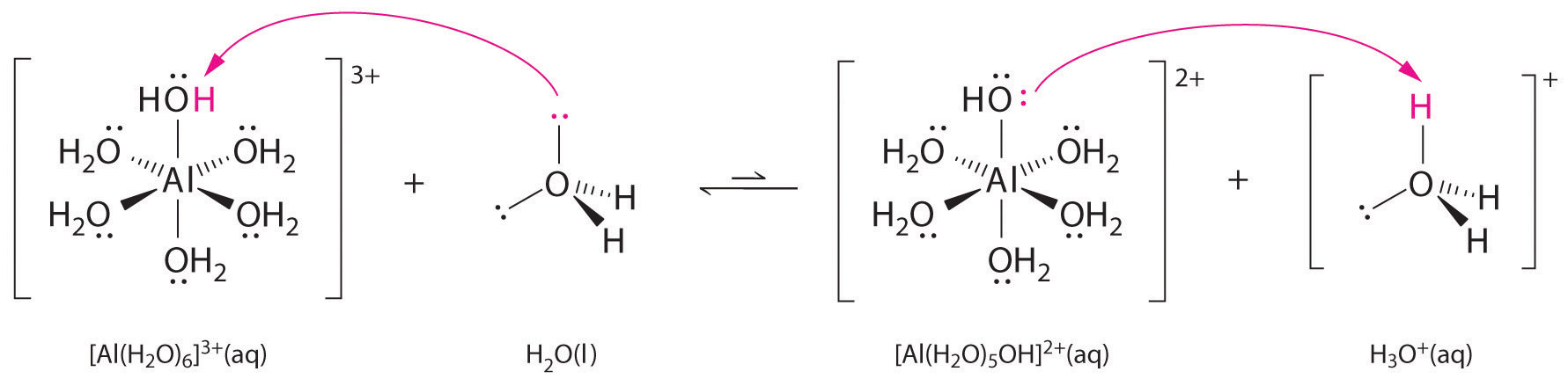
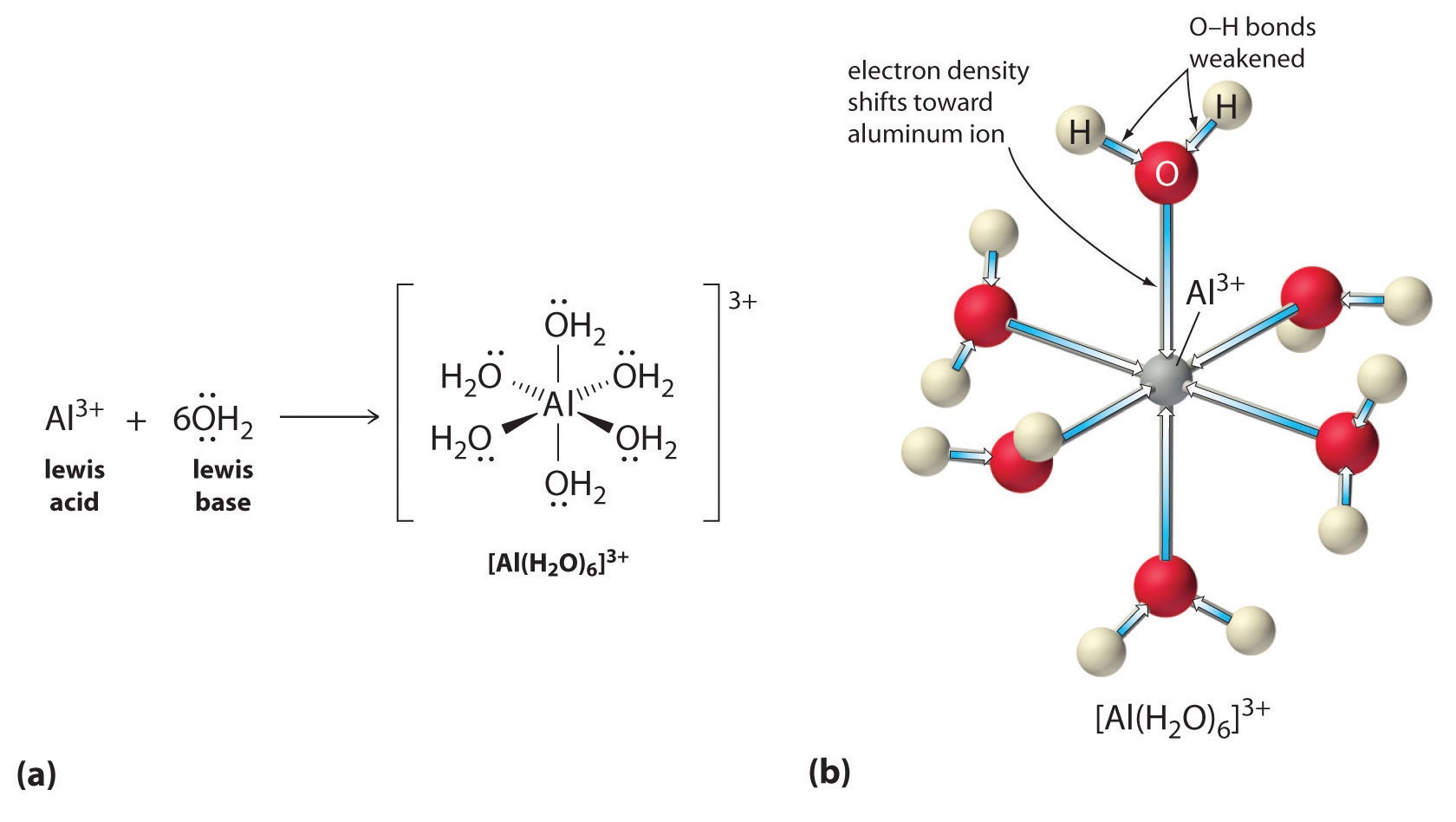
The magnitude of this effect depends on the following two factors (Figure \(\PageIndex{2}\)):
- The charge on the metal ion. A divalent ion (\(M^{2+}\)) has approximately twice as strong an effect on the electron density in a coordinated water molecule as a monovalent ion (\(M^+\)) of the same radius.
- The radius of the metal ion. For metal ions with the same charge, the smaller the ion, the shorter the internuclear distance to the oxygen atom of the water molecule and the greater the effect of the metal on the electron density distribution in the water molecule.
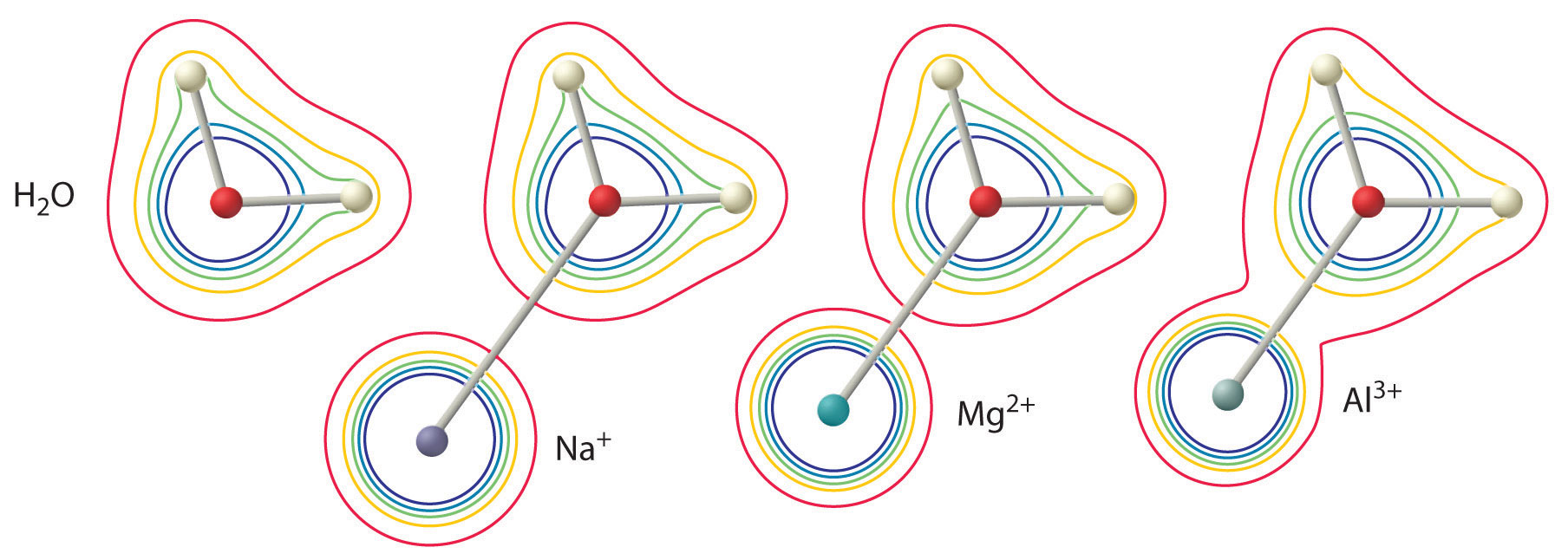
Thus aqueous solutions of small, highly charged metal ions, such as \(Al^{3+}\) and \(Fe^{3+}\), are acidic:
\[[Al(H_2O)_6]^{3+}_{(aq)} \rightleftharpoons [Al(H_2O)_5(OH)]^{2+}_{(aq)}+H^+_{(aq)} \label{16.36} \]
The \([Al(H_2O)_6]^{3+}\) ion has a \(pK_a\) of 5.0, making it almost as strong an acid as acetic acid. Because of the two factors described previously, the most important parameter for predicting the effect of a metal ion on the acidity of coordinated water molecules is the charge-to-radius ratio of the metal ion. A number of pairs of metal ions that lie on a diagonal line in the periodic table, such as \(Li^+\) and \(Mg^{2+}\) or \(Ca^{2+}\) and \(Y^{3+}\), have different sizes and charges, but similar charge-to-radius ratios. As a result, these pairs of metal ions have similar effects on the acidity of coordinated water molecules, and they often exhibit other significant similarities in chemistry as well.
Solutions of small, highly charged metal ions in water are acidic.
Reactions such as those discussed in this section, in which a salt reacts with water to give an acidic or basic solution, are often called hydrolysis reactions. Using a separate name for this type of reaction is unfortunate because it suggests that they are somehow different. In fact, hydrolysis reactions are just acid–base reactions in which the acid is a cation or the base is an anion; they obey the same principles and rules as all other acid–base reactions.
A hydrolysis reaction is an acid–base reaction.
Predict whether aqueous solutions of these compounds are acidic, basic, or neutral.
- \(\ce{KNO_3}\)
- \(\ce{CrBr_3} cdot \ce{H_2O}\)
- \(\ce{Na_2SO_4}\)
Given: compound
Asked for: acidity or basicity of aqueous solution
Strategy:
- Assess the acid–base properties of the cation and the anion. If the cation is a weak Lewis acid, it will not affect the \(pH\) of the solution. If the cation is the conjugate acid of a weak base or a relatively highly charged metal cation, however, it will react with water to produce an acidic solution.
- f the anion is the conjugate base of a strong acid, it will not affect the \(pH\) of the solution. If, however, the anion is the conjugate base of a weak acid, the solution will be basic.
Solution:
a
- The \(K^+\) cation has a small positive charge (+1) and a relatively large radius (because it is in the fourth row of the periodic table), so it is a very weak Lewis acid.
- The \(NO_3−\) anion is the conjugate base of a strong acid, so it has essentially no basic character (Table 16.1). Hence neither the cation nor the anion will react with water to produce \(H^+\) or \(OH^−\), and the solution will be neutral.
b.
- The \(Cr^{3+}\) ion is a relatively highly charged metal cation that should behave similarly to the \(Al^{3+}\) ion and form the \([Cr(H2O)_6]^{3+}\) complex, which will behave as a weak acid: \[ Cr(H_2O)_6]^{3+}_{(aq)} \ce{ <=>>} Cr(H_2O)_5(OH)]^{2+}_{(aq)} + H^+_{(aq)}\nonumber \]
- The \(Br^−\) anion is a very weak base (it is the conjugate base of the strong acid \(HBr\)), so it does not affect the \(pH\) of the solution. Hence the solution will be acidic.
c.
- The \(Na^+\) ion, like the \(K^+\), is a very weak acid, so it should not affect the acidity of the solution.
- In contrast, \(SO_4^{2−}\) is the conjugate base of \(HSO_4^−\), which is a weak acid. Hence the \(SO_4^{2−}\) ion will react with water as shown in Figure 16.6 to give a slightly basic solution.
Predict whether aqueous solutions of the following are acidic, basic, or neutral.
- \(KI\)
- \(Mg(ClO_4)_2\)
- \(NaHS\)
- Answer a
-
neutral
- Answer b
-
acidic
- Answer c
-
basic (due to the reaction of \(\ce{HS^{-}}\) with water to form \(\ce{H_2S}\) and \(\ce{OH^{-}}\))
Summary
A salt can dissolve in water to produce a neutral, a basic, or an acidic solution, depending on whether it contains the conjugate base of a weak acid as the anion (\(A^−\)), the conjugate acid of a weak base as the cation (\(BH^+\)), or both. Salts that contain small, highly charged metal ions produce acidic solutions in water. The reaction of a salt with water to produce an acidic or a basic solution is called a hydrolysis reaction.
Key Takeaways
- Acid–base reactions always contain two conjugate acid–base pairs.
- Each acid and each base has an associated ionization constant that corresponds to its acid or base strength.