13.12: Biocatalysis with Enzymes
- Page ID
- 285717
Nature has provided some ideal catalysts in the form of enzymes, the use of which offers some substantial advantages in green chemistry. The most obvious advantage is that enzymes have evolved to work under the mild conditions in which organisms function, especially moderate temperatures and physiological pH. Unlike precious metal catalysts that are commonly used, enzymes are made renewably from biomaterials. Enzymes have generally high activities and are highly selective in the chemical processes that they carry out. Whereas conventional organic syntheses often require activation or protection of functional groups, both of which may consume reagents and hence produce more wastes, these measures are often not needed with enzymes. The result is that biocatalyzed reactions can frequently be carried out with relatively fewer steps and less wastes making them more attractive environmentally and economically.
Although some biocatalyzed reactions have been used for production of chemicals for centuries — the production of ethanol by yeast fermentation of sugar comes immediately to mind— relatively recent advances in biotechnology have greatly increased their versatility and utility. One such advance has been with recombinant DNA in which enzymes that perform specific functions may be transferred between organisms. The other major advance has been with directed evolution in which the amino acid sequences in enzymes produced by genes are shuffled randomly and large numbers of the products are sampled for their activity, particularly for carrying out a particular biochemical synthetic step. This may be done within living cells, but can be carried out on a much larger scale outside of cells, a process called in vitro evolution. Obviously, most of the enzymes produced by this technique are not superior, or are even useless, but out of the enormous numbers generated, some will be superior. In vitro evolution is being carried out to provide enzymes with properties such as improved or different catalytic activity, catalytic specificity, thermostability, and pH optima that can be used in industrial, medical, or agricultural applications. It is proving particularly useful in developing enzymes that act upon compounds that do not occur in nature and for which enzymes have not evolved through natural evolution.
Immobilized Enzyme Catalysts
Disadvantages of catalytic enzymes include often low stability, limited storage times, difficult recovery, and product contamination, almost always a consideration with homogeneous catalysts. The stability and recyclability of enzymes may often be enhanced by immobilizing them on solid supports. A common means of immobilizing enzymes is by their precipitation from the fermentation broth in which they are generated by a buffer such as ammonium sulfate and stabilizing the precipitated aggregates with a reagent possessing two bonding functional groups, commonly glutaraldehyde a reagent that is widely used for cross-linking proteins:
The precipitation and cross-linking of the enzyme combines its isolation and immobilization into a single step. Enzymes prepared in this way are usually highly productive, stable and resistant to denaturation (loss of enzyme function by structural alteration) due to exposure to organic solvents, heating, or breakdown to shorter peptide chains or amino acids by the action of proteolytic enzymes (proteolysis).
Reduction in Synthesis Steps with Enzyme Catalysts
Typical synthesis of an organic compound, especially one as complicated as many pharmaceutical agents, may involve multiple steps. Because of factors such as product loss, the need to protect and deprotect functional groups, and generation of wastes from each step, these multistep syntheses tend to build high E factors overall. The ideal synthesis is a “one-pot” process in which all steps are carried out in the same operation without the need to isolate intermediates. Living cells are often “one-pot” synthetic factories, so it is natural to look to enzymes to accomplish the same thing in the laboratory and in chemical production using multiple enzymes ina single container and for a single multiple-step operation. Accomplishment of such a process can be complicated by incompatibility of different enzymes and the different conditions under which enzymes operate although in general they operate in water under ambient conditions compatible with life. (The relatively recent discovery of organisms that live under hot, extreme conditions on the deep ocean floor raises some interesting possibilities for the isolation of enzymes that might function under unusual conditions, particularly elevated temperatures.)
Enzyme Catalysts and Chirality
As shown by the example of the herbicide mecoprop in Figure 13.6, chiral molecules are three-dimensional molecules with structures such that a molecule cannot be directly imposed on its mirror image. Chiral molecules have different groups arranged around an atom, usually of carbon, that constitutes a chiral center. Two chiral molecules of the same compound are called enantiomers commonly designated as R and S. The physical and chemical properties of enantiomers are generally identical, having the same melting points, boiling points and solubilities. However, one enantiomer of a compound may fit exactly with an enzyme active site whereas the other does not. This results in often markedly different biochemical properties of enantiomers and consequently completely different environmental and toxicological behaviors. One enantiomer of a chiral pharmaceutical may function very well whereas the other does not work at all or may even be toxic. Biochemical differences between enantiomers may be especially pronounced for pesticides. For example, the R enantiomer of herbicidal mecoprop (Figure 13.6) kills weeds very effectively whereas the S enantiomer is inactive; therefore, the pure R enantiomer is now marketed as a herbicide in place of the racemic mixture with the S enantiomer.
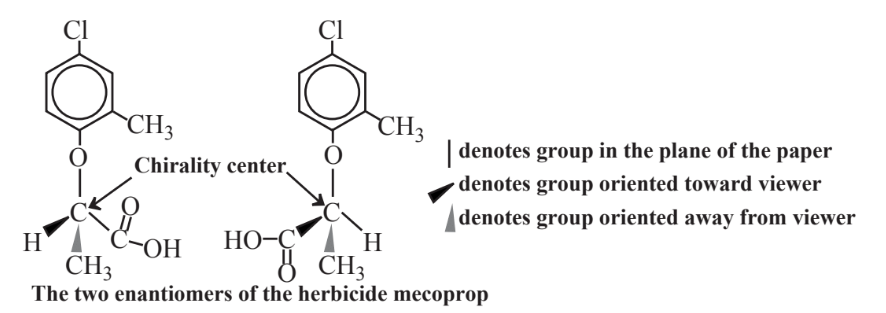
Normally when a chiral compound is synthesized by conventional chemical means a racemic mixture of the two enantiomers is produced. Because of their essentially identical chemical properties they are very hard to separate. However, it is possible to produce enantiomerically pure chemical compounds with appropriate enzymatic catalysts. Furthermore, it is possible to use enzymatic catalysts to convert racemic mixtures of compounds to enantiomerically pure forms. In one of the larger such industrial operations, BASF now uses enzymatically catalyzed processes to prepare enantiomerically pure amines in thousand-ton quantities.
Nature’s catalysts, the enzymes in organisms, are experts in carrying out chemical processes efficiently under mild conditions. In consideration of this fact, a great deal of attention is being devoted to using organisms, especially bacteria, to carry out chemical processes. By splicing desired genes for making specific enzymes into bacteria so that they will carry out desired reactions, genetic engineering is making an increasing contribution to the development of enzyme-catalyzed green chemical processes.
Chemists are trying to use enzymes as models for synthetic catalysts that have performance characteristics of enzymes, but which are much simpler and work under conditions that would destroy enzymatic catalysts. A promising area in which this might be accomplished is the use of iron-containing catalysts to oxidize alkene (C=C) groups in organic compounds using relatively mild hydrogen peroxide reagent, H2O2. Organisms accomplish this task using catalysts in which the Fe2+ ion is bonded by four N atoms in relatively large heme porphyrin molecules. The same oxidation has now been accomplished with a catalyst in which Fe2+ is bound by four N atoms by an organic molecule with the formidable name of N,N'-dimethyl-N,N'bis(2-pyridylmethyl)-ethylenediamine as shown in Figure 13.7. A big advantage of this catalyst that is shared with enzyme catalysts that enable peroxide oxidations is that it does not cause the decomposition of hydrogen peroxide as do a number of synthetic catalysts.