6.2A: Flames
- Page ID
- 111689
\( \newcommand{\vecs}[1]{\overset { \scriptstyle \rightharpoonup} {\mathbf{#1}} } \)
\( \newcommand{\vecd}[1]{\overset{-\!-\!\rightharpoonup}{\vphantom{a}\smash {#1}}} \)
\( \newcommand{\id}{\mathrm{id}}\) \( \newcommand{\Span}{\mathrm{span}}\)
( \newcommand{\kernel}{\mathrm{null}\,}\) \( \newcommand{\range}{\mathrm{range}\,}\)
\( \newcommand{\RealPart}{\mathrm{Re}}\) \( \newcommand{\ImaginaryPart}{\mathrm{Im}}\)
\( \newcommand{\Argument}{\mathrm{Arg}}\) \( \newcommand{\norm}[1]{\| #1 \|}\)
\( \newcommand{\inner}[2]{\langle #1, #2 \rangle}\)
\( \newcommand{\Span}{\mathrm{span}}\)
\( \newcommand{\id}{\mathrm{id}}\)
\( \newcommand{\Span}{\mathrm{span}}\)
\( \newcommand{\kernel}{\mathrm{null}\,}\)
\( \newcommand{\range}{\mathrm{range}\,}\)
\( \newcommand{\RealPart}{\mathrm{Re}}\)
\( \newcommand{\ImaginaryPart}{\mathrm{Im}}\)
\( \newcommand{\Argument}{\mathrm{Arg}}\)
\( \newcommand{\norm}[1]{\| #1 \|}\)
\( \newcommand{\inner}[2]{\langle #1, #2 \rangle}\)
\( \newcommand{\Span}{\mathrm{span}}\) \( \newcommand{\AA}{\unicode[.8,0]{x212B}}\)
\( \newcommand{\vectorA}[1]{\vec{#1}} % arrow\)
\( \newcommand{\vectorAt}[1]{\vec{\text{#1}}} % arrow\)
\( \newcommand{\vectorB}[1]{\overset { \scriptstyle \rightharpoonup} {\mathbf{#1}} } \)
\( \newcommand{\vectorC}[1]{\textbf{#1}} \)
\( \newcommand{\vectorD}[1]{\overrightarrow{#1}} \)
\( \newcommand{\vectorDt}[1]{\overrightarrow{\text{#1}}} \)
\( \newcommand{\vectE}[1]{\overset{-\!-\!\rightharpoonup}{\vphantom{a}\smash{\mathbf {#1}}}} \)
\( \newcommand{\vecs}[1]{\overset { \scriptstyle \rightharpoonup} {\mathbf{#1}} } \)
\( \newcommand{\vecd}[1]{\overset{-\!-\!\rightharpoonup}{\vphantom{a}\smash {#1}}} \)
\(\newcommand{\avec}{\mathbf a}\) \(\newcommand{\bvec}{\mathbf b}\) \(\newcommand{\cvec}{\mathbf c}\) \(\newcommand{\dvec}{\mathbf d}\) \(\newcommand{\dtil}{\widetilde{\mathbf d}}\) \(\newcommand{\evec}{\mathbf e}\) \(\newcommand{\fvec}{\mathbf f}\) \(\newcommand{\nvec}{\mathbf n}\) \(\newcommand{\pvec}{\mathbf p}\) \(\newcommand{\qvec}{\mathbf q}\) \(\newcommand{\svec}{\mathbf s}\) \(\newcommand{\tvec}{\mathbf t}\) \(\newcommand{\uvec}{\mathbf u}\) \(\newcommand{\vvec}{\mathbf v}\) \(\newcommand{\wvec}{\mathbf w}\) \(\newcommand{\xvec}{\mathbf x}\) \(\newcommand{\yvec}{\mathbf y}\) \(\newcommand{\zvec}{\mathbf z}\) \(\newcommand{\rvec}{\mathbf r}\) \(\newcommand{\mvec}{\mathbf m}\) \(\newcommand{\zerovec}{\mathbf 0}\) \(\newcommand{\onevec}{\mathbf 1}\) \(\newcommand{\real}{\mathbb R}\) \(\newcommand{\twovec}[2]{\left[\begin{array}{r}#1 \\ #2 \end{array}\right]}\) \(\newcommand{\ctwovec}[2]{\left[\begin{array}{c}#1 \\ #2 \end{array}\right]}\) \(\newcommand{\threevec}[3]{\left[\begin{array}{r}#1 \\ #2 \\ #3 \end{array}\right]}\) \(\newcommand{\cthreevec}[3]{\left[\begin{array}{c}#1 \\ #2 \\ #3 \end{array}\right]}\) \(\newcommand{\fourvec}[4]{\left[\begin{array}{r}#1 \\ #2 \\ #3 \\ #4 \end{array}\right]}\) \(\newcommand{\cfourvec}[4]{\left[\begin{array}{c}#1 \\ #2 \\ #3 \\ #4 \end{array}\right]}\) \(\newcommand{\fivevec}[5]{\left[\begin{array}{r}#1 \\ #2 \\ #3 \\ #4 \\ #5 \\ \end{array}\right]}\) \(\newcommand{\cfivevec}[5]{\left[\begin{array}{c}#1 \\ #2 \\ #3 \\ #4 \\ #5 \\ \end{array}\right]}\) \(\newcommand{\mattwo}[4]{\left[\begin{array}{rr}#1 \amp #2 \\ #3 \amp #4 \\ \end{array}\right]}\) \(\newcommand{\laspan}[1]{\text{Span}\{#1\}}\) \(\newcommand{\bcal}{\cal B}\) \(\newcommand{\ccal}{\cal C}\) \(\newcommand{\scal}{\cal S}\) \(\newcommand{\wcal}{\cal W}\) \(\newcommand{\ecal}{\cal E}\) \(\newcommand{\coords}[2]{\left\{#1\right\}_{#2}}\) \(\newcommand{\gray}[1]{\color{gray}{#1}}\) \(\newcommand{\lgray}[1]{\color{lightgray}{#1}}\) \(\newcommand{\rank}{\operatorname{rank}}\) \(\newcommand{\row}{\text{Row}}\) \(\newcommand{\col}{\text{Col}}\) \(\renewcommand{\row}{\text{Row}}\) \(\newcommand{\nul}{\text{Nul}}\) \(\newcommand{\var}{\text{Var}}\) \(\newcommand{\corr}{\text{corr}}\) \(\newcommand{\len}[1]{\left|#1\right|}\) \(\newcommand{\bbar}{\overline{\bvec}}\) \(\newcommand{\bhat}{\widehat{\bvec}}\) \(\newcommand{\bperp}{\bvec^\perp}\) \(\newcommand{\xhat}{\widehat{\xvec}}\) \(\newcommand{\vhat}{\widehat{\vvec}}\) \(\newcommand{\uhat}{\widehat{\uvec}}\) \(\newcommand{\what}{\widehat{\wvec}}\) \(\newcommand{\Sighat}{\widehat{\Sigma}}\) \(\newcommand{\lt}{<}\) \(\newcommand{\gt}{>}\) \(\newcommand{\amp}{&}\) \(\definecolor{fillinmathshade}{gray}{0.9}\)As alluded to earlier, flames can be used as an atomization source for liquid samples. The sample is introduced into the flame as an aerosol mist. The process of creating the aerosol is referred to as nebulization. Common nebulizer designs include pneumatic and ultrasonic devices, the details of which we will not go into here. The most common flame atomization device, which is illustrated in Figure \(\PageIndex{1}\), is known as a laminar flow or pre-mix burner. Note the unusual design of the burner head, which instead of having the shape of a common Bunsen burner, has a long, thin flame that is 10 cm long. Radiation from the course passes through the 10 cm distance of the flame. Often the monochromator is placed after the flame and before the detector. If atomic emission is being measured, there is no light source. The burner design provides a much longer path length to increase the sensitivity of the method.
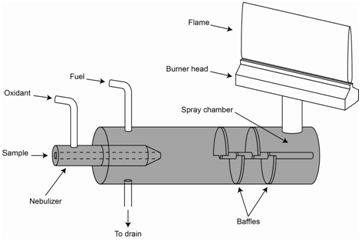
A flame requires a fuel and oxidant. In the laminar flow burner, the fuel and oxidant are pre-mixed at the bottom of a chamber. The force created by the flowing gases draws sample up through a thin piece of tubing where it is nebulized into the bottom of the chamber. The chamber has a series of baffles in it that creates an obstructed pathway up to the burner head. The purpose of the baffles is to allow only the finest aerosol particles to reach the flame. Larger particles strike the baffles, collect and empty out by the drain tube. Even using the best nebulizers that have been developed, only about 2% of the sample actually makes it through the baffles and to the flame. The remaining 98% empties out the drain.
At first it might seem counterintuitive to discard 98% of the sample and instead seem preferable to introduce the entire sample into the flame, but we must consider what happens to an aerosol droplet after it is created and as it enters the flame. Remembering that the solution has molecules but we need atoms, there are several steps required to complete this transformation. The first involves evaporating the solvent (Equation \ref{eq1}). Many metal complexes form hydrates and the next step involves dehydration (Equation \ref{eq2}). The metal complexes must be volatilized (Equation \ref{eq3}) and then decomposed (Equation \ref{eq4}). Finally, the metal ions must be reduced to neutral atoms (Equation \ref{eq5}). Only now are we able to measure the absorbance by the metal atoms. If the measurement involves atomic emission, then a sixth step (Equation \ref{eq6}) involves the excitation of the atoms.
\[\begin{align}
\ce{ML(aq)} &= \ce{ML^.xH_2O (s)} \label{eq1}\\[4pt]
\ce{ML^.xH_2O (s)} &= \ce{ML(s)} \label{eq2}\\[4pt]
\ce{ML(s)} &= \ce{ML(g)} \label{eq3}\\[4pt]
\ce{ML(g)} &= \ce{ M+ + L- } \label{eq4}\\[4pt]
\ce{M+ + e-} &= \ce{M} \label{eq5}\\[4pt]
\ce{M + heat} &= \ce{M^{*}} \label{eq6}\
\end{align} \nonumber \]
The problem with large aerosol droplets is that they will not make it through all of the necessary steps during their lifetime in the flame. These drops will contribute little to the signal, but their presence in the flame will create noise and instability in the flame that will compromise the measurement. Hence, only the finest aerosol droplets will lead to atomic species and only those are introduced into the flame.
The various steps outlined in Equations \ref{eq1}-\ref{eq6} also imply that there will be a distinct profile to the flame. Profiles result because of the efficiency with which neutral and excited atoms are formed in a flame. Therefore, a specific section of the flame will have the highest concentration of ground state atoms for the metal being analyzed. The absorbance profile that shows the concentration of ground state atoms in the flame is likely to be different than the emission profile that shows the concentration of excited state atoms in the flame.
Figure \(\PageIndex{2}\) shows representative absorption profiles for chromium, magnesium and silver. Magnesium shows a peak in its profile. The increase in the lower part of the flame occurs because exposure to the heat creates more neutral ground state atoms. The decrease in the upper part of the flame occurs due to the formation of magnesium oxide species that do not absorb the atomic line. Silver is not as easily oxidized and its concentration continually increases the longer the sample is exposed to the heat of the flame. Chromium forms very stable oxides and the concentration of ground state atoms decreases the longer it is exposed to the heat of the flame.
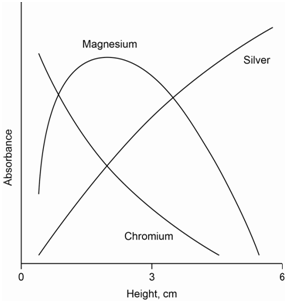
When performing atomic absorbance or emission measurements using a flame atomization source, it is important to measure the section of the flame with the highest concentration. There are controls in the instrument to raise and lower the burner head to insure that the light beam passes through the optimal part of the flame.
An important factor in the characteristics of a flame is the identity of the fuel and oxidant. Standard Bunsen burner flames use methane as the fuel and air as the oxidant and have a temperature in the range of 1,700-1,900oC. A flame with acetylene as the fuel and air as the oxidant has a temperature in the range of 2,100-2,400oC. For most elements, the methane/air flame is too cool to provide suitable atomization efficiencies for atomic absorbance or emission measurements, and an acetylene/air flame must be used. For some elements, the use of a flame with acetylene as the fuel and nitrous oxide (N2O) as the oxidant is recommended. The acetylene/nitrous oxide flame has a temperature range of about 2,600-2,800oC. There are standard reference books on atomic methods that specify the type of flame that is best suited for the analysis of particular elements.
It is also important to recognize that some elements do not atomize well in flames. Flame and other atomization methods are most suitable for the measurement of metals. Non-metallic elements rarely atomize with enough efficiency to permit analysis of trace levels. Metalloids such as arsenic and selenium have intermediate atomization efficiencies and may require specialized atomization methods for certain samples with trace levels of the elements. Mercury is another atom that does not atomize well and often requires the use of a specialized atomization procedure. Flame methods are usually used for atomic absorbance measurements because most elements do not produce high enough concentrations of excited atoms to facilitate sensitive detection based on atomic emission. Alkali metals can be measured in a flame by atomic emission. Alkaline earth metals can possibly be measured by flame emission as well provided the concentration is high enough.