11: Reprocessing Spent Fuel
- Page ID
- 19576
\( \newcommand{\vecs}[1]{\overset { \scriptstyle \rightharpoonup} {\mathbf{#1}} } \)
\( \newcommand{\vecd}[1]{\overset{-\!-\!\rightharpoonup}{\vphantom{a}\smash {#1}}} \)
\( \newcommand{\id}{\mathrm{id}}\) \( \newcommand{\Span}{\mathrm{span}}\)
( \newcommand{\kernel}{\mathrm{null}\,}\) \( \newcommand{\range}{\mathrm{range}\,}\)
\( \newcommand{\RealPart}{\mathrm{Re}}\) \( \newcommand{\ImaginaryPart}{\mathrm{Im}}\)
\( \newcommand{\Argument}{\mathrm{Arg}}\) \( \newcommand{\norm}[1]{\| #1 \|}\)
\( \newcommand{\inner}[2]{\langle #1, #2 \rangle}\)
\( \newcommand{\Span}{\mathrm{span}}\)
\( \newcommand{\id}{\mathrm{id}}\)
\( \newcommand{\Span}{\mathrm{span}}\)
\( \newcommand{\kernel}{\mathrm{null}\,}\)
\( \newcommand{\range}{\mathrm{range}\,}\)
\( \newcommand{\RealPart}{\mathrm{Re}}\)
\( \newcommand{\ImaginaryPart}{\mathrm{Im}}\)
\( \newcommand{\Argument}{\mathrm{Arg}}\)
\( \newcommand{\norm}[1]{\| #1 \|}\)
\( \newcommand{\inner}[2]{\langle #1, #2 \rangle}\)
\( \newcommand{\Span}{\mathrm{span}}\) \( \newcommand{\AA}{\unicode[.8,0]{x212B}}\)
\( \newcommand{\vectorA}[1]{\vec{#1}} % arrow\)
\( \newcommand{\vectorAt}[1]{\vec{\text{#1}}} % arrow\)
\( \newcommand{\vectorB}[1]{\overset { \scriptstyle \rightharpoonup} {\mathbf{#1}} } \)
\( \newcommand{\vectorC}[1]{\textbf{#1}} \)
\( \newcommand{\vectorD}[1]{\overrightarrow{#1}} \)
\( \newcommand{\vectorDt}[1]{\overrightarrow{\text{#1}}} \)
\( \newcommand{\vectE}[1]{\overset{-\!-\!\rightharpoonup}{\vphantom{a}\smash{\mathbf {#1}}}} \)
\( \newcommand{\vecs}[1]{\overset { \scriptstyle \rightharpoonup} {\mathbf{#1}} } \)
\( \newcommand{\vecd}[1]{\overset{-\!-\!\rightharpoonup}{\vphantom{a}\smash {#1}}} \)
\(\newcommand{\avec}{\mathbf a}\) \(\newcommand{\bvec}{\mathbf b}\) \(\newcommand{\cvec}{\mathbf c}\) \(\newcommand{\dvec}{\mathbf d}\) \(\newcommand{\dtil}{\widetilde{\mathbf d}}\) \(\newcommand{\evec}{\mathbf e}\) \(\newcommand{\fvec}{\mathbf f}\) \(\newcommand{\nvec}{\mathbf n}\) \(\newcommand{\pvec}{\mathbf p}\) \(\newcommand{\qvec}{\mathbf q}\) \(\newcommand{\svec}{\mathbf s}\) \(\newcommand{\tvec}{\mathbf t}\) \(\newcommand{\uvec}{\mathbf u}\) \(\newcommand{\vvec}{\mathbf v}\) \(\newcommand{\wvec}{\mathbf w}\) \(\newcommand{\xvec}{\mathbf x}\) \(\newcommand{\yvec}{\mathbf y}\) \(\newcommand{\zvec}{\mathbf z}\) \(\newcommand{\rvec}{\mathbf r}\) \(\newcommand{\mvec}{\mathbf m}\) \(\newcommand{\zerovec}{\mathbf 0}\) \(\newcommand{\onevec}{\mathbf 1}\) \(\newcommand{\real}{\mathbb R}\) \(\newcommand{\twovec}[2]{\left[\begin{array}{r}#1 \\ #2 \end{array}\right]}\) \(\newcommand{\ctwovec}[2]{\left[\begin{array}{c}#1 \\ #2 \end{array}\right]}\) \(\newcommand{\threevec}[3]{\left[\begin{array}{r}#1 \\ #2 \\ #3 \end{array}\right]}\) \(\newcommand{\cthreevec}[3]{\left[\begin{array}{c}#1 \\ #2 \\ #3 \end{array}\right]}\) \(\newcommand{\fourvec}[4]{\left[\begin{array}{r}#1 \\ #2 \\ #3 \\ #4 \end{array}\right]}\) \(\newcommand{\cfourvec}[4]{\left[\begin{array}{c}#1 \\ #2 \\ #3 \\ #4 \end{array}\right]}\) \(\newcommand{\fivevec}[5]{\left[\begin{array}{r}#1 \\ #2 \\ #3 \\ #4 \\ #5 \\ \end{array}\right]}\) \(\newcommand{\cfivevec}[5]{\left[\begin{array}{c}#1 \\ #2 \\ #3 \\ #4 \\ #5 \\ \end{array}\right]}\) \(\newcommand{\mattwo}[4]{\left[\begin{array}{rr}#1 \amp #2 \\ #3 \amp #4 \\ \end{array}\right]}\) \(\newcommand{\laspan}[1]{\text{Span}\{#1\}}\) \(\newcommand{\bcal}{\cal B}\) \(\newcommand{\ccal}{\cal C}\) \(\newcommand{\scal}{\cal S}\) \(\newcommand{\wcal}{\cal W}\) \(\newcommand{\ecal}{\cal E}\) \(\newcommand{\coords}[2]{\left\{#1\right\}_{#2}}\) \(\newcommand{\gray}[1]{\color{gray}{#1}}\) \(\newcommand{\lgray}[1]{\color{lightgray}{#1}}\) \(\newcommand{\rank}{\operatorname{rank}}\) \(\newcommand{\row}{\text{Row}}\) \(\newcommand{\col}{\text{Col}}\) \(\renewcommand{\row}{\text{Row}}\) \(\newcommand{\nul}{\text{Nul}}\) \(\newcommand{\var}{\text{Var}}\) \(\newcommand{\corr}{\text{corr}}\) \(\newcommand{\len}[1]{\left|#1\right|}\) \(\newcommand{\bbar}{\overline{\bvec}}\) \(\newcommand{\bhat}{\widehat{\bvec}}\) \(\newcommand{\bperp}{\bvec^\perp}\) \(\newcommand{\xhat}{\widehat{\xvec}}\) \(\newcommand{\vhat}{\widehat{\vvec}}\) \(\newcommand{\uhat}{\widehat{\uvec}}\) \(\newcommand{\what}{\widehat{\wvec}}\) \(\newcommand{\Sighat}{\widehat{\Sigma}}\) \(\newcommand{\lt}{<}\) \(\newcommand{\gt}{>}\) \(\newcommand{\amp}{&}\) \(\definecolor{fillinmathshade}{gray}{0.9}\)The plutonium for the atomic device tested in New Mexico and the atomic bomb dropped on Nagasaki, Japan was produced by bombarding fertile U-238 with neutrons in nuclear reactors and recovering the resulting fissile Pu-239 by chemical methods. Until 1942, bombardment of targets composed of uranium compounds with deuterons produced by a cyclotron was the only source of the newly discovered element, plutonium.
In December 1942, Enrico Fermi’s controlled chain reaction at the University of Chicago produced tiny quantities of plutonium in addition to energy and fission products. In November 1943, a larger pilot reactor at Oak Ridge, Tennessee, known as X-10, went critical and began to produce plutonium (Figure 1). Fuel rods consisting of natural uranium were inserted into the reactor for a given period of time. At the end of this period, the rods were removed and allowed to cool. Plutonium at a concentration of approximately 250 parts per million was then separated from fission products and uranium-238. In 1945, the first of three large-scale reactors began to produce plutonium at Hanford, Washington.
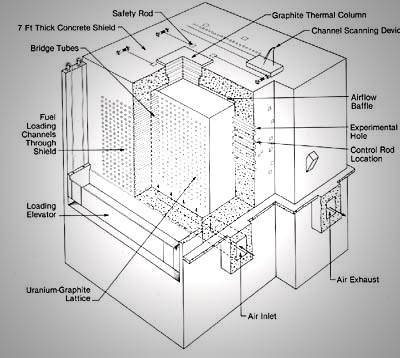
The fertile U-238 in natural uranium captures neutrons to produce Pu-239, while the U-235 sustains the chain reaction required to produce more neutrons. As the concentration of Pu-239 increases in the reactor, it also undergoes fission. The production of other plutonium isotopes occurs at a slower rate than that of Pu-239. Thus, uranium that has been in the reactor for a short period of time has a significant amount of Pu-239 relative to the heavier Pu isotopes. The fuel rods are removed from the reactor after a period of time chosen to maximize the yield of Pu-239 while minimizing the other neutron absorbing plutonium isotopes. Today most nuclear weapons use Pu-239 as the fissile material.
Recovery of Plutonium from Spent Fuel Rods
The next problem was the quantitative recovery of the small amounts of plutonium from the large amounts of uranium and fission products in the fuel rods. The radioactive products of U-235 fission had to be reduced to a concentration of less than one part in 107 parts plutonium. This step was required to reduce the gamma radiation from the fission products to make the plutonium safer to handle and to remove neutron-absorbing impurities. This process, carried on at Hanford in large buildings known as “Queen Marys”, produced the plutonium for the Trinity test and the Nagasaki atomic bomb.
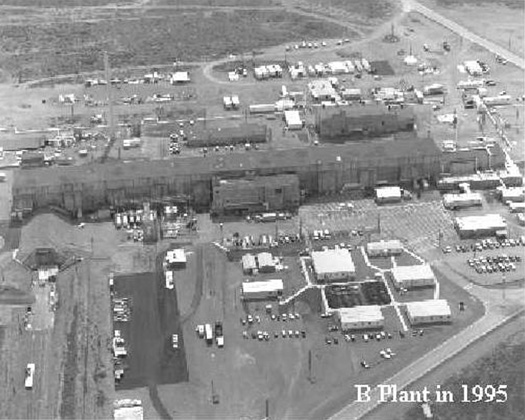
Because of the low concentration of plutonium, plutonium compounds could not be precipitated directly. Thus, any separation process involving precipitation had to be based on coprecipitation techniques that employed carriers for plutonium. The bismuth phosphate process was first used for large-scale separation of plutonium from uranium and the fission products (Figure 2). The keys to this process were the quantitative, selective coprecipitation of \(Pu^{4+}\) from an acid solution by a bismuth phosphate carrier and the ability of \(Pu^{6+}\) to remain in solution in the presence of the bismuth phosphate carrier (Figure 3)
Figure 3: Bismuth Phosphate Process for Producing Plutonium. Courtesy of the U.S. Department of Energy
The neutron-irradiated, aluminum-clad fuel rods were removed from the reactor to a pool of water, where they remained for a number of days to allow the short-lived, high-activity fission products to decay. The aluminum casing was removed from the rods with a concentrated sodium hydroxide solution. The contents of the fuel rods were dissolved in nitric acid and, after the addition of sulfuric acid to keep the uranium and other fission products in solution, Pu4+ was coprecipitated with bismuth phosphate. The precipitate containing the plutonium was then dissolved in nitric acid and the Pu4+ was oxidized to Pu6+ with sodium dichromate. This time, the plutonium remained in solution as Pu6+ while any residual uranium and fission products were precipitated with additional bismuth phosphate. The Pu6+ was then reduced to Pu4+ and the cycle was repeated. At this point, the carrier was changed to lanthanum fluoride (LaF3) and a similar oxidation-reduction cycle was performed to achieve further purification and concentration of the plutonium.
The concentration of plutonium was high enough so that no carrier was required for the final purification step, the precipitation of plutonium peroxide from a basic solution. The peroxide was converted to a plutonium nitrate paste that was shipped to Los Alamos. The overall recovery of plutonium by this process was greater than 95%. The process generated large amounts of chemical and radioactive wastes (approximately 10,000 gallons per metric ton of uranium processed). A major shortcoming was that only plutonium could be extracted; the uranium remained in the waste.
By the 1960s, the plutonium/uranium/recovery/extraction (PUREX) process replaced the bismuth phosphate process (Figure 4). It is capable of recovering plutonium, uranium, and other materials with a continuous extraction using organic solvents. This process also generates less waste than the bismuth phosphate process did. After dissolution in nitric acid, the plutonium and uranium nitrates are transferred into the organic phase, while the fission products are removed in the aqueous phase. The separated plutonium is reduced to the metal, alloyed with gallium, and shaped into grapefruit sized pits, which are the heart of a fission weapon.
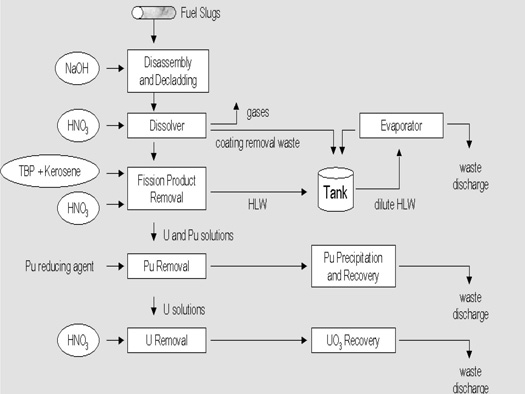
Reprocessing Spent Nuclear Fuel for Nuclear Power
In the closed nuclear fuel cycle, spent reactor fuel is reprocessed to recover the uranium and plutonium to fuel nuclear reactors using the PUREX process. Reprocessing also reduces the amount of radioactive waste that must be managed. Table 1 shows the composition of fresh and spent fuel rods removed from a typical light water reactor after three years.
Material | Fresh Fuel % | Spent Fuel % | Type of Waste |
---|---|---|---|
Transuranic elements | 0.00 | 0.065 | Transuranic |
U-236 | 0.00 | 0.46 | Depleted uranium |
Pu isotopes | 0.00 | 0.89 | Transuranic |
Fission products | 0.00 | 0.35 | High level |
U-235 | 3.3 | 0.08 | Depleted uranium |
U-238 | 96.7 | 94.3 | Depleted uranium |
After 3 years in a reactor, a fuel rod assembly containing 264 rods weighing 1,450 lbs would contain 12.9 pounds of plutonium, 5.07 pounds of fission products, and 1,367 pounds of U-238. Separating the plutonium and uranium from the other products addresses two major issues. The plutonium can be used directly as nuclear fuel and the uranium can be reprocessed to produce additional fuel. However, reprocessing raises concerns about the production of fissile Pu-239 which can be used in nuclear weapons. It also produces large volumes of chemical and radioactive wastes.
The fuel extracted from power reactors where fuel rods remain for extended periods of time contains concentrations of Pu-240, which makes the plutonium unsuitable for use in a weapon. The plutonium produced in reactors which are refueled frequently contains lower concentrations of Pu-240 and is suitable for use in weapons.
The separated plutonium in the form of PuO2 is mixed with depleted uranium containing greater than 99.3% U-238 from an enrichment plant to form the mixed oxide (MOX) fuel containing 7 to 9% Pu and the reminder UO2. The MOX is then mixed with low enriched uranium (LEU) oxide for use as fuel in light water reactors. Existing reactor designs limit the fuel composition to 1/3 MOX and 2/3 LEU. Currently once the MOX fuel is used in a reactor it is not reprocessed again.
Plutonium from dismantled nuclear warheads can also be used as fuel for power reactors. However, the method of reprocessing this plutonium is different from that used for spent reactor fuel because the plutonium used in weapons is alloyed with gallium (Figure 5). The plutonium is separated from the gallium and converted into PuO2 to be mixed with UO2 containing depleted uranium. At this point the MOX is similar to that produced from spent reactor fuel with the exception that the MOX composition is adjusted to 5% Pu since weapons grade plutonium contains over 90% Pu-239. The MOX powder is converted to pellets, which are sealed in fuel rods for placement in fuel assemblies.
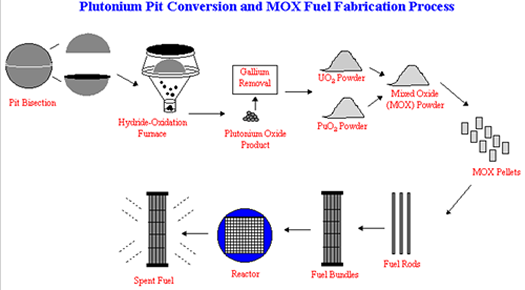
In 2008 France and the United Kingdom each had one reprocessing facility in operation. Japan plans to bring one on line in 2012. In 1977 President Carter established a United States policy that prohibited reprocessing based on the premise that limiting plutonium would limit the spread of nuclear weapons around the world. Although President Reagan later reversed this policy, no spent fuel from power reactors has been reprocessed. This is most likely a result of the fact that producing enriched uranium in the United States has remained less expensive than reprocessing spent fuel.
Complete Bibliography on Plutonium from the Alsos Digital Library for Nuclear Issues
Contributors
Frank A. Settle (Washington and Lee University)