Exceptions to the Octet Rule
- Page ID
- 50807
\( \newcommand{\vecs}[1]{\overset { \scriptstyle \rightharpoonup} {\mathbf{#1}} } \)
\( \newcommand{\vecd}[1]{\overset{-\!-\!\rightharpoonup}{\vphantom{a}\smash {#1}}} \)
\( \newcommand{\id}{\mathrm{id}}\) \( \newcommand{\Span}{\mathrm{span}}\)
( \newcommand{\kernel}{\mathrm{null}\,}\) \( \newcommand{\range}{\mathrm{range}\,}\)
\( \newcommand{\RealPart}{\mathrm{Re}}\) \( \newcommand{\ImaginaryPart}{\mathrm{Im}}\)
\( \newcommand{\Argument}{\mathrm{Arg}}\) \( \newcommand{\norm}[1]{\| #1 \|}\)
\( \newcommand{\inner}[2]{\langle #1, #2 \rangle}\)
\( \newcommand{\Span}{\mathrm{span}}\)
\( \newcommand{\id}{\mathrm{id}}\)
\( \newcommand{\Span}{\mathrm{span}}\)
\( \newcommand{\kernel}{\mathrm{null}\,}\)
\( \newcommand{\range}{\mathrm{range}\,}\)
\( \newcommand{\RealPart}{\mathrm{Re}}\)
\( \newcommand{\ImaginaryPart}{\mathrm{Im}}\)
\( \newcommand{\Argument}{\mathrm{Arg}}\)
\( \newcommand{\norm}[1]{\| #1 \|}\)
\( \newcommand{\inner}[2]{\langle #1, #2 \rangle}\)
\( \newcommand{\Span}{\mathrm{span}}\) \( \newcommand{\AA}{\unicode[.8,0]{x212B}}\)
\( \newcommand{\vectorA}[1]{\vec{#1}} % arrow\)
\( \newcommand{\vectorAt}[1]{\vec{\text{#1}}} % arrow\)
\( \newcommand{\vectorB}[1]{\overset { \scriptstyle \rightharpoonup} {\mathbf{#1}} } \)
\( \newcommand{\vectorC}[1]{\textbf{#1}} \)
\( \newcommand{\vectorD}[1]{\overrightarrow{#1}} \)
\( \newcommand{\vectorDt}[1]{\overrightarrow{\text{#1}}} \)
\( \newcommand{\vectE}[1]{\overset{-\!-\!\rightharpoonup}{\vphantom{a}\smash{\mathbf {#1}}}} \)
\( \newcommand{\vecs}[1]{\overset { \scriptstyle \rightharpoonup} {\mathbf{#1}} } \)
\( \newcommand{\vecd}[1]{\overset{-\!-\!\rightharpoonup}{\vphantom{a}\smash {#1}}} \)
\(\newcommand{\avec}{\mathbf a}\) \(\newcommand{\bvec}{\mathbf b}\) \(\newcommand{\cvec}{\mathbf c}\) \(\newcommand{\dvec}{\mathbf d}\) \(\newcommand{\dtil}{\widetilde{\mathbf d}}\) \(\newcommand{\evec}{\mathbf e}\) \(\newcommand{\fvec}{\mathbf f}\) \(\newcommand{\nvec}{\mathbf n}\) \(\newcommand{\pvec}{\mathbf p}\) \(\newcommand{\qvec}{\mathbf q}\) \(\newcommand{\svec}{\mathbf s}\) \(\newcommand{\tvec}{\mathbf t}\) \(\newcommand{\uvec}{\mathbf u}\) \(\newcommand{\vvec}{\mathbf v}\) \(\newcommand{\wvec}{\mathbf w}\) \(\newcommand{\xvec}{\mathbf x}\) \(\newcommand{\yvec}{\mathbf y}\) \(\newcommand{\zvec}{\mathbf z}\) \(\newcommand{\rvec}{\mathbf r}\) \(\newcommand{\mvec}{\mathbf m}\) \(\newcommand{\zerovec}{\mathbf 0}\) \(\newcommand{\onevec}{\mathbf 1}\) \(\newcommand{\real}{\mathbb R}\) \(\newcommand{\twovec}[2]{\left[\begin{array}{r}#1 \\ #2 \end{array}\right]}\) \(\newcommand{\ctwovec}[2]{\left[\begin{array}{c}#1 \\ #2 \end{array}\right]}\) \(\newcommand{\threevec}[3]{\left[\begin{array}{r}#1 \\ #2 \\ #3 \end{array}\right]}\) \(\newcommand{\cthreevec}[3]{\left[\begin{array}{c}#1 \\ #2 \\ #3 \end{array}\right]}\) \(\newcommand{\fourvec}[4]{\left[\begin{array}{r}#1 \\ #2 \\ #3 \\ #4 \end{array}\right]}\) \(\newcommand{\cfourvec}[4]{\left[\begin{array}{c}#1 \\ #2 \\ #3 \\ #4 \end{array}\right]}\) \(\newcommand{\fivevec}[5]{\left[\begin{array}{r}#1 \\ #2 \\ #3 \\ #4 \\ #5 \\ \end{array}\right]}\) \(\newcommand{\cfivevec}[5]{\left[\begin{array}{c}#1 \\ #2 \\ #3 \\ #4 \\ #5 \\ \end{array}\right]}\) \(\newcommand{\mattwo}[4]{\left[\begin{array}{rr}#1 \amp #2 \\ #3 \amp #4 \\ \end{array}\right]}\) \(\newcommand{\laspan}[1]{\text{Span}\{#1\}}\) \(\newcommand{\bcal}{\cal B}\) \(\newcommand{\ccal}{\cal C}\) \(\newcommand{\scal}{\cal S}\) \(\newcommand{\wcal}{\cal W}\) \(\newcommand{\ecal}{\cal E}\) \(\newcommand{\coords}[2]{\left\{#1\right\}_{#2}}\) \(\newcommand{\gray}[1]{\color{gray}{#1}}\) \(\newcommand{\lgray}[1]{\color{lightgray}{#1}}\) \(\newcommand{\rank}{\operatorname{rank}}\) \(\newcommand{\row}{\text{Row}}\) \(\newcommand{\col}{\text{Col}}\) \(\renewcommand{\row}{\text{Row}}\) \(\newcommand{\nul}{\text{Nul}}\) \(\newcommand{\var}{\text{Var}}\) \(\newcommand{\corr}{\text{corr}}\) \(\newcommand{\len}[1]{\left|#1\right|}\) \(\newcommand{\bbar}{\overline{\bvec}}\) \(\newcommand{\bhat}{\widehat{\bvec}}\) \(\newcommand{\bperp}{\bvec^\perp}\) \(\newcommand{\xhat}{\widehat{\xvec}}\) \(\newcommand{\vhat}{\widehat{\vvec}}\) \(\newcommand{\uhat}{\widehat{\uvec}}\) \(\newcommand{\what}{\widehat{\wvec}}\) \(\newcommand{\Sighat}{\widehat{\Sigma}}\) \(\newcommand{\lt}{<}\) \(\newcommand{\gt}{>}\) \(\newcommand{\amp}{&}\) \(\definecolor{fillinmathshade}{gray}{0.9}\)Exceptions to the Octet Rule in Environmental Chemistry
- Free Radicals
- Electron Deficient Species
- Expanded Octets
1. Free Radicals
A free radical is a chemical species with an odd number of valance electrons—consequently, it violates the octet rule. One important example is nitrogen dioxide (NO2). Its electron dot structure is shown below.
Figure \(\PageIndex{1}\) Nitrogen Dioxide (http://chemistry.about.com/od/chemicalbonding/tp/Exceptions-To-The-Octet-Rule.htm)
As you can see, only seven electrons surround the nitrogen atom. Atoms and molecules with odd numbers of electrons are generally unstable and reactive. The reactivity of free radicals plays a very important role in atmospheric chemistry, especially in the formation of photochemical smog.
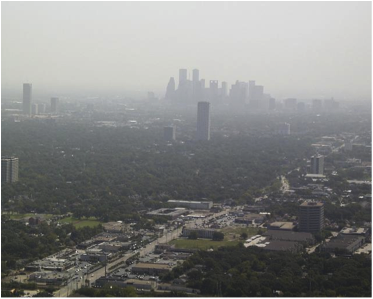
Photochemical smog (shown above) is a visible haze in the atmosphere that is harmful to both plants and animals [1]. In humans, it irritates the eyes and lungs. One main source of photochemical smog is automobiles [2]. The combustion of fuel in automobile engines produces carbon monoxide, carbon dioxide, nitrogen monoxide, nitrogen dioxide, and uncombusted hydrocarbons [2]. The role of these last three molecules in the formation of photochemical smog will be discussed later.
The species that is perhaps most central to the formation of photochemical smog is called the hydroxyl radical [1]. The dot structure of the hydroxyl radical is shown below.
Figure \(\PageIndex{3}\) Hydroxyl Radical(http://www.scienceinschool.org/print/1041)
The hydroxyl radical can also be represented as •OH, with the dot next to the oxygen representing the odd electron. Similar to nitrogen in the NO2 molecule, oxygen is only surrounded by seven valence electrons. Since the hydroxyl radical will be far more stable if it obtains an eighth electron, it is very reactive. When it reacts with another molecule, it takes away a single electron, causing the other molecule to become a free radical. One example, shown below, is the reaction of hydroxyl radical with a hydrocarbon, which, as discussed earlier, may be present in the atmosphere from unburned automobile fuel.
Figure \(\PageIndex{4}\) Reaction Involving Hydroxyl Radical (http://mtweb.mtsu.edu/nchong/Smog-Atm1.htm)
In the reaction above, R represents any hydrocarbon (e.g. ethane, CH3CH3). The hydroxyl radical removes a hydrogen atom (including its electron) to form a stable water molecule [1]. As shown in the second reaction, the newly formed hydrocarbon radical (R•) can quickly react with oxygen (O2), which is abundant in the atmosphere, to form a peroxide radical (ROO•).
Peroxide radicals can then react with nitrogen monoxide (NO) to form nitrogen dioxide, which can lead to the creation of yet more hydroxyl radicals [1]. Or, peroxide radicals can instead react with nitrogen monoxide and diatomic oxygen, forming aldehydes, as well as more hydroxyl radicals.
Aldehydes can react with hydroxyl radicals, ultimately leading to the formation of substances called peroxyacetic nitric anhydrides (PAN) [1]. PANs are the most significant eye irritants in smog. Not to mention, after traveling long distances, PANs can degrade to form nitrogen dioxide, ozone, and hydroxyl radicals—all species that contribute to unhealthy photochemical smog.
The reaction sequences described above are just a small sampling of the many reaction pathways that atmospheric free radicals can follow. In general, it is important to remember that free radicals are very reactive species that can lead to the formation of the various components of photochemical smog. Next, we will look at how hydroxyl radicals are formed in the first place.
The formation of hydroxyl radicals in the atmosphere largely results from reactions involving diatomic nitrogen (N2), diatomic oxygen (O2), and uncombusted hydrocarbons [1]. First, nitrogen and oxygen must react with each other to form nitrogen monoxide.
\[\ce{N2 + O2 <-> 2NO}\nonumber\]
Since this reversible reaction requires a significant input of energy in order to proceed right, it only occurs in high temperature environments like the inside of a car engine [1].
Nitrogen monoxide must next be oxidized to form nitrogen dioxide [1]. This occurs when nitrogen monoxide reacts with a peroxide radical, mentioned earlier.
\[\ce{NO + ROO \cdot -> RO \cdot + NO2}\nonumber\]
Again, as a free radical, NO2 is relatively unstable. Therefore, it is readily cleaved by electromagnetic radiation from the sun [1].
\[\ce{NO2 + hv -> NO + O}\nonumber\]
(λ < 400 nm)
As indicated in the reaction equation, the wavelength of electromagnetic radiation from the sun must be shorter than 400 nm in order to cleave the nitrogen-oxygen bond in NO2 [1].
Next, the lone oxygen atom reacts with diatomic oxygen in the presence of a third neutral species (M) to form an ozone molecule (O3) [1].
\[\ce{O2 + O + M -> O3 + M}\nonumber\]
This is the same ozone that forms the protective ozone layer in the stratosphere. However, lower in the atmosphere, ozone is harmful. It is toxic to humans and also results in the formation of hydroxyl radicals. Below are the resonance structures of ozone.
Figure \(\PageIndex{5}\) Ozone (http://www.mikeblaber.org/oldwine/chm1045/notes/Bonding/Resonan/Bond07.htm)
Ozone is very reactive and can react directly with other species in the atmosphere [2]. Or, like NO2, the relatively weak bonds in ozone can be cleaved by light. In the case of ozone, the bonds are stronger, so the light must have a minimum wavelength of 315 nm [1].
\[\ce{O3 + hv -> O2* + O*}\nonumber\]
The asterisk indicates that the oxygen atom produced is in an excited state, making it more reactive [1]. This excited oxygen atom can react with a water molecule to form two hydroxyl radicals.
\[\ce{O* + H2O -> 2 \cdot OH}\nonumber\]
Overall, each NO2 molecule can result in the formation of two hydroxyl radicals according to this reaction mechanism [1]. Because of their denser populations and greater use of automobiles, urban areas have approximately 100 times the concentration of NO2 compared to rural areas [1]. Consequently, urban areas also have greater concentrations of hydroxyl radicals, as well as the associated components of photochemical smog. This is a major reason why the air in cities is generally regarded as “unhealthy.”
Besides creating smog, hydroxyl radicals can also contribute to acid rain [2]. When hydroxyl radicals react with nitrogen dioxide, they form nitric acid.
\[\ce{\cdot OH + NO2 -> HNO3}\nonumber\]
Like smog, acid rain is harmful to living systems [2]. For example, some freshwater lakes in southeastern Canada and the northeastern United States have become too acidic to support life [2]. Additionally, acid rain can cause damage to human-made structures, especially those made out of calcium carbonate, which reacts with acidic substances.
2. Electron-Deficient Species
Not all species that are short of an octet of valence electrons have an odd number of electrons. Hydrogen and helium atoms only need two valence electrons to fill their 1s orbitals. Beryllium appears to be stable with four valence electrons, while boron atoms form stable molecules in which they appear to have only six valence electrons. An example of these “electron-deficient species” is boron trifluoride (BF3), shown below.
Figure \(\PageIndex{6}\) Boron Trifluoride (www.chemeddl.org/alfresco/ser...s=3&guest=true)
Though recent studies indicate that double bonding may actually be important in the structure of BF3, this molecule acts as if it is electron-deficient, which is evidence supporting the structure shown above [2].
Boron trifluoride is a very reactive and toxic gas. However, it has proved an important resource for monitoring the environment. BF3 is used in devices called neutron proportional counters, which are used to monitor radiation coming from nuclear waste [3]. This device is shown in the picture below.
Figure \(\PageIndex{7}\) Neutron Proportional Counters (www.centronic.co.uk/boron.htm)
Neutron proportional counters detect neutron radiation when the neutrons undergo nuclear reactions with the boron, forming lithium atoms and alpha particles (α) [4]. This reaction is presented below.
\[{}_{\text{0}}^{\text{1}}\text{n } + {}_{\text{5}}^{\text{10}}\text{B} \rightarrow \text{ }{}_{\text{3}}^{\text{7}}\text{Li} + {}_{\text{2}}^{\text{4}} \alpha \nonumber \]
Noble gases can then be used to count the alpha particles produced by this reaction [4].
In sufficient quantity, nuclear radiation can be harmful to living things. Thus, it is important that humans continually monitor the environment for radioactivity, especially in areas where nuclear power is produced and nuclear waste is stored.
3. Expanded Octets
Finally, some atoms are able to accommodate more than eight valence electrons. While elements in the first two periods only have access to the 1s, 2s, and 2p energy sublevels, elements in the third period or higher have access to d orbitals. For example, on the third energy level, there are 3s, 3p, and 3d orbitals. Consequently, an atom on the third energy level has room for more than eight electrons.
An example of a species that breaks the octet rule in this way is sulfur hexafluoride (SF6). Its structure is shown below.
Figure \(\PageIndex{8}\) Sulfur Hexafluoride (www.uwplatt.edu/~sundin/114/plSF6.htm)
Unfortunately, the SF6 molecule appears to be very stable. This is unfortunate because the Intergovernmental Panel on Climate Change (IPCC) reported that SF6 had, by far, the greatest global warming potential as a greenhouse gas [5]. The IPCC also reported that its lifetime was 3,200 years.
Measurements taken on Mauna Loa, Hawaii indicate that the levels of SF6 in the atmosphere have been increasing steadily since the year 2000 [6]. The data is presented in the graph below.

The only practical way to limit sulfur hexafluoride from further contributing to the greenhouse effect is to stop its production. It is used in electric power transmission and distribution, in the magnesium industry, in the production of silicon semiconductors, and in leak detection [7]. While the uses of SF6 may be important to industry, it is worth reconsidering their use in light of this gas’s longevity and global warming potential.
References:
- [1] Boehmler D. 2012. Unit 2: Combustion. [Online course notes]. 2012 Jun 10.
- [2] Zumdahl SS, Zumdahl SA. Chemistry. 6th ed. New York: Houghton Mifflin Company; 2003.
- [3] Centronic. 2011. BF3 neutron proportional counters. [Online]. Available from: www.centronic.co.uk/boron.htm. Accessed 2012 Jun.
- [4] Frame P. 2007. [Online]. Available from: http://www.orau.org/ptp/collection/proportional%20counters/introprops.htm. Accessed 2012 Jun 10.
- [5] Intergovernmental Panel on Climate Change. 2007. IPCC fourth assessment report: Climate change 2007. [Online]. Available from: http://www.ipcc.ch/publications_and_data/ar4/wg1/en/ch2s2-10-2.html. Accessed 2012 Jun 10.
- [6] National Oceanic and Atmospheric Association. 2012. Chromatograph for atmospheric trace species. [Online]. Available from: www.esrl.noaa.gov/gmd/hats/in...nc/mlosf6.html. Accessed 2012 Jun 10.
- [7] U.S. Environmental Protection Agency. [Online]. High global warming potential gases. Available from: www.epa.gov/highgwp/scientific.html. Accessed 2012 Jun 10.
Contributors and Attributions
Ed Vitz (Kutztown University), John W. Moore (UW-Madison), Justin Shorb (Hope College), Xavier Prat-Resina (University of Minnesota Rochester), Tim Wendorff, and Adam Hahn.