P15.1: Show a mechanism for each of the redox reactions below. Do not abbreviate the reactive parts of the redox coenzyme.
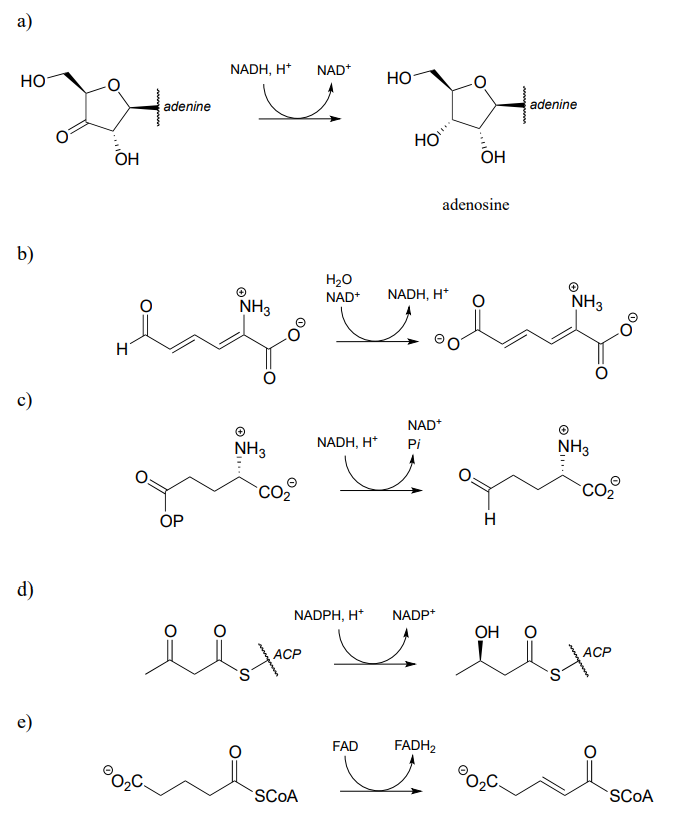
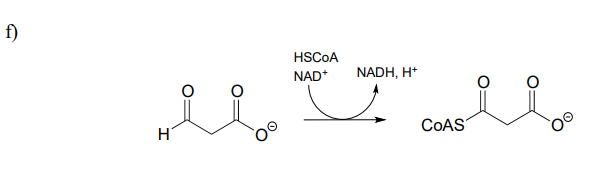
g) regeneration of reduced flavin by \(NADH\)):
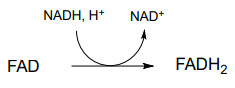
h) In reaction (a), near which face of the substrate is the cofactor bound in the active site?
i) In reaction (d), near which face of the product is the cofactor bound in the active site?
P15.2: In the reactions below ((EC 2.7.2.4; EC 1.2.1.11) , the side chain of aspartate is altered, but the main peptide chain is not affected. Show the most probable structure of species A and B.
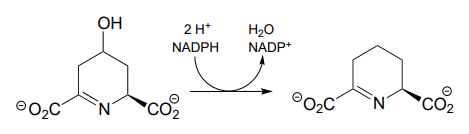
P15.3: Propose complete mechanisms for the following reactions.
- (EC 1.3.1.26, from lysine biosynthesis)
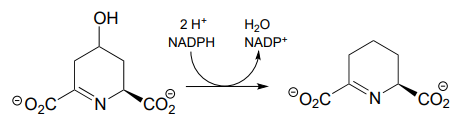
- (EC 1.1.1.205, from guanosine ribonucleotide biosynthesis) The mechanism involves a covalent cysteine-linked enzyme-substrate intermediate.

P15.4: The first step in the lysine degradation pathway is a reductive condensation with a-ketoglutarate to form an intermediate called saccharopine. (EC 1.5.1.8)
- Propose a mechanism for this transformation.

- Saccharopine (see part (a) above) is then broken up to yield glutamate and a second product that contains an aldehyde group. Predict the structure of this second product, and propose a likely mechanism for the reaction which involves and imine intermediate. (EC 1.5.1.10)
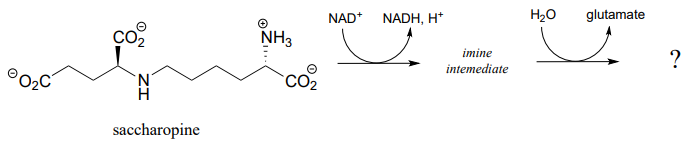
P15.5: Predict the structures of species A and B in the pathway below.
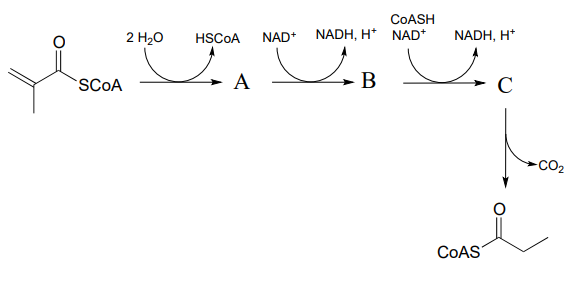
P15.6: Bilirubin, the molecule responsible for the yellowish color of bruises, is formed from the \(NADPH\)-dependent hydrogenation of a double bond in biliverdin (EC 1.3.1.24), which is a product of heme degradation (heme is an iron-containing coenzyme in the oxygen-carrying blood protein hemoglobin). Draw a likely mechanism for this reaction.
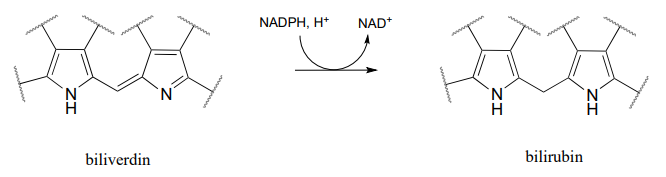
P15.7: Draw a likely mechanism for each of the reactions below.
- From the oxidation of polyunsaturated fatty acids:
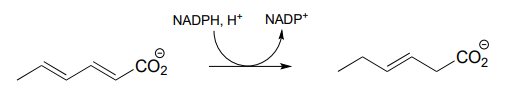
-

P15.8: Predict the structures of pathway intermediates A, B, and C:

P15.9: An enzyme called DsbA (EC1.8.4.2) is responsible for the formation of disulfide bonds in bacterial proteins. The process - which can be thought of as a 'disulfide exchange', involves the cleavage of a disulfide bond between two active site cysteines in DsbA. It is accomplished through two successive \(S_N2\) displacements.
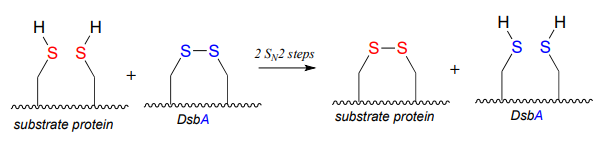
DsbA is then returned to it's starting (disulfide) state through a second disulfide exchange reaction with another protein called DsbB:
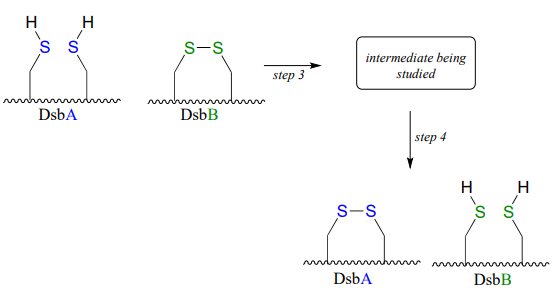
Scientists were interested in studying the intermediate species formed in step 3, but found that it is very short-lived and difficult to isolate. In order to address this problem, they ran the reaction with a synthetic analog of DsbB that contained an unnatural bromoalanine amino acid in place of one of the active site cysteines.
- Draw a complete mechanism for the disulfide exchange reaction between DsbA and DsbB.
- Show how the bromoalanine-containing DsbB analog allowed for the isolation of an intermediate that resembles the true, short-lived intermediate. (J. Am. Chem. Soc. 2004, 126, 15060; J. Nat. Prod. 2001, 64, 597).
P15.10:
- In chapter 16 we will learn how ascorbate (vitamin C) acts as a 'radical scavenger' antioxidant to protect our cells from damage by free radical species. When ascorbate scavenges a radical, it ends up being converted to dehydroascorbate . One possible metabolic fate of dehydroascorbate is to be recycled back to ascorbate through an enzyme-free reaction with glutathione. Biocemistry 1999, 38, 268.
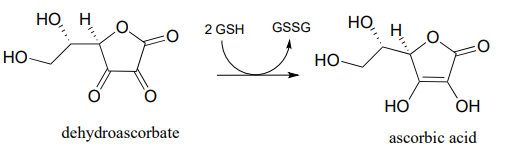
Suggest a likely mechanism for the enzyme-free reaction.
- In the introduction to chapter 16, we will learn that most animals - but not humans - are able to synthesize their own ascorbate. Humans cannot synthesize vitamin C because we lack the enzyme for the final step in the biosynthetic pathway, gulonolactone oxidase:
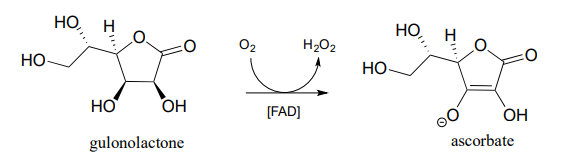
This enzyme uses \(FAD\) as an oxidizing agent, and \(FADH_2\) is oxidized back to \(FAD\) at the end of the catalytic cycle by molecular oxygen, with hydrogen peroxide as a side product. Draw out a likely mechanism showing how gulonolactone is converted to ascorbate, and how \(FAD\) is regenerated.
- Artemisinin is a naturally occurring compound with demonstrated antimalarial properties. It is thought to act by depleting the malaria-causing microbe's store of reduced flavin, thus disrupting the redox balance. The relevant reaction is shown below:
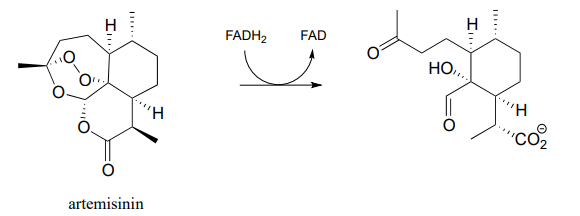
Draw mechanistic arrows for the step as shown above. (Molecules 2010, 15, 1705)
- Draw the mechanistic step in the reaction below in which the C-O bond indicated by the arrow in the figure below is formed.
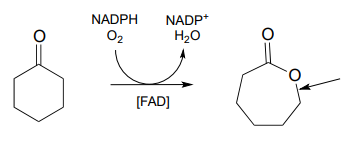
P15.11: Methanogens are a class of microorganisms in the domain archaea which inhabit a diversity of anaerobic (oxygen-lacking) environments, from the intestines of humans, to swamp mud, to the base of deep sea hot water vents. They obtain energy by reducing carbon dioxide to methane:
\[CO_2 + 4H_2 \rightarrow CH_4 + 2H_2O + \text{energy}\]
Methanogenesis', like the oxidation of glucose in animals, is not accomplished in a single reaction - it requires a long series of enzymatic steps, and involves the participation of several unique coenzymes (if you are interested in learning more, see FEMS Microbiol. Rev. 1999, 23, 13 for a detailed review of the enzymatic reactions of methanogenesis).
The oxidation of methane to carbon dioxide when you burn natural gas for heating your house is obviously an exergonic process. How then is it possible that reducing carbon dioxide to methane could also be exergonic? Explain.
Multistep pathway prediction problems
In chapters 12 and 13, we were introduced to the challenge of mapping out potential multi-step transformations using the retrosynthesis approach, where we start with the more complex molecule and take it apart step be step. These problems have necessarily involved hypothetical, generalized transformations, because many of the reaction types involved in actual biochemical transformations were as-yet unfamiliar to us.
We have now reached a point in our study of organic reactivity where we can look at an actual metabolic pathway and probably recognize most of the reactions taking place - so were are ready to try our hand at mapping out real metabolic pathways.
Before we dive in, you may want to go back to the retrosynthesis interchapter and review the key elements of the retrosynthetic approach. You may (or may not) also need a little more practice with some simpler, shorter, hypothetical problems, similar to what we worked on in the last three chapters but incorporating some of the redox reactions that we have just finished learning about. These are provided in problems 15.12 and 15.13 below.
As before, your job is to draw out a 'pathway diagram' for each transformation, using the 'arrow in - arrow out' convention to indicate the role of other necessary participants in each reaction, such as ATP, \(NADH\), water, or another organic molecule. An example for a simple two-step pathway is provided in problem 15.12 below. A three-step pathway would of course show two intermediate compounds.
Remember, it is most important that your proposed pathway be chemically reasonable - in other words, each hypothetical reaction that you propose should be very similar to a reaction pattern that we have seen in chapters 8-15. You should be able to put a name on each step: for example, 'step 1 is a Claisen condensation; step 2 is a ketone reduction', and so forth.
Also remember that there is usually no one correct way to approach problems like this - they are puzzles to solve, and success will be dependent in large part on having a solid grasp on the chemical 'tools' available to us: in other words, the biochemical reaction types that we have been studying, starting with nucleophilic substitutions in chapter 8.
P15.12: Hypothetical 2-step transformations:
Each of the generalized transformations below would be expected to require two enzymatic steps. Draw a reasonable pathway diagram for each transformation.
Example:
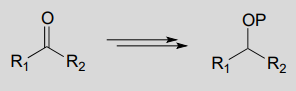
Diagram:

The first step is a nicotinamide-dependent ketone reduction/hydrogenation, and step 2 is ATP-dependent phosphorylation (ie. a kinase reaction). Note that the reducing agent in the first step could also be \(NADPH\))


P15.13: Hypothetical 3-step transformations:
Each of the generalized transformations below would be expected to require three enzymatic steps. Draw a reasonable pathway diagram for each transformation.
P15.14: Now, let's try our hand at predicting the steps of some actual metabolic pathways. For each, draw a complete pathway diagram. As needed, use any of the coenzymes we have studied, water, and ammonia.
Note: you will probably find these quite challenging! Do not expect to be able to figure them out in a few minutes - rather, think of them as puzzles to work on over a period of time, sharing ideas and strategies with classmates. Remember, if one side of the transformation is larger or more complex, start there and work towards the simpler molecules. It is also a good idea, when applicable, to start the process by a) counting carbons on each side of the transformation, and b) identifying the key bond being formed (or broken) in the transformation.

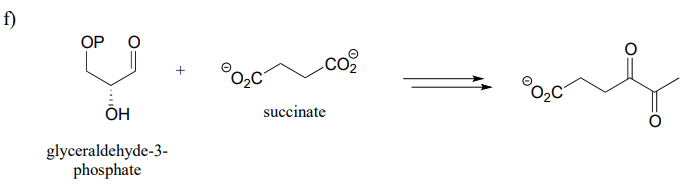
P15.15: Propose a pathway diagram for each of the metabolic pathways below. Note that some pathways contain steps that will be unfamiliar to you, and are therefore provided already.
- one cycle of fatty acid biosynthesis:

- one cycle of fatty acid degradation:
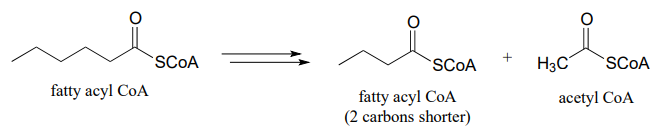
- Diabetics and people who adopt an extreme low-carbohydrate diet sometimes have breath that smells like acetone, due to 'ketone body' formation that occurs when acetyl-\(CoA\) from fatty acid oxidation (see part (b) above) is not able to enter into the citric acid cycle. Draw a pathway diagram showing how three molecules of excess acetyl \(CoA\) combine to form acetone (all three acetyl-\(CoA\) molecules first link together, but one is left over at the end of the process).

- Pentose phosphate pathway (oxidative branch):
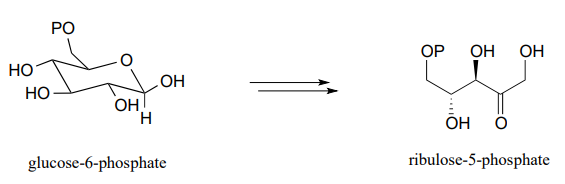
- Citric acid (Krebs) cycle:
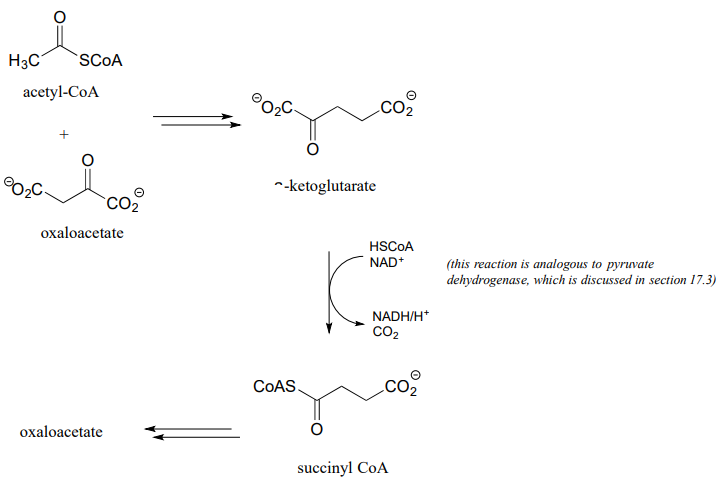
- proline biosynthesis:
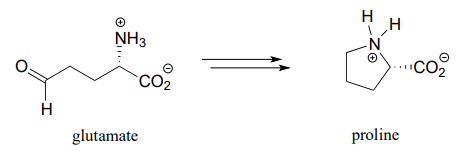
- First half of lysine biosynthesis:
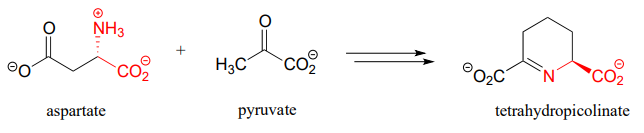
- From the biosynthesis of membrane lipid in archaea: J. Bacteriol. 2003, 185, 1181
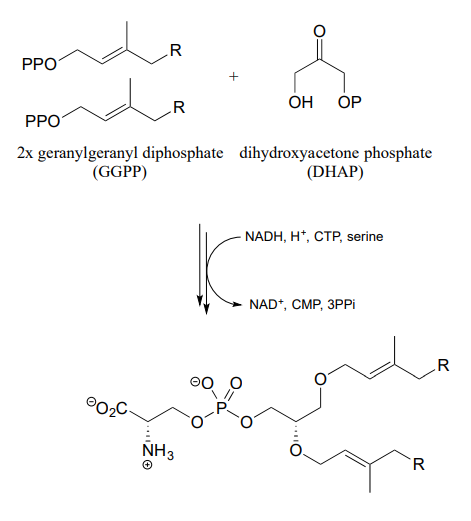