10.7: Electrons and Light
- Page ID
- 476562
\( \newcommand{\vecs}[1]{\overset { \scriptstyle \rightharpoonup} {\mathbf{#1}} } \)
\( \newcommand{\vecd}[1]{\overset{-\!-\!\rightharpoonup}{\vphantom{a}\smash {#1}}} \)
\( \newcommand{\id}{\mathrm{id}}\) \( \newcommand{\Span}{\mathrm{span}}\)
( \newcommand{\kernel}{\mathrm{null}\,}\) \( \newcommand{\range}{\mathrm{range}\,}\)
\( \newcommand{\RealPart}{\mathrm{Re}}\) \( \newcommand{\ImaginaryPart}{\mathrm{Im}}\)
\( \newcommand{\Argument}{\mathrm{Arg}}\) \( \newcommand{\norm}[1]{\| #1 \|}\)
\( \newcommand{\inner}[2]{\langle #1, #2 \rangle}\)
\( \newcommand{\Span}{\mathrm{span}}\)
\( \newcommand{\id}{\mathrm{id}}\)
\( \newcommand{\Span}{\mathrm{span}}\)
\( \newcommand{\kernel}{\mathrm{null}\,}\)
\( \newcommand{\range}{\mathrm{range}\,}\)
\( \newcommand{\RealPart}{\mathrm{Re}}\)
\( \newcommand{\ImaginaryPart}{\mathrm{Im}}\)
\( \newcommand{\Argument}{\mathrm{Arg}}\)
\( \newcommand{\norm}[1]{\| #1 \|}\)
\( \newcommand{\inner}[2]{\langle #1, #2 \rangle}\)
\( \newcommand{\Span}{\mathrm{span}}\) \( \newcommand{\AA}{\unicode[.8,0]{x212B}}\)
\( \newcommand{\vectorA}[1]{\vec{#1}} % arrow\)
\( \newcommand{\vectorAt}[1]{\vec{\text{#1}}} % arrow\)
\( \newcommand{\vectorB}[1]{\overset { \scriptstyle \rightharpoonup} {\mathbf{#1}} } \)
\( \newcommand{\vectorC}[1]{\textbf{#1}} \)
\( \newcommand{\vectorD}[1]{\overrightarrow{#1}} \)
\( \newcommand{\vectorDt}[1]{\overrightarrow{\text{#1}}} \)
\( \newcommand{\vectE}[1]{\overset{-\!-\!\rightharpoonup}{\vphantom{a}\smash{\mathbf {#1}}}} \)
\( \newcommand{\vecs}[1]{\overset { \scriptstyle \rightharpoonup} {\mathbf{#1}} } \)
\( \newcommand{\vecd}[1]{\overset{-\!-\!\rightharpoonup}{\vphantom{a}\smash {#1}}} \)
\(\newcommand{\avec}{\mathbf a}\) \(\newcommand{\bvec}{\mathbf b}\) \(\newcommand{\cvec}{\mathbf c}\) \(\newcommand{\dvec}{\mathbf d}\) \(\newcommand{\dtil}{\widetilde{\mathbf d}}\) \(\newcommand{\evec}{\mathbf e}\) \(\newcommand{\fvec}{\mathbf f}\) \(\newcommand{\nvec}{\mathbf n}\) \(\newcommand{\pvec}{\mathbf p}\) \(\newcommand{\qvec}{\mathbf q}\) \(\newcommand{\svec}{\mathbf s}\) \(\newcommand{\tvec}{\mathbf t}\) \(\newcommand{\uvec}{\mathbf u}\) \(\newcommand{\vvec}{\mathbf v}\) \(\newcommand{\wvec}{\mathbf w}\) \(\newcommand{\xvec}{\mathbf x}\) \(\newcommand{\yvec}{\mathbf y}\) \(\newcommand{\zvec}{\mathbf z}\) \(\newcommand{\rvec}{\mathbf r}\) \(\newcommand{\mvec}{\mathbf m}\) \(\newcommand{\zerovec}{\mathbf 0}\) \(\newcommand{\onevec}{\mathbf 1}\) \(\newcommand{\real}{\mathbb R}\) \(\newcommand{\twovec}[2]{\left[\begin{array}{r}#1 \\ #2 \end{array}\right]}\) \(\newcommand{\ctwovec}[2]{\left[\begin{array}{c}#1 \\ #2 \end{array}\right]}\) \(\newcommand{\threevec}[3]{\left[\begin{array}{r}#1 \\ #2 \\ #3 \end{array}\right]}\) \(\newcommand{\cthreevec}[3]{\left[\begin{array}{c}#1 \\ #2 \\ #3 \end{array}\right]}\) \(\newcommand{\fourvec}[4]{\left[\begin{array}{r}#1 \\ #2 \\ #3 \\ #4 \end{array}\right]}\) \(\newcommand{\cfourvec}[4]{\left[\begin{array}{c}#1 \\ #2 \\ #3 \\ #4 \end{array}\right]}\) \(\newcommand{\fivevec}[5]{\left[\begin{array}{r}#1 \\ #2 \\ #3 \\ #4 \\ #5 \\ \end{array}\right]}\) \(\newcommand{\cfivevec}[5]{\left[\begin{array}{c}#1 \\ #2 \\ #3 \\ #4 \\ #5 \\ \end{array}\right]}\) \(\newcommand{\mattwo}[4]{\left[\begin{array}{rr}#1 \amp #2 \\ #3 \amp #4 \\ \end{array}\right]}\) \(\newcommand{\laspan}[1]{\text{Span}\{#1\}}\) \(\newcommand{\bcal}{\cal B}\) \(\newcommand{\ccal}{\cal C}\) \(\newcommand{\scal}{\cal S}\) \(\newcommand{\wcal}{\cal W}\) \(\newcommand{\ecal}{\cal E}\) \(\newcommand{\coords}[2]{\left\{#1\right\}_{#2}}\) \(\newcommand{\gray}[1]{\color{gray}{#1}}\) \(\newcommand{\lgray}[1]{\color{lightgray}{#1}}\) \(\newcommand{\rank}{\operatorname{rank}}\) \(\newcommand{\row}{\text{Row}}\) \(\newcommand{\col}{\text{Col}}\) \(\renewcommand{\row}{\text{Row}}\) \(\newcommand{\nul}{\text{Nul}}\) \(\newcommand{\var}{\text{Var}}\) \(\newcommand{\corr}{\text{corr}}\) \(\newcommand{\len}[1]{\left|#1\right|}\) \(\newcommand{\bbar}{\overline{\bvec}}\) \(\newcommand{\bhat}{\widehat{\bvec}}\) \(\newcommand{\bperp}{\bvec^\perp}\) \(\newcommand{\xhat}{\widehat{\xvec}}\) \(\newcommand{\vhat}{\widehat{\vvec}}\) \(\newcommand{\uhat}{\widehat{\uvec}}\) \(\newcommand{\what}{\widehat{\wvec}}\) \(\newcommand{\Sighat}{\widehat{\Sigma}}\) \(\newcommand{\lt}{<}\) \(\newcommand{\gt}{>}\) \(\newcommand{\amp}{&}\) \(\definecolor{fillinmathshade}{gray}{0.9}\)- Understand how light interacts with electrons to change their energy levels.
- Explain how atomic emission spectrum is different than the spectrum of light from the Sun.
Electrons turn out to be the cause of chemical reactions. This makes a lot of sense if you consider that the electrons are the part of an atom which are on the outside, and therefore most likely to collide with another atom that could lead to a chemical reaction. But electrons behave much differently than the macroscopic matter that we are familiar with. We will develop a bit of an understanding of this behavior throughout the rest of this chapter, and then connect that back to the Periodic Table.
Atomic Emission Spectra
The electrons in an atom tend to be arranged in such a way that the energy of the atom is as low as possible. The ground state of an atom is the lowest energy state of the atom. When those atoms are given energy, the electrons absorb the energy and move to a higher energy level. These energy levels of the electrons in atoms are quantized, meaning again that the electron must move from one energy level to another in discrete steps, rather than continuously. An excited state of an atom is a state where its potential energy is higher than the ground state. An atom in the excited state is not stable. When it returns back to the ground state, it releases the energy that it had previously gained in the form of electromagnetic radiation.
So how do atoms gain energy in the first place? One way is to pass an electric current through an enclosed sample of a gas at low pressure. Since the electron energy levels are unique for each element, every gas discharge tube will glow with a distinctive color, depending on the identity of the gas (see below).
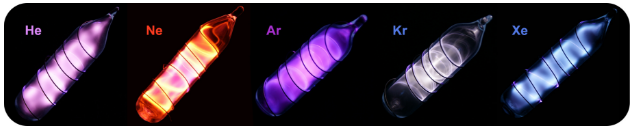
"Neon" signs are familiar examples of gas discharge tubes. However, only signs that glow with the red-orange color seen in the figure are actually filled with neon. Signs of other colors contain different gases or mixtures of gases.
Scientists studied the distinctive pink color of the gas discharge created by hydrogen gas. When a narrow beam of this light was viewed through a prism, the light was separated into four lines of very specific wavelengths (and frequencies since \(\lambda\) and \(\nu\) are inversely related). An atomic emission spectrum is the pattern of lines formed when light passes through a prism to separate it into the different frequencies of light it contains. The figure below shows the atomic emission spectrum of hydrogen. Notice how this behavior differs from the continuous spectrum of visible light that we discussed earlier in this text. This difference was a challenge to solve and resulted in several models of electron behavior, each of which was more bizarre than the one before it. The laws of nature work very different on the scale of electrons than they do in the world we are used to.
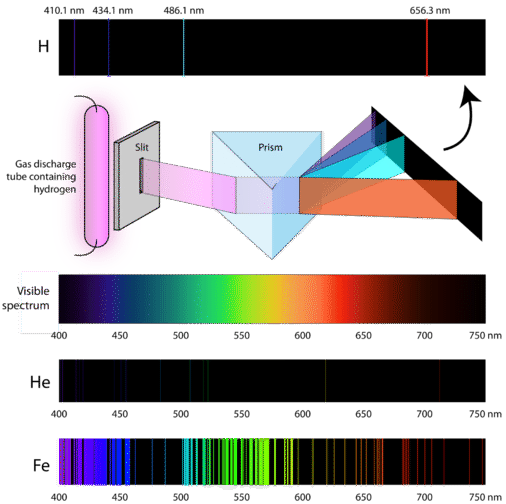
Bohr's Atomic Model
Following the discoveries of hydrogen emission spectra and the photoelectric effect, the Danish physicist Niels Bohr (1885-1962) proposed a new model of the atom in 1915. Bohr proposed that electrons do not radiate energy as they orbit the nucleus, but exist in states of constant energy that he called stationary states. This means that the electrons orbit at fixed distances from the nucleus (see below). Bohr's work was primarily based on the emission spectra of hydrogen. This is also referred to as the planetary model of the atom. It explained the inner workings of the hydrogen atom. Bohr was awarded the Nobel Prize in physics in 1922 for his work.

Bohr explained that electrons can be moved into different orbits with the addition of energy. When the energy is removed, the electrons return back to their ground state, emitting a corresponding amount of energy—a quantum of light, or photon. This was the basis for what later became known as quantum theory. This is a theory based on the principle that matter and energy have the properties of both particles and waves. It accounts for a wide range of physical phenomena, including the existence of discrete packets of energy and matter, the uncertainty principle, and the exclusion principle.
According to the Bohr model, often referred to as a planetary model, the electrons encircle the nucleus of the atom in specific allowable paths called orbits. When the electron is in one of these orbits, its energy is fixed. The ground state of the hydrogen atom, where its energy is lowest, is when the electron is in the orbit that is closest to the nucleus. The orbits that are further from the nucleus are all of successively greater energy. The electron is not allowed to occupy any of the spaces in between the orbits. An everyday analogy to the Bohr model is the rungs of a ladder. As you move up or down a ladder, you can only occupy specific rungs and cannot be in the spaces in between rungs. Moving up the ladder increases your potential energy, while moving down the ladder decreases your energy.
Based on the wavelengths of the spectral lines, Bohr was able to calculate the energies that the hydrogen electron would have in each of its allowed energy levels. He then mathematically showed which energy level transitions correspond to the spectral lines in the atomic emission spectrum, as shown in Figure \(\PageIndex{4}\).
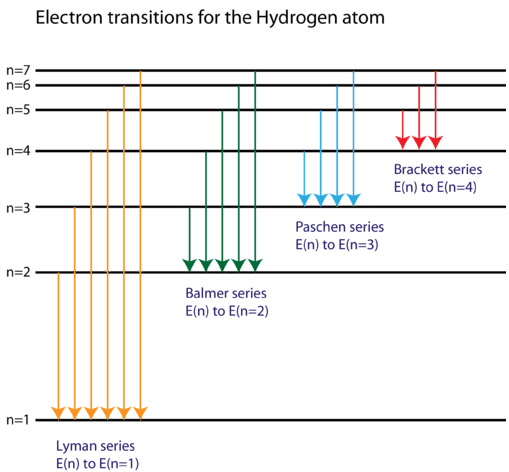
Bohr's model was a tremendous success in explaining the spectrum of the hydrogen atom. Unfortunately, when the mathematics of the model were applied to atoms with more than one electron, it was not able to correctly predict the frequencies of the spectral lines. While Bohr's model represented a great advancement in the atomic model and the concept of electron transitions between energy levels is valid, improvements were needed in order to fully understand all atoms and their chemical behavior. Shortly after Bohr published his planetary model of the atom, several new discoveries were made, which resulted in, yet again, a revised view of the atom.
Summary
- Atomic emission spectra are produced when excited electrons return to the ground state.
- The emitted light of electrons corresponds to energies of the specific electrons.
- The Bohr model postulates that electrons orbit the nucleus at fixed energy levels.
- Orbits further from the nucleus exist at higher energy levels.
- When electrons return to a lower energy level, they emit energy in the form of light.
- Emission lines for hydrogen correspond to energy changes related to electron transitions.
- The Bohr model works only for the hydrogen atom.
Glossary
- ground state
- lowest energy state of the atom
- excited state
- state where its potential energy is higher than the ground state
- atomic emission spectrum
- pattern of lines formed when light emitted from an excited atom passes through a prism to separate it into the different frequencies of light it contains
- electron transitions
- process by which electrons move between different allowable energy levels within the atom.