23.2: Carbohydrates
- Page ID
- 55122
\( \newcommand{\vecs}[1]{\overset { \scriptstyle \rightharpoonup} {\mathbf{#1}} } \)
\( \newcommand{\vecd}[1]{\overset{-\!-\!\rightharpoonup}{\vphantom{a}\smash {#1}}} \)
\( \newcommand{\id}{\mathrm{id}}\) \( \newcommand{\Span}{\mathrm{span}}\)
( \newcommand{\kernel}{\mathrm{null}\,}\) \( \newcommand{\range}{\mathrm{range}\,}\)
\( \newcommand{\RealPart}{\mathrm{Re}}\) \( \newcommand{\ImaginaryPart}{\mathrm{Im}}\)
\( \newcommand{\Argument}{\mathrm{Arg}}\) \( \newcommand{\norm}[1]{\| #1 \|}\)
\( \newcommand{\inner}[2]{\langle #1, #2 \rangle}\)
\( \newcommand{\Span}{\mathrm{span}}\)
\( \newcommand{\id}{\mathrm{id}}\)
\( \newcommand{\Span}{\mathrm{span}}\)
\( \newcommand{\kernel}{\mathrm{null}\,}\)
\( \newcommand{\range}{\mathrm{range}\,}\)
\( \newcommand{\RealPart}{\mathrm{Re}}\)
\( \newcommand{\ImaginaryPart}{\mathrm{Im}}\)
\( \newcommand{\Argument}{\mathrm{Arg}}\)
\( \newcommand{\norm}[1]{\| #1 \|}\)
\( \newcommand{\inner}[2]{\langle #1, #2 \rangle}\)
\( \newcommand{\Span}{\mathrm{span}}\) \( \newcommand{\AA}{\unicode[.8,0]{x212B}}\)
\( \newcommand{\vectorA}[1]{\vec{#1}} % arrow\)
\( \newcommand{\vectorAt}[1]{\vec{\text{#1}}} % arrow\)
\( \newcommand{\vectorB}[1]{\overset { \scriptstyle \rightharpoonup} {\mathbf{#1}} } \)
\( \newcommand{\vectorC}[1]{\textbf{#1}} \)
\( \newcommand{\vectorD}[1]{\overrightarrow{#1}} \)
\( \newcommand{\vectorDt}[1]{\overrightarrow{\text{#1}}} \)
\( \newcommand{\vectE}[1]{\overset{-\!-\!\rightharpoonup}{\vphantom{a}\smash{\mathbf {#1}}}} \)
\( \newcommand{\vecs}[1]{\overset { \scriptstyle \rightharpoonup} {\mathbf{#1}} } \)
\( \newcommand{\vecd}[1]{\overset{-\!-\!\rightharpoonup}{\vphantom{a}\smash {#1}}} \)
\(\newcommand{\avec}{\mathbf a}\) \(\newcommand{\bvec}{\mathbf b}\) \(\newcommand{\cvec}{\mathbf c}\) \(\newcommand{\dvec}{\mathbf d}\) \(\newcommand{\dtil}{\widetilde{\mathbf d}}\) \(\newcommand{\evec}{\mathbf e}\) \(\newcommand{\fvec}{\mathbf f}\) \(\newcommand{\nvec}{\mathbf n}\) \(\newcommand{\pvec}{\mathbf p}\) \(\newcommand{\qvec}{\mathbf q}\) \(\newcommand{\svec}{\mathbf s}\) \(\newcommand{\tvec}{\mathbf t}\) \(\newcommand{\uvec}{\mathbf u}\) \(\newcommand{\vvec}{\mathbf v}\) \(\newcommand{\wvec}{\mathbf w}\) \(\newcommand{\xvec}{\mathbf x}\) \(\newcommand{\yvec}{\mathbf y}\) \(\newcommand{\zvec}{\mathbf z}\) \(\newcommand{\rvec}{\mathbf r}\) \(\newcommand{\mvec}{\mathbf m}\) \(\newcommand{\zerovec}{\mathbf 0}\) \(\newcommand{\onevec}{\mathbf 1}\) \(\newcommand{\real}{\mathbb R}\) \(\newcommand{\twovec}[2]{\left[\begin{array}{r}#1 \\ #2 \end{array}\right]}\) \(\newcommand{\ctwovec}[2]{\left[\begin{array}{c}#1 \\ #2 \end{array}\right]}\) \(\newcommand{\threevec}[3]{\left[\begin{array}{r}#1 \\ #2 \\ #3 \end{array}\right]}\) \(\newcommand{\cthreevec}[3]{\left[\begin{array}{c}#1 \\ #2 \\ #3 \end{array}\right]}\) \(\newcommand{\fourvec}[4]{\left[\begin{array}{r}#1 \\ #2 \\ #3 \\ #4 \end{array}\right]}\) \(\newcommand{\cfourvec}[4]{\left[\begin{array}{c}#1 \\ #2 \\ #3 \\ #4 \end{array}\right]}\) \(\newcommand{\fivevec}[5]{\left[\begin{array}{r}#1 \\ #2 \\ #3 \\ #4 \\ #5 \\ \end{array}\right]}\) \(\newcommand{\cfivevec}[5]{\left[\begin{array}{c}#1 \\ #2 \\ #3 \\ #4 \\ #5 \\ \end{array}\right]}\) \(\newcommand{\mattwo}[4]{\left[\begin{array}{rr}#1 \amp #2 \\ #3 \amp #4 \\ \end{array}\right]}\) \(\newcommand{\laspan}[1]{\text{Span}\{#1\}}\) \(\newcommand{\bcal}{\cal B}\) \(\newcommand{\ccal}{\cal C}\) \(\newcommand{\scal}{\cal S}\) \(\newcommand{\wcal}{\cal W}\) \(\newcommand{\ecal}{\cal E}\) \(\newcommand{\coords}[2]{\left\{#1\right\}_{#2}}\) \(\newcommand{\gray}[1]{\color{gray}{#1}}\) \(\newcommand{\lgray}[1]{\color{lightgray}{#1}}\) \(\newcommand{\rank}{\operatorname{rank}}\) \(\newcommand{\row}{\text{Row}}\) \(\newcommand{\col}{\text{Col}}\) \(\renewcommand{\row}{\text{Row}}\) \(\newcommand{\nul}{\text{Nul}}\) \(\newcommand{\var}{\text{Var}}\) \(\newcommand{\corr}{\text{corr}}\) \(\newcommand{\len}[1]{\left|#1\right|}\) \(\newcommand{\bbar}{\overline{\bvec}}\) \(\newcommand{\bhat}{\widehat{\bvec}}\) \(\newcommand{\bperp}{\bvec^\perp}\) \(\newcommand{\xhat}{\widehat{\xvec}}\) \(\newcommand{\vhat}{\widehat{\vvec}}\) \(\newcommand{\uhat}{\widehat{\uvec}}\) \(\newcommand{\what}{\widehat{\wvec}}\) \(\newcommand{\Sighat}{\widehat{\Sigma}}\) \(\newcommand{\lt}{<}\) \(\newcommand{\gt}{>}\) \(\newcommand{\amp}{&}\) \(\definecolor{fillinmathshade}{gray}{0.9}\)Skills to Develop
- To recognize carbohydrates and classify them as mono-, di-, or polysaccharides.
All carbohydrates consist of carbon, hydrogen, and oxygen atoms and are polyhydroxy aldehydes or ketones or are compounds that can be broken down to form such compounds. Examples of carbohydrates include starch, fiber, the sweet-tasting compounds called sugars, and structural materials such as cellulose. The term carbohydrate had its origin in a misinterpretation of the molecular formulas of many of these substances. For example, because its formula is C6H12O6, glucose was once thought to be a “carbon hydrate” with the structure C6·6H2O.
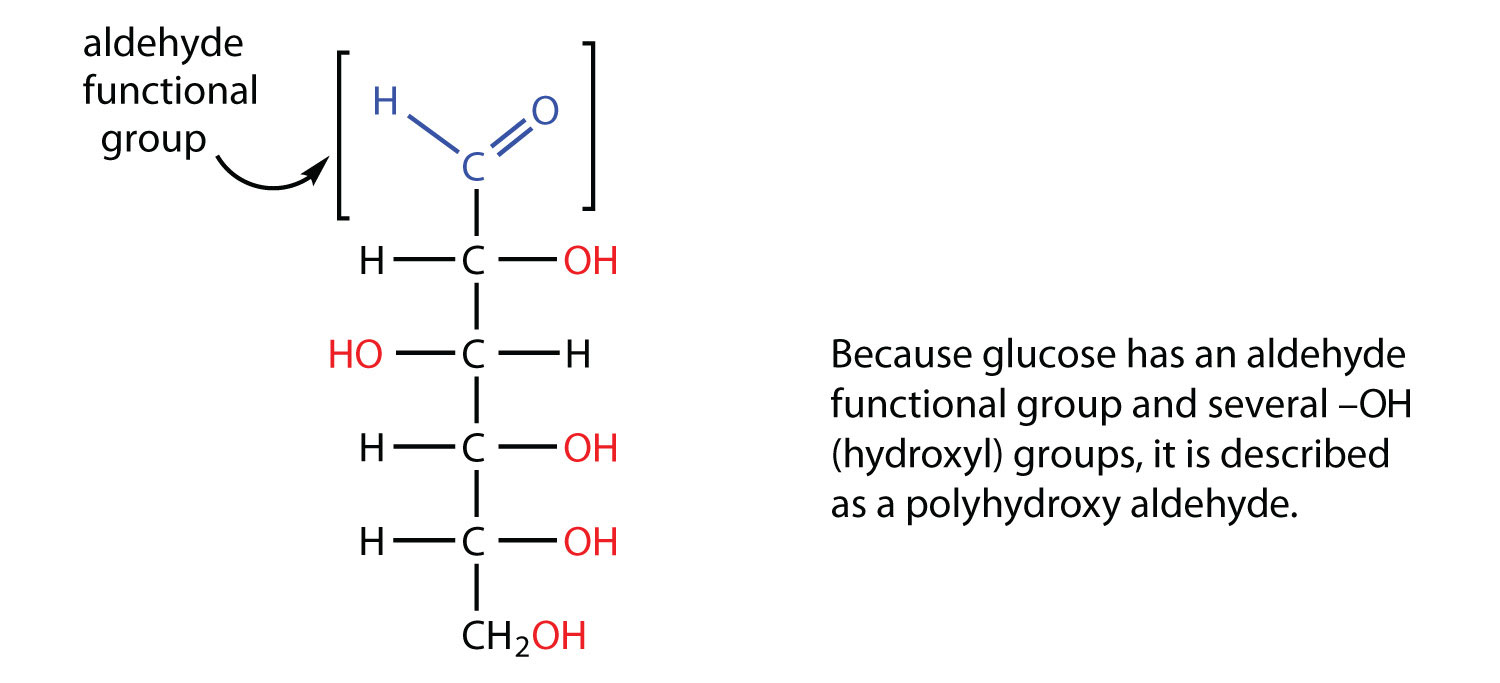
Example
Which compounds would be classified as carbohydrates?
SOLUTION
- This is a carbohydrate because the molecule contains an aldehyde functional group with OH groups on the other two carbon atoms.
- This is not a carbohydrate because the molecule does not contain an aldehyde or a ketone functional group.
- This is a carbohydrate because the molecule contains a ketone functional group with OH groups on the other two carbon atoms.
- This is not a carbohydrate; although it has a ketone functional group, one of the other carbons atoms does not have an OH group attached.
Exercise
Which compounds would be classified as carbohydrates?
Green plants are capable of synthesizing glucose (C6H12O6) from carbon dioxide (CO2) and water (H2O) by using solar energy in the process known as photosynthesis:
6 CO2(g) + 6 H2O(l) + 686 kcal --> C6H12O6(s) + 6 O2(g)
(The 686 kcal come from solar energy.) Plants can use the glucose for energy or convert it to larger carbohydrates, such as starch or cellulose. Starch provides energy for later use, perhaps as nourishment for a plant’s seeds, while cellulose is the structural material of plants. We can gather and eat the parts of a plant that store energy—seeds, roots, tubers, and fruits—and use some of that energy ourselves. Carbohydrates are also needed for the synthesis of nucleic acids and many proteins and lipids.
Animals, including humans, cannot synthesize carbohydrates from carbon dioxide and water and are therefore dependent on the plant kingdom to provide these vital compounds. We use carbohydrates not only for food (about 60%–65% by mass of the average diet) but also for clothing (cotton, linen, rayon), shelter (wood), fuel (wood), and paper (wood).
The simplest carbohydrates—those that cannot be hydrolyzed to produce even smaller carbohydrates—are called monosaccharides. Two or more monosaccharides can link together to form chains that contain from two to several hundred or thousand monosaccharide units. Prefixes are used to indicate the number of such units in the chains. Disaccharide molecules have two monosaccharide units, trisaccharide molecules have three units, and so on. Chains with many monosaccharide units joined together are called polysaccharides. All these so-called higher saccharides can be hydrolyzed back to their constituent monosaccharides.
Compounds that cannot be hydrolyzed will not react with water to form two or more smaller compounds.
Summary
Carbohydrates are an important group of biological molecules that includes sugars and starches. Photosynthesis is the process by which plants use energy from sunlight to synthesize carbohydrates. A monosaccharide is the simplest carbohydrate and cannot be hydrolyzed to produce a smaller carbohydrate molecule. Disaccharides contain two monosaccharide units, and polysaccharides contain many monosaccharide units.
Concept Review Exercises
-
Why is photosynthesis important?
-
Identify the differences among monosaccharides, disaccharides, and polysaccharides.
Answers
- Photosynthesis is the process by which solar energy is used to reduce carbon dioxide to carbohydrates, which are needed for energy by plants and other living organisms that eat plants.
- A monosaccharide is the simplest carbohydrate and cannot be hydrolyzed to produce a smaller carbohydrate; a disaccharide is composed of two monosaccharide units; and a polysaccharide contains many saccharide units.
Monosaccharides
Skills to Develop
- Classify monosaccharides as aldoses or ketoses and as trioses, tetroses, pentoses, or hexoses.
- Distinguish between a D sugar and an L sugar.
The naturally occurring monosaccharides contain three to seven carbon atoms per molecule. Monosaccharides of specific sizes may be indicated by names composed of a stem denoting the number of carbon atoms and the suffix -ose. For example, the terms triose, tetrose, pentose, and hexose signify monosaccharides with, respectively, three, four, five, and six carbon atoms. Monosaccharides are also classified as aldoses or ketoses. Those monosaccharides that contain an aldehyde functional group are called aldoses; those containing a ketone functional group on the second carbon atom are ketoses. Combining these classification systems gives general names that indicate both the type of carbonyl group and the number of carbon atoms in a molecule. Thus, monosaccharides are described as aldotetroses, aldopentoses, ketopentoses, ketoheptoses, and so forth. Glucose and fructose are specific examples of an aldohexose and a ketohexose, respectively.
Example
Draw an example of each type of compound.
- a ketopentose
- an aldotetrose
SOLUTION
-
The structure must have five carbon atoms with the second carbon atom being a carbonyl group and the other four carbon atoms each having an OH group attached. Several structures are possible, but one example is shown.
-
The structure must have four carbon atoms with the first carbon atom part of the aldehyde functional group. The other three carbon atoms each have an OH group attached. Several structures are possible, but one example is shown.
Exercise
Draw an example of each type of compound.
- an aldohexose
- a ketotetrose
The simplest sugars are the trioses. The possible trioses are shown in part (a) of Figure ; glyceraldehyde is an aldotriose, while dihydroxyacetone is a ketotriose. Notice that two structures are shown for glyceraldehyde. These structures are stereoisomers, and hence are isomers having the same structural formula but differing in the arrangement of atoms or groups of atoms in three-dimensional space. If you make models of the two stereoisomers of glyceraldehyde, you will find that you cannot place one model on top of the other and have each functional group point in the same direction. However, if you place one of the models in front of a mirror, the image in the mirror will be identical to the second stereoisomer in part (b) of Figure . Molecules that are nonsuperimposable (nonidentical) mirror images of each other are a type of stereoisomer called enantiomers (Greek enantios, meaning “opposite”).
Note
These are another type of stereoisomers than the Cis-trans (geometric) isomers previously discussed.
Figure
: Structures of the Trioses. (a) D- and L-glyceraldehyde are mirror images of each other and represent a pair of enantiomers. (b) A ball-and-stick model of D-glyceraldehyde is reflected in a mirror. Note that the reflection has the same structure as L-glyceraldehyde.
A key characteristic of enantiomers is that they have a carbon atom to which four different groups are attached. Note, for example, the four different groups attached to the central carbon atom of glyceraldehyde (part (a) of Figure ). A carbon atom that has four different groups attached is a chiral carbon. If a molecule contains one or more chiral carbons, it is likely to exist as two or more stereoisomers. Dihydroxyacetone does not contain a chiral carbon and thus does not exist as a pair of stereoisomers. Glyceraldehyde, however, has a chiral carbon and exists as a pair of enantiomers. Except for the direction in which each enantiomer rotates plane-polarized light, these two molecules have identical physical properties. One enantiomer has a specific rotation of +8.7°, while the other has a specific rotation of −8.7°.
H. Emil Fischer, a German chemist, developed the convention commonly used for writing two-dimensional representations of the monosaccharides, such as those in part (a) of Figure . In these structural formulas, the aldehyde group is written at the top, and the hydrogen atoms and OH groups that are attached to each chiral carbon are written to the right or left. (If the monosaccharide is a ketose, the ketone functional group is the second carbon atom.) Vertical lines represent bonds pointing away from you, while horizontal lines represent bonds coming toward you. The formulas of chiral molecules represented in this manner are referred to as Fischer projections.
The two enantiomers of glyceraldehyde are especially important because monosaccharides with more than three carbon atoms can be considered as being derived from them. Thus, D- and L-glyceraldehyde provide reference points for designating and drawing all other monosaccharides. Sugars whose Fischer projections terminate in the same configuration as D-glyceraldehyde are designated as D sugars; those derived from L-glyceraldehyde are designated as L sugars.
By convention, the penultimate (next-to-last) carbon atom has been chosen as the carbon atom that determines if a sugar is D or L. It is the chiral carbon farthest from the aldehyde or ketone functional group.
Looking Closer: Polarized Light
A beam of ordinary light can be pictured as a bundle of waves; some move up and down, some sideways, and others at all other conceivable angles. When a beam of light has been polarized, however, the waves in the bundle all vibrate in a single plane. Light altered in this way is called plane-polarized light. Much of what chemists know about stereoisomers comes from studying the effects they have on plane-polarized light. In this illustration, the light on the left is not polarized, while that on the right is polarized.
Sunlight, in general, is not polarized; light from an ordinary light bulb or an ordinary flashlight is not polarized. One way to polarize ordinary light is to pass it through Polaroid sheets, special plastic sheets containing carefully oriented organic compounds that permit only light vibrating in a single plane to pass through. To the eye, polarized light doesn’t “look” any different from nonpolarized light. We can detect polarized light, however, by using a second sheet of polarizing material, as shown here.
In the photo on the left, two Polaroid sheets are aligned in the same direction; plane-polarized light from the first Polaroid sheet can pass through the second sheet. In the photo on the right, the top Polaroid sheet has been rotated 90° and now blocks the plane-polarized light that comes through the first Polaroid sheet.
Certain substances act on polarized light by rotating the plane of vibration. Such substances are said to be optically active. The extent of optical activity is measured by a polarimeter, an instrument that contains two polarizing lenses separated by a sample tube, as shown in the accompanying figure. With the sample tube empty, maximum light reaches the observer’s eye when the two lenses are aligned so that both pass light vibrating in the same plane. When an optically active substance is placed in the sample tube, that substance rotates the plane of polarization of the light passing through it, so that the polarized light emerging from the sample tube is vibrating in a different direction than when it entered the tube. To see the maximum amount of light when the sample is in place, the observer must rotate one lens to accommodate the change in the plane of polarization.
Figure
: Diagram of a Polarimeter
Some optically active substances rotate the plane of polarized light to the right (clockwise) from the observer’s point of view. These compounds are said to be dextrorotatory; substances that rotate light to the left (counterclockwise) are levorotatory. To denote the direction of rotation, a positive sign (+) is given to dextrorotatory substances, and a negative sign (−) is given to levorotatory substances.
Summary
Monosaccharides can be classified by the number of carbon atoms in the structure and/or the type of carbonyl group they contain (aldose or ketose). Most monosaccharides contain at least one chiral carbon and can form stereoisomers. Enantiomers are a specific type of stereoisomers that are mirror images of each other.
Answers
- A chiral carbon is a carbon atom with four different groups attached to it.
- Enantiomers are mirror images of each other; they differ in the arrangements of atoms around a chiral carbon.
Exercises
-
Identify each sugar as an aldose or a ketose and then as a triose, tetrose, pentose, or hexose.
-
D-glucose
-
L-ribulose
-
D-glyceraldehyde
-
-
Identify each sugar as an aldose or a ketose and then as a triose, tetrose, pentose, or hexose.
-
dihydroxyacetone
-
D-ribose
-
D-galactose
-
-
Identify each sugar as an aldose or a ketose and then as a D sugar or an L sugar.
-
-
Identify each sugar as an aldose or a ketose and then as a D sugar or an L sugar.
-
Disaccharides
Disaccharides are made by the combination of two mono units joined together in a specific way. The two mono units can be the same or different from each other. In the reaction to join the two, one water molecule is lost to yield the disaccharide. For example, sucrose (table sugar) consists of a glucose and fructose molecules both with the formula C6H12O6. When joined together, the combined formulas become C12H22O11, which is two hydrogen and one oxygen atom less than the original two parts contained. This reaction can then be reversed in aqueous solution when an acid is present to return to the two mono units, where the reaction can be accelerated with the presence of an acid. This is, of course, exactly the conditions that are present in our stomach when we consume table sugar.
Sucrose
In sucrose, the glucose and fructose units are joined by an acetal oxygen bridge in the alpha orientation. The structure is easy to recognize because it contains the six member ring of glucose and the five member ring of fructose. The Figure below shows the structure of sucrose as it appears in solution.
Lactose
Lactose or milk sugar occurs in the milk of mammals - 4-6% in cow's milk and 5-8% in human milk. It is also a by product in the the manufacture of cheese. Lactose is made from galactose and glucose units. The galactose and glucose units are joined by an acetal oxygen bridge in the beta orientation. To recognize galactose look for the upward projection of the -OH on carbon # 4. See details on the galactose page towards the bottom.
Lactose intolerance
Lactose intolerance is the inability to digest significant amounts of lactose, the predominant sugar of milk. This inability results from a shortage of the enzyme lactase, which is normally produced by the cells that line the small intestine. Lactase breaks down the lactose, milk sugar, into glucose and galactose that can then be absorbed into the bloodstream. When there is not enough lactase to digest the amount of lactose consumed, produce some uncomfortable symptoms. Some adults have low levels of lactase. This leads to lactose intolerance. The ingested lactose is not absorbed in the small intestine, but instead is fermented by bacteria in the large intestine, producing uncomfortable volumes of carbon dioxide gas. While not all persons deficient in lactase have symptoms, those who do are considered to be lactose intolerant.
Common symptoms include nausea, cramps, bloating, gas, and diarrhea, which begin about 30 minutes to 2 hours after eating or drinking foods containing lactose. The severity of symptoms varies depending on the amount of lactose each individual can tolerate.
Fortunately, lactose intolerance is relatively easy to treat by controlling the diet. No cure or treatment exists to improve the body's ability to produce lactase. Young children with lactase deficiency should not eat any foods containing lactose. Most older children and adults need not avoid lactose completely, but individuals differ in the amounts and types of foods they can handle. Dietary control of lactose intolerance depends on each person's learning through trial and error how much lactose he or she can handle.
Acetal Functional Group
Carbon # 1 (red on left) is called the anomeric carbon and is the center of an acetal functional group. A carbon that has two ether oxygens attached is an acetal. The Beta position is defined as the ether oxygen being on the same side of the ring as the C # 6. In the chair structure this results in a horizontal or up projection. This is the same definition as the -OH in a hemiacetal.
Compare Lactose and Maltose Acetals
The position of the oxygen in the acetal on the anomeric carbon (#1) is an important distinction for disaccharide chemistry. Lactose has a beta acetal. The Beta position is defined as the oxygen in the acetal being on the same side of the ring as the C # 6. In the chair structure this results in a horizontal projection. Maltose has an alpha acetal. The Alpha position is defined as the oxygen in the acetal being on the opposite side of the ring as the C # 6. In the chair structure this results in a downward projection. The alpha and beta acetal label is not applied to any other carbon - only the anomeric carbon of the left monosaccharide, in this case # 1 (red).
Recognize Galactose and Glucose
To further identify lactose and maltose, identify the presence of galactose in lactose in the left most structure by the upward -OH on the carbon # 4. Identify glucose in maltose in the left most structure by the horizontal -OH on the carbon # 4.
Outlide Links
- Adapted from: Lactose Intolerance.
Contributors
- Charles Ophardt, Professor Emeritus, Elmhurst College; Virtual Chembook
Polysaccharides
As the name implies, polysaccharides are large high-molecular weight molecules constructed by joining monosaccharide units together by glycosidic bonds. They are sometimes called glycans. The most important compounds in this class, cellulose, starch and glycogen are all polymers of glucose. This is easily demonstrated by acid-catalyzed hydrolysis to the monosaccharide. Since partial hydrolysis of cellulose gives varying amounts of cellobiose, we conclude the glucose units in this macromolecule are joined by beta-glycoside bonds between C-1 and C-4 sites of adjacent sugars. Partial hydrolysis of starch and glycogen produces the disaccharide maltose together with low molecular weight dextrans, polysaccharides in which glucose molecules are joined by alpha-glycoside links between C-1 and C-6, as well as the alpha C-1 to C-4 links found in maltose. Polysaccharides built from other monosaccharides (e.g. mannose, galactose, xylose and arabinose) are also known, but will not be discussed here.
Over half of the total organic carbon in the earth's biosphere is in cellulose. Cotton fibers are essentially pure cellulose, and the wood of bushes and trees is about 50% cellulose. As a polymer of glucose, cellulose has the formula (C6H10O5)n where n ranges from 500 to 5,000, depending on the source of the polymer. The glucose units in cellulose are linked in a linear fashion, as shown in the drawing below. The beta-glycoside bonds permit these chains to stretch out, and this conformation is stabilized by intramolecular hydrogen bonds. A parallel orientation of adjacent chains is also favored by intermolecular hydrogen bonds. Although an individual hydrogen bond is relatively weak, many such bonds acting together can impart great stability to certain conformations of large molecules. Most animals cannot digest cellulose as a food, and in the diets of humans this part of our vegetable intake functions as roughage and is eliminated largely unchanged. Some animals (the cow and termites, for example) harbor intestinal microorganisms that breakdown cellulose into monosaccharide nutrients by the use of beta-glycosidase enzymes.
Cellulose is commonly accompanied by a lower molecular weight, branched, amorphous polymer called hemicellulose. In contrast to cellulose, hemicellulose is structurally weak and is easily hydrolyzed by dilute acid or base. Also, many enzymes catalyze its hydrolysis. Hemicelluloses are composed of many D-pentose sugars, with xylose being the major component. Mannose and mannuronic acid are often present, as well as galactose and galacturonic acid.
Starch is a polymer of glucose, found in roots, rhizomes, seeds, stems, tubers and corms of plants, as microscopic granules having characteristic shapes and sizes. Most animals, including humans, depend on these plant starches for nourishment. The structure of starch is more complex than that of cellulose. The intact granules are insoluble in cold water, but grinding or swelling them in warm water causes them to burst.
The released starch consists of two fractions. About 20% is a water soluble material called amylose. Molecules of amylose are linear chains of several thousand glucose units joined by alpha C-1 to C-4 glycoside bonds. Amylose solutions are actually dispersions of hydrated helical micelles. The majority of the starch is a much higher molecular weight substance, consisting of nearly a million glucose units, and called amylopectin. Molecules of amylopectin are branched networks built from C-1 to C-4 and C-1 to C-6 glycoside links, and are essentially water insoluble. Representative structural formulas for amylose and amylopectin are shown above. The branching in this diagram is exaggerated, since on average, branches only occur every twenty five glucose units.
Hydrolysis of starch, usually by enzymatic reactions, produces a syrupy liquid consisting largely of glucose. When cornstarch is the feedstock, this product is known as corn syrup. It is widely used to soften texture, add volume, prohibit crystallization and enhance the flavor of foods. Glycogen is the glucose storage polymer used by animals. It has a structure similar to amylopectin, but is even more highly branched (about every tenth glucose unit). The degree of branching in these polysaccharides may be measured by enzymatic or chemical analysis.
Glycogen is the storage form of glucose in animals and humans which is analogous to the starch in plants. Glycogen is synthesized and stored mainly in the liver and the muscles. Structurally, glycogen is very similar to amylopectin with alpha acetal linkages, however, it has even more branching and more glucose units are present than in amylopectin. Various samples of glycogen have been measured at 1,700-600,000 units of glucose.
The structure of glycogen consists of long polymer chains of glucose units connected by an alpha acetal linkage. The graphic on the left shows a very small portion of a glycogen chain. All of the monomer units are alpha-D-glucose, and all the alpha acetal links connect C # 1 of one glucose to C # 4 of the next glucose.
The branches are formed by linking C #1 to a C #6 through an acetal linkages. In glycogen, the branches occur at intervals of 8-10 glucose units, while in amylopectin the branches are separated by 12-20 glucose units.
Contributors
Prof. Steven Farmer (Sonoma State University)
William Reusch, Professor Emeritus (Michigan State U.), Virtual Textbook of Organic Chemistry