14.1.6: Glycolysis
- Page ID
- 338388
\( \newcommand{\vecs}[1]{\overset { \scriptstyle \rightharpoonup} {\mathbf{#1}} } \)
\( \newcommand{\vecd}[1]{\overset{-\!-\!\rightharpoonup}{\vphantom{a}\smash {#1}}} \)
\( \newcommand{\id}{\mathrm{id}}\) \( \newcommand{\Span}{\mathrm{span}}\)
( \newcommand{\kernel}{\mathrm{null}\,}\) \( \newcommand{\range}{\mathrm{range}\,}\)
\( \newcommand{\RealPart}{\mathrm{Re}}\) \( \newcommand{\ImaginaryPart}{\mathrm{Im}}\)
\( \newcommand{\Argument}{\mathrm{Arg}}\) \( \newcommand{\norm}[1]{\| #1 \|}\)
\( \newcommand{\inner}[2]{\langle #1, #2 \rangle}\)
\( \newcommand{\Span}{\mathrm{span}}\)
\( \newcommand{\id}{\mathrm{id}}\)
\( \newcommand{\Span}{\mathrm{span}}\)
\( \newcommand{\kernel}{\mathrm{null}\,}\)
\( \newcommand{\range}{\mathrm{range}\,}\)
\( \newcommand{\RealPart}{\mathrm{Re}}\)
\( \newcommand{\ImaginaryPart}{\mathrm{Im}}\)
\( \newcommand{\Argument}{\mathrm{Arg}}\)
\( \newcommand{\norm}[1]{\| #1 \|}\)
\( \newcommand{\inner}[2]{\langle #1, #2 \rangle}\)
\( \newcommand{\Span}{\mathrm{span}}\) \( \newcommand{\AA}{\unicode[.8,0]{x212B}}\)
\( \newcommand{\vectorA}[1]{\vec{#1}} % arrow\)
\( \newcommand{\vectorAt}[1]{\vec{\text{#1}}} % arrow\)
\( \newcommand{\vectorB}[1]{\overset { \scriptstyle \rightharpoonup} {\mathbf{#1}} } \)
\( \newcommand{\vectorC}[1]{\textbf{#1}} \)
\( \newcommand{\vectorD}[1]{\overrightarrow{#1}} \)
\( \newcommand{\vectorDt}[1]{\overrightarrow{\text{#1}}} \)
\( \newcommand{\vectE}[1]{\overset{-\!-\!\rightharpoonup}{\vphantom{a}\smash{\mathbf {#1}}}} \)
\( \newcommand{\vecs}[1]{\overset { \scriptstyle \rightharpoonup} {\mathbf{#1}} } \)
\( \newcommand{\vecd}[1]{\overset{-\!-\!\rightharpoonup}{\vphantom{a}\smash {#1}}} \)
\(\newcommand{\avec}{\mathbf a}\) \(\newcommand{\bvec}{\mathbf b}\) \(\newcommand{\cvec}{\mathbf c}\) \(\newcommand{\dvec}{\mathbf d}\) \(\newcommand{\dtil}{\widetilde{\mathbf d}}\) \(\newcommand{\evec}{\mathbf e}\) \(\newcommand{\fvec}{\mathbf f}\) \(\newcommand{\nvec}{\mathbf n}\) \(\newcommand{\pvec}{\mathbf p}\) \(\newcommand{\qvec}{\mathbf q}\) \(\newcommand{\svec}{\mathbf s}\) \(\newcommand{\tvec}{\mathbf t}\) \(\newcommand{\uvec}{\mathbf u}\) \(\newcommand{\vvec}{\mathbf v}\) \(\newcommand{\wvec}{\mathbf w}\) \(\newcommand{\xvec}{\mathbf x}\) \(\newcommand{\yvec}{\mathbf y}\) \(\newcommand{\zvec}{\mathbf z}\) \(\newcommand{\rvec}{\mathbf r}\) \(\newcommand{\mvec}{\mathbf m}\) \(\newcommand{\zerovec}{\mathbf 0}\) \(\newcommand{\onevec}{\mathbf 1}\) \(\newcommand{\real}{\mathbb R}\) \(\newcommand{\twovec}[2]{\left[\begin{array}{r}#1 \\ #2 \end{array}\right]}\) \(\newcommand{\ctwovec}[2]{\left[\begin{array}{c}#1 \\ #2 \end{array}\right]}\) \(\newcommand{\threevec}[3]{\left[\begin{array}{r}#1 \\ #2 \\ #3 \end{array}\right]}\) \(\newcommand{\cthreevec}[3]{\left[\begin{array}{c}#1 \\ #2 \\ #3 \end{array}\right]}\) \(\newcommand{\fourvec}[4]{\left[\begin{array}{r}#1 \\ #2 \\ #3 \\ #4 \end{array}\right]}\) \(\newcommand{\cfourvec}[4]{\left[\begin{array}{c}#1 \\ #2 \\ #3 \\ #4 \end{array}\right]}\) \(\newcommand{\fivevec}[5]{\left[\begin{array}{r}#1 \\ #2 \\ #3 \\ #4 \\ #5 \\ \end{array}\right]}\) \(\newcommand{\cfivevec}[5]{\left[\begin{array}{c}#1 \\ #2 \\ #3 \\ #4 \\ #5 \\ \end{array}\right]}\) \(\newcommand{\mattwo}[4]{\left[\begin{array}{rr}#1 \amp #2 \\ #3 \amp #4 \\ \end{array}\right]}\) \(\newcommand{\laspan}[1]{\text{Span}\{#1\}}\) \(\newcommand{\bcal}{\cal B}\) \(\newcommand{\ccal}{\cal C}\) \(\newcommand{\scal}{\cal S}\) \(\newcommand{\wcal}{\cal W}\) \(\newcommand{\ecal}{\cal E}\) \(\newcommand{\coords}[2]{\left\{#1\right\}_{#2}}\) \(\newcommand{\gray}[1]{\color{gray}{#1}}\) \(\newcommand{\lgray}[1]{\color{lightgray}{#1}}\) \(\newcommand{\rank}{\operatorname{rank}}\) \(\newcommand{\row}{\text{Row}}\) \(\newcommand{\col}{\text{Col}}\) \(\renewcommand{\row}{\text{Row}}\) \(\newcommand{\nul}{\text{Nul}}\) \(\newcommand{\var}{\text{Var}}\) \(\newcommand{\corr}{\text{corr}}\) \(\newcommand{\len}[1]{\left|#1\right|}\) \(\newcommand{\bbar}{\overline{\bvec}}\) \(\newcommand{\bhat}{\widehat{\bvec}}\) \(\newcommand{\bperp}{\bvec^\perp}\) \(\newcommand{\xhat}{\widehat{\xvec}}\) \(\newcommand{\vhat}{\widehat{\vvec}}\) \(\newcommand{\uhat}{\widehat{\uvec}}\) \(\newcommand{\what}{\widehat{\wvec}}\) \(\newcommand{\Sighat}{\widehat{\Sigma}}\) \(\newcommand{\lt}{<}\) \(\newcommand{\gt}{>}\) \(\newcommand{\amp}{&}\) \(\definecolor{fillinmathshade}{gray}{0.9}\)Glycolysis, which literally means “breakdown of sugar," is a catabolic process in which six-carbon sugars (hexoses) are oxidized and broken down into pyruvate molecules. The corresponding anabolic pathway by which glucose is synthesized is termed gluconeogenesis. Both glycolysis and gluconeogenesis are not major oxidative/reductive processes by themselves, with one step in each one involving loss/gain of electrons, but the product of glycolysis, pyruvate, can be completely oxidized to carbon dioxide. Indeed, without production of pyruvate from glucose in glycolysis, a major energy source for the cell is not available. By contrast, gluconeogenesis can synthesize glucose reductively from very simple materials, such as pyruvate and acetyl-CoA/ glyoxylate (at least in plants). For these reasons we include these pathways in the red/ox collection.
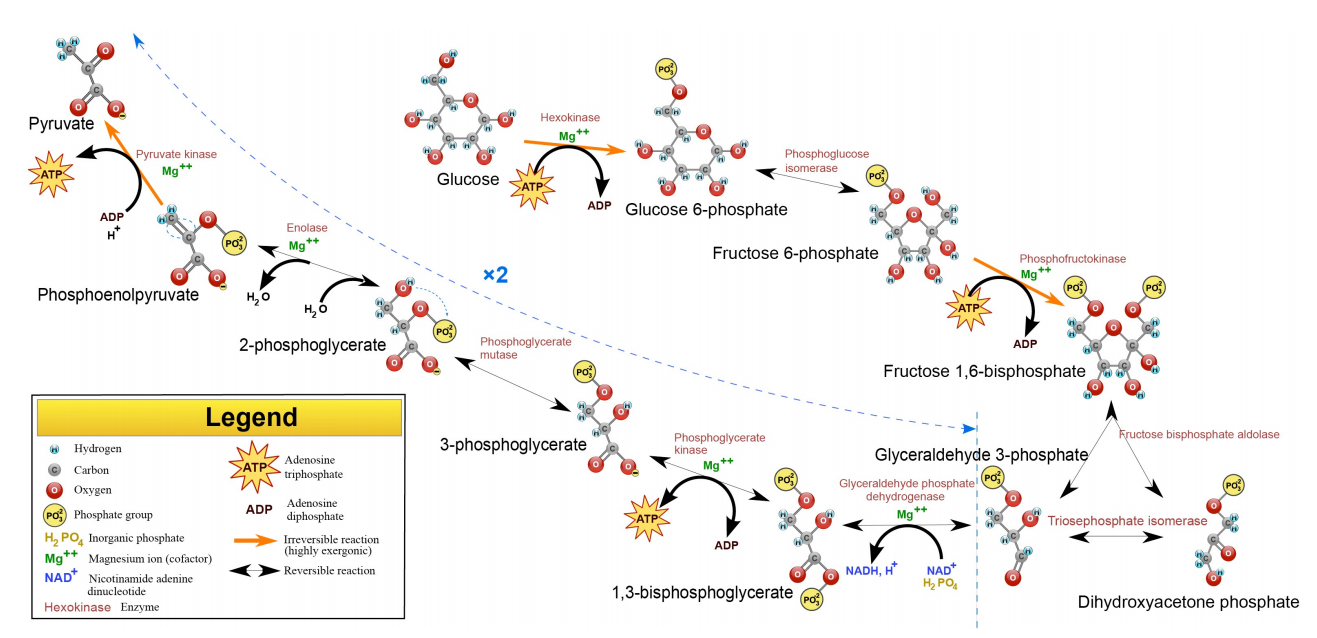
Glucose is the most abundant hexose in nature and is the one people typically associate with glycolysis, but fructose (in the form of fructose-6-phosphate) is metabolized in the cell and galactose can easily be converted into glucose for catabolism in the pathway as well. The end metabolic products of the pathway are two molecules of ATP, two molecules of NADH and two molecules of pyruvate, which, in turn, can be oxidized further in citric acid cycle.
Intermediates
Glucose and fructose are the sugar ‘funnels’ serving as entry points to the glycolytic pathway. Other sugars must be converted to either of these forms to be directly metabolized. Some pathways, including the Calvin Cycle and the Pentose Phosphate Pathway (PPP, see below) contain intermediates in common with glycolysis, so in that sense, almost any cellular sugar can be metabolized here. Intermediates of glycolysis that are common to other pathways include glucose-6-phosphate (PPP, glycogen metabolism), F6P (PPP), G3P (Calvin, PPP), DHAP (PPP, glycerol metabolism, Calvin), 3PG (Calvin, PPP), PEP (C4 plant metabolism, Calvin), and pyruvate (fermentation, acetyl-CoA genesis, amino acid metabolism).
Reactions
The pathway of glycolysis begins with two inputs of energy. First, glucose gets a phosphate from ATP to make glucose-6-phosphate (G6P) and later fructose-6-phosphate (F6P) gets another phosphate from ATP to make fructose-1,6-bisphosphate (F1,6BP). With the pump thus primed, the pathway proceeds first to split the F1,6BP into two 3-carbon intermediates. Later the only oxidation step in the entire pathway occurs. In that reaction, glyceraldehyde-3-phosphate (G3P) is oxidized and a phosphate is added, creating 1,3-bisphosphoglycerate (1,3 BPG).
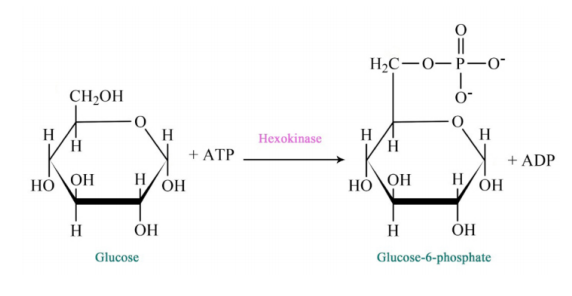
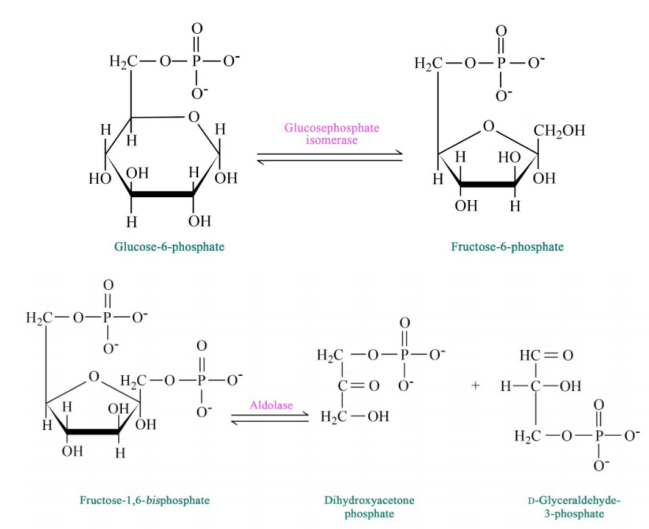
The addition of the phosphate sometimes conceals the oxidation that occurred. G3P was an aldehyde. 1,3 BGP is an acid esterified to a phosphate. The two phosphates in the tiny 1,3BPG molecule repel each other and give the molecule high energy. It uses this energy to phosphorylate ADP to make ATP.
Since there are two 1,3 BPGs produced for every glucose, the two ATP produced replenish the two ATPs used to start the cycle.
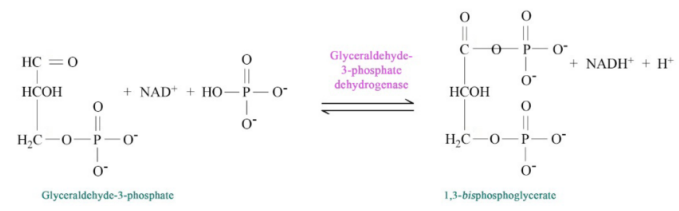
The synthesis of ATP directly from a metabolic reaction is known as substrate level phosphorylation, though it is not a significant source of ATP. Glycolysis has two reactions during which substrate-level phosphorylation occurs.
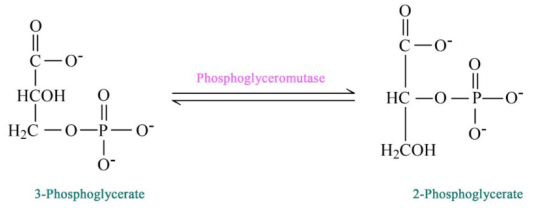
The transfer of phosphate from 1,3BPG to ATP creates 3-phosphoglycerate (3-PG). Conversion of 3-PG to 2-PG occurs by an important mechanism. An intermediate in the reaction (catalyzed by phosphogly cerate mutase) is 2,3 BPG. This intermediate, which is stable, is released with low frequency by the enzyme instead of being converted to 2-PG. 2,3BPG is important because it binds to hemoglobin and stimulates release of oxygen. Thus, cells which are metabolizing glucose rapidly release more 2,3BPG and, as a result, stimulate release of more oxygen, supporting their needs.
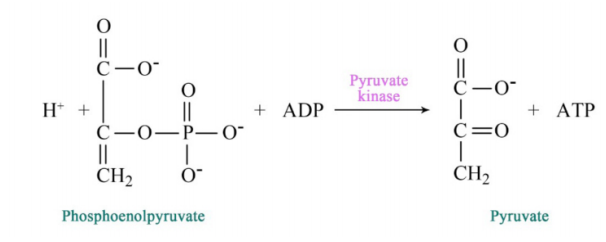
2-PG is converted to phosphoenolpyyruvate (PEP) by removal of water, creating a very high energy intermediate. Conversion of PEP to pyruvate is the second substrate level phosphorylation of glycolysis, creating ATP. There is almost enough energy in PEP to stimulate production of a second ATP, but it is not used. Consequently, the energy is lost as heat. If you wonder why you get hot when you exercise, the reaction that converts PEP to pyruvate is a prime culprit.

Enzymes/Control
Control of glycolysis is unusual for a metabolic pathway, in that regulation occurs at three enzymatic points:
\[\underbrace{ \ce{Glucose <=> G6P}}_{\text{hexokinase} }\]
\[\underbrace{ \ce{F6P <=> F1,6BP}}_{\text{phosphofructokinase (PFK)} }\]
and
\[\underbrace{ \ce{PEP <=> pyruvate}}_{\text{pyruvate kinase} }.\]
Glycolysis is regulated in a reciprocal fashion compared to its corresponding anabolic pathway, gluconeogenesis. Reciprocal regulation occurs when the same molecule or treatment (phosphorylation, for example) has opposite effects on catabolic and anabolic pathways. Reciprocal regulation is important when anabolic and corresponding catabolic pathways are occurring in the same cellular location.
As an example, consider regulation of PFK. It is activated by several molecules, most importantly fructose-2,6- bisphosphate (F2,6BP). This molecule has an inhibitory effect on the corresponding gluconeogenesis enzyme, fructose-1,6-bisphosphatase (F1,6BPase).
You might wonder why pyruvate kinase, the last enzyme in the pathway, is regulated. The answer is simple. Pyruvate kinase catalyzes the most energetically rich reaction of glycolysis. The reaction is favored so strongly in the forward direction that cells must do a ‘two-step’ around it in the reverse direction when making glucose. In other words, it takes two enzymes, two reactions, and two triphosphates to go from pyruvate back to PEP in gluconeogenesis. When cells are needing to make glucose, they can’t be sidetracked by having the PEP they have made in gluconeogenesis be converted directly back to pyruvate by pyruvate kinase. Consequently, pyruvate kinase is inhibited during gluconeogenesis, lest a “futile cycle" occur.
Another interesting control mechanism called feedforward activation involves pyruvate kinase. Pyruvate kinase is activated allosterically by F1,6BP. This molecule is a product of the PFK reaction and a substrate for the aldolase reaction. It should be noted that the aldolase reaction is energetically unfavorable (high +\(\Delta\)G°’), thus allowing F1,6BP to accumulate. When this happens, some of the excess F1,6BP activates pyruvate kinase, which jump-starts the conversion of PEP to pyruvate. The resulting drop in PEP levels has the effect of “pulling" on the reactions preceding pyruvate kinase. As a consequence, the concentrations of G3P and DHAP fall, helping to move the aldolase reaction forward.
Pyruvate Metabolism
As noted, pyruvate produced in glycolysis can be oxidized to acetyl-CoA, which is itself oxidized in the citric acid cycle to carbon dioxide. That is not the only metabolic fate of pyruvate, though.
Pyruvate is a “starting" point for gluconeogenesis, being converted to oxaloacetate in the mitochondrion in the first step. Pyruvate in animals can also be reduced to lactate when oxygen is limiting. This reaction, which requires NADH produces \(\text{NAD}^+\) and is critical for generating the latter molecule to keep the glyceraldehyde-3-phosphate dehydrogenase reaction of glycolysis going when there is no oxygen.
Oxygen is necessary for the electron transport system to operate and this, in turn, is what oxidizes NADH to \(\text{NAD}^+\). In the absence of oxygen, thus, an alternative means of making \(\text{NAD}^+\) is necessary, or else glycolysis will halt. Bacteria and yeast have NADH requiring reactions that regenerate \(\text{NAD}^+\) while producing ethanol from pyruvate under anaerobic conditions, instead of lactic acid. Thus, fermentation of pyruvate is necessary to keep glycolysis operating when oxygen is limiting. It is also for these reasons that brewing of beer (using yeast) involves depletion of oxygen and muscles low in oxygen produce lactic acid (animals).
Pyruvate is a precursor of alanine which can be easily synthesized by transfer of a nitrogen from an amine donor, such as glutamic acid. Pyruvate can also be converted into oxaloacetate by carboxylation in the process of gluconeogenesis (see Figure 6.3.8).
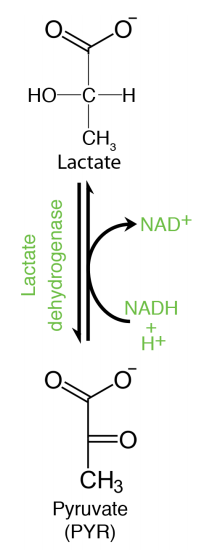
The enzymes involved in pyruvate metabolism include pyruvate dehydrogenase (makes acetyl-CoA), lactate dehydrogenase (makes lactate), transaminases (make alanine), an
pyruvate carboxylase (makes oxaloacetate).