1.1: Matter and energy
- Page ID
- 371338
\( \newcommand{\vecs}[1]{\overset { \scriptstyle \rightharpoonup} {\mathbf{#1}} } \)
\( \newcommand{\vecd}[1]{\overset{-\!-\!\rightharpoonup}{\vphantom{a}\smash {#1}}} \)
\( \newcommand{\id}{\mathrm{id}}\) \( \newcommand{\Span}{\mathrm{span}}\)
( \newcommand{\kernel}{\mathrm{null}\,}\) \( \newcommand{\range}{\mathrm{range}\,}\)
\( \newcommand{\RealPart}{\mathrm{Re}}\) \( \newcommand{\ImaginaryPart}{\mathrm{Im}}\)
\( \newcommand{\Argument}{\mathrm{Arg}}\) \( \newcommand{\norm}[1]{\| #1 \|}\)
\( \newcommand{\inner}[2]{\langle #1, #2 \rangle}\)
\( \newcommand{\Span}{\mathrm{span}}\)
\( \newcommand{\id}{\mathrm{id}}\)
\( \newcommand{\Span}{\mathrm{span}}\)
\( \newcommand{\kernel}{\mathrm{null}\,}\)
\( \newcommand{\range}{\mathrm{range}\,}\)
\( \newcommand{\RealPart}{\mathrm{Re}}\)
\( \newcommand{\ImaginaryPart}{\mathrm{Im}}\)
\( \newcommand{\Argument}{\mathrm{Arg}}\)
\( \newcommand{\norm}[1]{\| #1 \|}\)
\( \newcommand{\inner}[2]{\langle #1, #2 \rangle}\)
\( \newcommand{\Span}{\mathrm{span}}\) \( \newcommand{\AA}{\unicode[.8,0]{x212B}}\)
\( \newcommand{\vectorA}[1]{\vec{#1}} % arrow\)
\( \newcommand{\vectorAt}[1]{\vec{\text{#1}}} % arrow\)
\( \newcommand{\vectorB}[1]{\overset { \scriptstyle \rightharpoonup} {\mathbf{#1}} } \)
\( \newcommand{\vectorC}[1]{\textbf{#1}} \)
\( \newcommand{\vectorD}[1]{\overrightarrow{#1}} \)
\( \newcommand{\vectorDt}[1]{\overrightarrow{\text{#1}}} \)
\( \newcommand{\vectE}[1]{\overset{-\!-\!\rightharpoonup}{\vphantom{a}\smash{\mathbf {#1}}}} \)
\( \newcommand{\vecs}[1]{\overset { \scriptstyle \rightharpoonup} {\mathbf{#1}} } \)
\( \newcommand{\vecd}[1]{\overset{-\!-\!\rightharpoonup}{\vphantom{a}\smash {#1}}} \)
\(\newcommand{\avec}{\mathbf a}\) \(\newcommand{\bvec}{\mathbf b}\) \(\newcommand{\cvec}{\mathbf c}\) \(\newcommand{\dvec}{\mathbf d}\) \(\newcommand{\dtil}{\widetilde{\mathbf d}}\) \(\newcommand{\evec}{\mathbf e}\) \(\newcommand{\fvec}{\mathbf f}\) \(\newcommand{\nvec}{\mathbf n}\) \(\newcommand{\pvec}{\mathbf p}\) \(\newcommand{\qvec}{\mathbf q}\) \(\newcommand{\svec}{\mathbf s}\) \(\newcommand{\tvec}{\mathbf t}\) \(\newcommand{\uvec}{\mathbf u}\) \(\newcommand{\vvec}{\mathbf v}\) \(\newcommand{\wvec}{\mathbf w}\) \(\newcommand{\xvec}{\mathbf x}\) \(\newcommand{\yvec}{\mathbf y}\) \(\newcommand{\zvec}{\mathbf z}\) \(\newcommand{\rvec}{\mathbf r}\) \(\newcommand{\mvec}{\mathbf m}\) \(\newcommand{\zerovec}{\mathbf 0}\) \(\newcommand{\onevec}{\mathbf 1}\) \(\newcommand{\real}{\mathbb R}\) \(\newcommand{\twovec}[2]{\left[\begin{array}{r}#1 \\ #2 \end{array}\right]}\) \(\newcommand{\ctwovec}[2]{\left[\begin{array}{c}#1 \\ #2 \end{array}\right]}\) \(\newcommand{\threevec}[3]{\left[\begin{array}{r}#1 \\ #2 \\ #3 \end{array}\right]}\) \(\newcommand{\cthreevec}[3]{\left[\begin{array}{c}#1 \\ #2 \\ #3 \end{array}\right]}\) \(\newcommand{\fourvec}[4]{\left[\begin{array}{r}#1 \\ #2 \\ #3 \\ #4 \end{array}\right]}\) \(\newcommand{\cfourvec}[4]{\left[\begin{array}{c}#1 \\ #2 \\ #3 \\ #4 \end{array}\right]}\) \(\newcommand{\fivevec}[5]{\left[\begin{array}{r}#1 \\ #2 \\ #3 \\ #4 \\ #5 \\ \end{array}\right]}\) \(\newcommand{\cfivevec}[5]{\left[\begin{array}{c}#1 \\ #2 \\ #3 \\ #4 \\ #5 \\ \end{array}\right]}\) \(\newcommand{\mattwo}[4]{\left[\begin{array}{rr}#1 \amp #2 \\ #3 \amp #4 \\ \end{array}\right]}\) \(\newcommand{\laspan}[1]{\text{Span}\{#1\}}\) \(\newcommand{\bcal}{\cal B}\) \(\newcommand{\ccal}{\cal C}\) \(\newcommand{\scal}{\cal S}\) \(\newcommand{\wcal}{\cal W}\) \(\newcommand{\ecal}{\cal E}\) \(\newcommand{\coords}[2]{\left\{#1\right\}_{#2}}\) \(\newcommand{\gray}[1]{\color{gray}{#1}}\) \(\newcommand{\lgray}[1]{\color{lightgray}{#1}}\) \(\newcommand{\rank}{\operatorname{rank}}\) \(\newcommand{\row}{\text{Row}}\) \(\newcommand{\col}{\text{Col}}\) \(\renewcommand{\row}{\text{Row}}\) \(\newcommand{\nul}{\text{Nul}}\) \(\newcommand{\var}{\text{Var}}\) \(\newcommand{\corr}{\text{corr}}\) \(\newcommand{\len}[1]{\left|#1\right|}\) \(\newcommand{\bbar}{\overline{\bvec}}\) \(\newcommand{\bhat}{\widehat{\bvec}}\) \(\newcommand{\bperp}{\bvec^\perp}\) \(\newcommand{\xhat}{\widehat{\xvec}}\) \(\newcommand{\vhat}{\widehat{\vvec}}\) \(\newcommand{\uhat}{\widehat{\uvec}}\) \(\newcommand{\what}{\widehat{\wvec}}\) \(\newcommand{\Sighat}{\widehat{\Sigma}}\) \(\newcommand{\lt}{<}\) \(\newcommand{\gt}{>}\) \(\newcommand{\amp}{&}\) \(\definecolor{fillinmathshade}{gray}{0.9}\)- Define matter, element, molecule, compound, molecule, mixture, homogeneous and heterogeneous mixtures, the law of constant composition, states of matter, i.e., solid, liquid, gas, and plasma, energy and its types kinetic and potential energy, endothermic and exothermic and exothermic processes, and the law of conservation of energy.
- Be able to write and interpret symbols of elements, molecules, and compounds.
What is the matter?
Matter is anything that has mass and occupies space.
Matter is a natural material that makes up the universe. The matter comprises tiny particles called atoms held together by forces called bonds. The matter is classified as pure if it has a constant and a non-variable composition of the type of atoms. Pure matter is either an element or a compound.
Element
An element is pure matter that is composed of only one type of atom
For example, carbon, shown in Fig. 1.1.1, is an element. Elements can not convert to simpler matter by physical or chemical methods. There are around a hundred different elements known at this time. For example, hydrogen, oxygen, carbon, nitrogen, sodium, chlorine, iron, cobalt, gold, and silver are a few elements.

Symbol of an element
Symbols represent elements, the first alphabet of their English or non-English name, written in capital letters. For example, C for carbon, O for oxygen, and H for hydrogen. Usually, another alphabet is also chosen from the element's name and written as a small letter, e.g., He for helium, Co for cobalt. Some element symbols are derived from non-English names, e.g., Fe for iron is from its Latin name Ferrum, and Au for gold is from its Latin name Aurum.
Two consecutive capital letters do not represent an element; they may combine two elements.
CO is not a symbol of an element; it is a pure matter, a combination of carbon and oxygen bonded together in a 1:1 atom ratio.
Molecule
A molecule is a group of two or more atoms held together by forces called chemical bonds. The molecule is the smallest particle of matter that can exist freely. A single atom of some elements can exist freely and is also considered a molecule. For example, He, O2, P4, and S8 are examples of elements having molecules composed of one, two, four, and eight atoms of the same element, respectively, as illustrated in Fig. 1.1.2. Although metal elements exist as a vast number of atoms bonded together by a special type of bond called metallic bonds, their symbol is that of a single atom, e.g., Fe for iron and Au for gold.

Compound
A compound is a pure matter composed of atoms of two or more different elements in a constant whole-number ratio held together by chemical bonds.
The symbol of a compound is a combination of its constituent elements with a subscript to the right of the element symbol representing the whole number ratio of the atoms of the element in the compound. For example, H2O symbolizes a compound called water composed of hydrogen (H) and oxygen (O) atoms in a 2:1 ratio. Similarly, NaCl symbolizes a table salt compound composed of sodium (Na) and chlorine (Cl) atoms in a 1:1 ratio. If the symbol of a compound also represents a molecule of the compound, it is called a molecular formula. For example, H2O is a molecule formula of water. On the other hand, table salt is another class of compound composed of a vast number of atoms of its constituent elements arranged in a specific arrangement in 3D space called a crystal lattice, as illustrated in Fig. 1.1.3. When the compound symbol does not represent a molecule, it only means the simple whole-number ratio of the constituent elements; it is called the formula unit. For example, NaCl is a chemical formula of a compound called table salt.

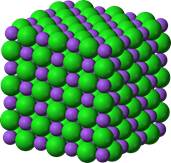
A chemical compound always contains the number of atoms of its component elements in a constant ratio, or the ratio of masses of the constituent elements is a constant in a given compound.
Pure matter, i.e., elements or compounds, has a fixed proportion of atoms of element/s independent of the source or method of their preparation. It is also called a chemical or a substance. The atoms in a compound are held together by attractive forces called chemical bonds. Constituent elements in a compound can be separated only by breaking chemical bonds and making new chemical bonds which is a chemical reaction.
Mixture
Two or more pure substances mixed such that ratio of the constituents is variable is called a mixture.
For example, table salt can be mixed with water, but the salt (NaCl) to water (H2O) can be varied to give less salty or more salty water in a mixture. A mixture in which the components are thoroughly mixed, and the composition is constant throughout a given sample, is a homogeneous mixture or a solution. For example, table salt dissolved in water is an example of a homogeneous mixture or a solution. Other homogeneous mixtures include sugar dissolved in water; the air, a mixture of nitrogen, oxygen, carbon dioxide, and other gases; and metal alloys like brass, a mix of copper and zinc metals, etc.


It is a heterogeneous mixture if the miitsnents are not thoroughly mixed and the composition varies within different regions of a given sample. For example, sulfur (S) powder mixed with iron (Fe) filling is a heterogeneous mixture shown in Fig. 1.1.4. Other examples of heterogeneous mixtures include smoke which is a mixture of air and carbon particles; smog which is a mixture of liquid water droplets suspended in air; and orange juice which is a mixture of sugar, water, fiber particles, etc. Fig. 1.1.5 illustrates the classification of the matter described above.

States of matter
Matter exists in one of the four physical states or phases, i.e., solid (s), liquid (l), gas (g), or plasma. Fig. 1.1.6 illustrates the four states of matter at the molecular level, and Fig. 1.1.7 shows examples of the four states of matter.


Solid-state
In the solid state, the particles, i.e., atoms or molecules, are very close to each other and held strongly by intermolecular forces. The particles can vibrate around their mean positions, but they cannot slide past each other. Expansion and contraction in a solid state are negligible. The solid has a fixed shape and a fixed volume.
Liquid state
In the liquid state, the particles are close enough to experience strong intermolecular interactions that usually do not let the particles cross the liquid boundary. Still, the particles can move around within the liquid. Consequently, the particles in a liquid can flow and acquire the shape of the container but have a fixed volume. Expansion and contraction are negligible in the liquid state.
Gas state
In the gas state, the particles are far apart. The intermolecular interactions are negligible in the gas phase due to the large distances between the particles. The gas molecules move in straight lines in random directions until they collide with other molecules or the walls of the container. The collisions are elastic; that is, the molecules bounce off like elastic balls, and the total kinetic energy of the system is conserved. If exposed to space, the particles keep moving into space. In other words, the particles in a gas can flow, acquire the shape of the container, and expand or contract to fill up the available space. The gases do not have a fixed shape and do not have a fixed volume.
Plasma state
In the plasma state, the particles are far apart like gases, and a portion of the negative charge of the particles, i.e., electrons, are separated from the positive charge potion, i.e., the nucleus. In other words, the atoms in the plasma state are ionized. The plasma state is not common on Earth but is the universe's most common state of matter. For example, the matter in the sun and stars is in the plasma state. Examples of the plasma state on Earth include the matter in the lightning bolts and electrical sparks.
What is energy
Energy is a quantitative property transferred to an object and recognizable as performing work or as heat or light. In simple words: energy is the ability to do work.
What we commonly encounter other than matter is energy. There are two basic types of energies, i.e., the kinetic energy of moving objects and potential energy stored by the position of an object in a force field, e.g., gravitation potential energy under the gravity force and chemical potential energy under the electrical fields in the bonds, as illustrated in Figure \(\PageIndex{8}\).

Kinetic energy
The energy of moving objects is kinetic energy. The mathematical form of kinetic energy (KE) is \(K E=\frac{1}{2} m v^{2}\), where m is the mass and v is the velocity of the moving object. Examples of kinetic energy include the energy of all moving things that we see around, like moving vehicles or a moving turbine that generates electricity. Thermal energy is also the kinetic energy of the atoms and molecules in matter.
Potential energy
Potential energy is due to the position of an object in a force field. Examples of force fields responsible for the potential energy include electric, magnetic, gravitational, and elastic forces. Examples of potential energies are electrical energy and gravitational energy. Light is potential energy due to moving electric and magnetic fields. Chemical energy is potential energy stored in chemical bonds in electrostatic potential energy.
Gravitation potential energy is due to position or height relative to the earth. The earth attracts other objects with force \( F = mg\), where F is the force, m is the object's mass, and g is the acceleration due to gravity. When an object falls, the potential energy changes to several forms of energy, including kinetic energy, work against friction from air, sound, and work done in deformations when it hits the ground.
Chemical potential energy is due to chemical bonds that hold the atoms together in a molecule or compound through electrical forces between negative charge electrons and positive charge nuclei. Bond-forming always releases energy, and bond-breaking absorbs the same energy. Each bond has different bond energy. In chemical reactions, some bonds break, and some bonds form.
A combination of kinetic and potential energy is also possible, e.g., in mechanical waves. A sound wave is a mechanical wave that combines kinetic and potential energy –kinetic because particles move and potential due to the elasticity of the material in which the deformation (sound) is propagating.
Exothermic and endothermic reactions


The balance between the energy needed to break the bonds and the energy released from forming new bonds determines whether the chemical reaction releases or absorbs energy. Chemical reactions that release energy are called exothermic reactions. For example, the combustion of methane gas in kitchen burners releases energy. A reaction that absorbs energy is called an endothermic reaction. For example, photosynthesis converts carbon dioxide and water to glucose by absorbing sunlight, as illustrated in Fig. 1.1.9.
Law of conservation of energy

The energy conservation law states that energy can transform from one form to another, but the total energy of an isolated system remains the same.
Energy can transform from one form to another through work or heat. An oscillating pendulum is an example of kinetic and gravitational potential energy periodically transforming into each other through work. Radiation energy in sunlight transfers to chemical energy during photosynthesis, heat, and work during glucose metabolism, but the total energy remains the same, as illustrated in Figure \(\PageIndex{10}\).