13.9: Carbon Dioxide and Climate Change
- Page ID
- 153872
\( \newcommand{\vecs}[1]{\overset { \scriptstyle \rightharpoonup} {\mathbf{#1}} } \)
\( \newcommand{\vecd}[1]{\overset{-\!-\!\rightharpoonup}{\vphantom{a}\smash {#1}}} \)
\( \newcommand{\id}{\mathrm{id}}\) \( \newcommand{\Span}{\mathrm{span}}\)
( \newcommand{\kernel}{\mathrm{null}\,}\) \( \newcommand{\range}{\mathrm{range}\,}\)
\( \newcommand{\RealPart}{\mathrm{Re}}\) \( \newcommand{\ImaginaryPart}{\mathrm{Im}}\)
\( \newcommand{\Argument}{\mathrm{Arg}}\) \( \newcommand{\norm}[1]{\| #1 \|}\)
\( \newcommand{\inner}[2]{\langle #1, #2 \rangle}\)
\( \newcommand{\Span}{\mathrm{span}}\)
\( \newcommand{\id}{\mathrm{id}}\)
\( \newcommand{\Span}{\mathrm{span}}\)
\( \newcommand{\kernel}{\mathrm{null}\,}\)
\( \newcommand{\range}{\mathrm{range}\,}\)
\( \newcommand{\RealPart}{\mathrm{Re}}\)
\( \newcommand{\ImaginaryPart}{\mathrm{Im}}\)
\( \newcommand{\Argument}{\mathrm{Arg}}\)
\( \newcommand{\norm}[1]{\| #1 \|}\)
\( \newcommand{\inner}[2]{\langle #1, #2 \rangle}\)
\( \newcommand{\Span}{\mathrm{span}}\) \( \newcommand{\AA}{\unicode[.8,0]{x212B}}\)
\( \newcommand{\vectorA}[1]{\vec{#1}} % arrow\)
\( \newcommand{\vectorAt}[1]{\vec{\text{#1}}} % arrow\)
\( \newcommand{\vectorB}[1]{\overset { \scriptstyle \rightharpoonup} {\mathbf{#1}} } \)
\( \newcommand{\vectorC}[1]{\textbf{#1}} \)
\( \newcommand{\vectorD}[1]{\overrightarrow{#1}} \)
\( \newcommand{\vectorDt}[1]{\overrightarrow{\text{#1}}} \)
\( \newcommand{\vectE}[1]{\overset{-\!-\!\rightharpoonup}{\vphantom{a}\smash{\mathbf {#1}}}} \)
\( \newcommand{\vecs}[1]{\overset { \scriptstyle \rightharpoonup} {\mathbf{#1}} } \)
\( \newcommand{\vecd}[1]{\overset{-\!-\!\rightharpoonup}{\vphantom{a}\smash {#1}}} \)
\(\newcommand{\avec}{\mathbf a}\) \(\newcommand{\bvec}{\mathbf b}\) \(\newcommand{\cvec}{\mathbf c}\) \(\newcommand{\dvec}{\mathbf d}\) \(\newcommand{\dtil}{\widetilde{\mathbf d}}\) \(\newcommand{\evec}{\mathbf e}\) \(\newcommand{\fvec}{\mathbf f}\) \(\newcommand{\nvec}{\mathbf n}\) \(\newcommand{\pvec}{\mathbf p}\) \(\newcommand{\qvec}{\mathbf q}\) \(\newcommand{\svec}{\mathbf s}\) \(\newcommand{\tvec}{\mathbf t}\) \(\newcommand{\uvec}{\mathbf u}\) \(\newcommand{\vvec}{\mathbf v}\) \(\newcommand{\wvec}{\mathbf w}\) \(\newcommand{\xvec}{\mathbf x}\) \(\newcommand{\yvec}{\mathbf y}\) \(\newcommand{\zvec}{\mathbf z}\) \(\newcommand{\rvec}{\mathbf r}\) \(\newcommand{\mvec}{\mathbf m}\) \(\newcommand{\zerovec}{\mathbf 0}\) \(\newcommand{\onevec}{\mathbf 1}\) \(\newcommand{\real}{\mathbb R}\) \(\newcommand{\twovec}[2]{\left[\begin{array}{r}#1 \\ #2 \end{array}\right]}\) \(\newcommand{\ctwovec}[2]{\left[\begin{array}{c}#1 \\ #2 \end{array}\right]}\) \(\newcommand{\threevec}[3]{\left[\begin{array}{r}#1 \\ #2 \\ #3 \end{array}\right]}\) \(\newcommand{\cthreevec}[3]{\left[\begin{array}{c}#1 \\ #2 \\ #3 \end{array}\right]}\) \(\newcommand{\fourvec}[4]{\left[\begin{array}{r}#1 \\ #2 \\ #3 \\ #4 \end{array}\right]}\) \(\newcommand{\cfourvec}[4]{\left[\begin{array}{c}#1 \\ #2 \\ #3 \\ #4 \end{array}\right]}\) \(\newcommand{\fivevec}[5]{\left[\begin{array}{r}#1 \\ #2 \\ #3 \\ #4 \\ #5 \\ \end{array}\right]}\) \(\newcommand{\cfivevec}[5]{\left[\begin{array}{c}#1 \\ #2 \\ #3 \\ #4 \\ #5 \\ \end{array}\right]}\) \(\newcommand{\mattwo}[4]{\left[\begin{array}{rr}#1 \amp #2 \\ #3 \amp #4 \\ \end{array}\right]}\) \(\newcommand{\laspan}[1]{\text{Span}\{#1\}}\) \(\newcommand{\bcal}{\cal B}\) \(\newcommand{\ccal}{\cal C}\) \(\newcommand{\scal}{\cal S}\) \(\newcommand{\wcal}{\cal W}\) \(\newcommand{\ecal}{\cal E}\) \(\newcommand{\coords}[2]{\left\{#1\right\}_{#2}}\) \(\newcommand{\gray}[1]{\color{gray}{#1}}\) \(\newcommand{\lgray}[1]{\color{lightgray}{#1}}\) \(\newcommand{\rank}{\operatorname{rank}}\) \(\newcommand{\row}{\text{Row}}\) \(\newcommand{\col}{\text{Col}}\) \(\renewcommand{\row}{\text{Row}}\) \(\newcommand{\nul}{\text{Nul}}\) \(\newcommand{\var}{\text{Var}}\) \(\newcommand{\corr}{\text{corr}}\) \(\newcommand{\len}[1]{\left|#1\right|}\) \(\newcommand{\bbar}{\overline{\bvec}}\) \(\newcommand{\bhat}{\widehat{\bvec}}\) \(\newcommand{\bperp}{\bvec^\perp}\) \(\newcommand{\xhat}{\widehat{\xvec}}\) \(\newcommand{\vhat}{\widehat{\vvec}}\) \(\newcommand{\uhat}{\widehat{\uvec}}\) \(\newcommand{\what}{\widehat{\wvec}}\) \(\newcommand{\Sighat}{\widehat{\Sigma}}\) \(\newcommand{\lt}{<}\) \(\newcommand{\gt}{>}\) \(\newcommand{\amp}{&}\) \(\definecolor{fillinmathshade}{gray}{0.9}\)- Define global warming, climate change, the greenhouse effect, and greenhouse gases.
- Discuss strategies for reducing the intensity of the human influence on the greenhouse effect.
Global warming refers to the increase in the average temperature of the Earth’s atmosphere due to elevated greenhouse gas concentrations, heightening the greenhouse effect. Climate change includes both global warming driven by human-induced emissions of greenhouse gases and the resulting large-scale shifts in weather patterns. Though there have been previous periods of climatic change, since the mid-20th century humans have had an unprecedented impact on Earth's climate system and caused change on a global scale.
The largest driver of warming is the emission of gases that create a greenhouse effect, of which more than 90% are carbon dioxide (CO
2) and methane. Fossil fuel burning (coal, oil, and natural gas) for energy consumption is the main source of these emissions, with additional contributions from agriculture, deforestation, and manufacturing. The human cause of climate change is not disputed by any scientific body of national or international standing. Temperature rise is accelerated or tempered by climate feedbacks, such as loss of sunlight-reflecting snow and ice cover, increased water vapour (a greenhouse gas itself), and changes to land and ocean carbon sinks.
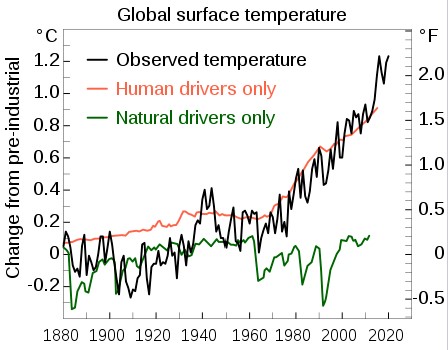
The greenhouse effect is the process by which radiation from a planet's atmosphere warms the planet's surface to a temperature above what it would be without this atmosphere (Figure \(\PageIndex{2}\)). Radiatively active gases (i.e. greenhouse gases) in a planet's atmosphere radiate energy in all directions. Part of this radiation is directed towards the surface, thus warming it. The intensity of downward radiation – that is, the strength of the greenhouse effect – depends on the amount of greenhouse gases that the atmosphere contains. The temperature rises until the intensity of upward radiation from the surface, thus cooling it, balances the downward flow of energy.
Earth's natural greenhouse effect is critical to supporting life and initially was a precursor to life moving out of the ocean onto land. Human activities, mainly the burning of fossil fuels and clear cutting of forests, have increased the greenhouse effect and caused global warming.
The term greenhouse effect is a slight misnomer in the sense that physical greenhouses warm via a different mechanism. The greenhouse effect as an atmospheric mechanism functions through radiative heat loss while a traditional greenhouse as a built structure blocks convective heat loss. The result, however, is an increase in temperature in both cases.
Figure \(\PageIndex{2}\) The greenhouse effect of solar radiation on the Earth's surface caused by emission of greenhouse gases. Source: Wikipedia
Greenhouse Gases and Global Warming
A greenhouse gas (sometimes abbreviated GHG) is a gas that absorbs and emits radiant energy within the thermal infrared range, causing the greenhouse effect. It’s important to realize that water vapor (H2O) is also a greenhouse gas. While humans have little direct impact on water vapor concentrations in the atmosphere, it is still an essential component of the natural greenhouse effect that occurs in our atmosphere. The four major categories of greenhouse gases that have been impacted by humans the most will be discussed in detail below. See Table \(\PageIndex{1}\) for a numeric comparison of these greenhouse gases.
• Carbon dioxide, CO2
• Methane, CH4
• Nitrous oxide, N2O
• Synthetic fluorinated gases, including hydrofluorocarbons (HFCs), perfluorocarbons (PFCs), and sulfur hexafluoride (SF6)
Carbon dioxide (CO2) is the greenhouse gas responsible for most of the human-caused climate change in our atmosphere. It has the highest concentration in the atmosphere of any of the greenhouse gases that we’ll discuss here. Remember that CO2 is a direct product of both combustion and cellular respiration, causing it to be produced in great quantities both naturally and anthropogenically. Any time biomass or fossil fuels are burned, CO2 is released. Major anthropogenic sources include: electricity production from coal-fired and natural gas power plants, transportation, and industry (Chapter 4). To get an idea of how CO2 concentration has changed over time, watch this video compiled by the National Oceanic and Atmospheric Administration (NOAA): http://www.esrl.noaa.gov/gmd/ccgg/trends/history.html. This video contains atmospheric CO2 concentrations measured directly, dating back to 1958, as well as atmospheric CO2 concentrations measured indirectly from ice core data, dating back to 800,000 BCE. By 1990, a quantity of over seven billion tons of carbon (equivalent to 26 billion tons of carbon dioxide when the weight of the oxygen atoms are also considered) was being emitted into the atmosphere every year, much of it from industrialized nations. Similar to the action of the naturally existing greenhouse gases, any additional greenhouse gases leads to an increase in the surface temperature of the Earth.
While CO2 is produced by aerobic cellular respiration, gases such as CH4 and N2O are often the products of anaerobic metabolisms. Agriculture is a major contributor to CH4 emissions. In addition to anaerobic bacteria, methane is also a significant component of natural gas, and is commonly emitted through the mining and use of natural gas and petroleum, in addition to coal mining. Finally, landfills contribute significantly to CH4 emissions, as the waste put into the landfill largely undergoes anaerobic decomposition as it is buried under many layers of trash and soil. Natural sources of CH4 include swamps and wetlands, and volcanoes.
The vast majority of N2O production by humans comes from agricultural land management. While some N2O is naturally emitted to the atmosphere from soil as part of the nitrogen cycle, human changes in land management, largely due to agricultural practices, have greatly increased N2O emissions. Some N2O is also emitted from transportation and industry.
Due to their relatively high concentrations in the atmosphere compared to synthetic gases, CO2, CH4, and N2O, are responsible for most of the human-caused global climate change over the past century. Figure \(\PageIndex{2}\) shows the increases in all three gases since 1750. Ice core data shows us that the atmospheric CO2 concentration never exceeded 300 ppm before the industrial revolution. As of early 2015, the current atmospheric CO2 concentration is 400 ppm.
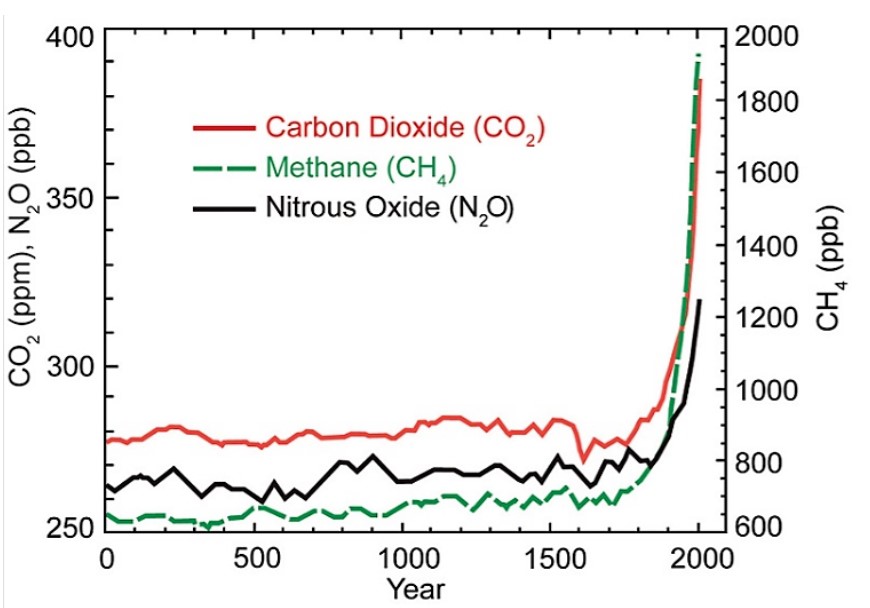
One class of greenhouse gas chemicals that has no natural sources is the fluorinated gases. These include HFCs, PFCs, and SF6, among others. Because these are synthetic chemicals that are only created by humans, these gases were essentially non-existent before the industrial revolution. These synthetic gases are used for a wide variety of applications, from refrigerants to semiconductor manufacturing, and propellants to fire retardants. They tend to have a long lifetime in the atmosphere, as seen in Table \(\PageIndex{1}\). Some of these chemicals, as well as the older chlorofluorocarbons (CFCs), have been phased out by international environmental legislation under the Montreal Protocol. Due to their long lifespan, many of these now banned CFCs remain in the atmosphere. Newer chemical replacements, such as HFCs, provide many of the same industrial applications, but unfortunately have their own environmental consequences.
Just as greenhouse gases differ in their sources and their residence time in the atmosphere, they also differ in their ability to produce the greenhouse effect. This is measured by the global warming potential, or GWP, of each greenhouse gas. The GWP of a greenhouse gas is based on its ability to absorb and scatter energy, as well as its lifetime in the atmosphere. Since CO2 is the most prevalent greenhouse gas, all other greenhouse gases are measured relative to it. As the reference point, CO2 always has a GWP of 1. Note the very high GWP values of the synthetic fluorinated gases in Table \(\PageIndex{1}\). This is largely due to their very long residence time in the atmosphere. Also note the higher GWP values for CH4 and N2O compared to CO2.
Greenhouse gas | Chemical formula or abbreviation | Lifetime in atmosphere | Global warming potential (100-year) |
Carbon dioxide | CO2 | Variable | 1 |
Methane | CH4 | 12 years | 28-36 |
Nitrous oxide | N2O | 114 years | 298 |
Hydrofluorocarbons | Abbreviation: HFCs | 1-270 years | 12-14,800 |
Perfluorocarbons | Abbreviation: PFCs | 2,600-50,000 years | 7,390 |
Sulfur hexafluoride | SF6 | 3,200 years | 22,800 |
Other climate influencers
In addition to greenhouse gases, other manmade changes may be forcing climate change. Increases in near-surface ozone from internal combustion engines, aerosols such as carbon black, mineral dust and aviation-induced exhaust are acting to raise the surface temperature. This primarily occurs due to a decrease in the albedo of light-colored surfaces by the darker-colored carbon black, soot, dust, or particulate matter. As you know, it is more comfortable to wear a white shirt on a hot summer day than a black shirt. Why is this? Because the lighter-colored material bounces more solar radiation back toward space than the darker-colored material does, allowing it to stay cooler. The darker-colored material absorbs more solar radiation, increasing its temperature. Just as the white shirt has a higher albedo than the black shirt, light-colored objects in nature (such as snow) have a higher albedo than dark-colored objects (such as soot or dust). As humans increase the amount of carbon black, soot, dust, and particulates in the atmosphere, we decrease the albedo of light-colored surfaces, causing them to absorb more solar radiation and become warmer than they would without human influence. An example of this can be seen in the snow on Figure \(\PageIndex{3}\).
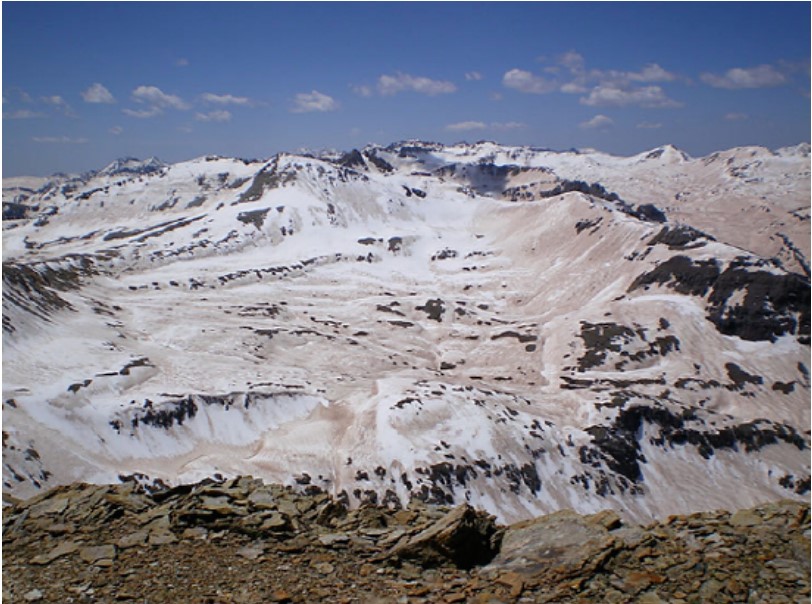
Consequences of Climate Change
We will only discuss some of the consequences of climate change in this section, including changes in temperature, precipitation, ocean level, and ocean acidity. There are many more changes that have been seen, and are projected to continue in the future. These include: changes in the amount and distribution of ice and snow, changes in seasonality, ecosystem shift, and habitat changes of plant and animal populations, in addition to others. For more information about these consequence of climate change, visit this site: http://www.epa.gov/climatechange/sci...ors/index.html.
Temperature and precipitation
Temperature and precipitation are the two most direct impacts on the Earth’s climate due to climate change. By now, you should already understand why an increase in greenhouse gas levels in the atmosphere causes an increase in temperature. But why does it also impact precipitation patterns? As you already know, water vapor is an important component of the Earth’s atmosphere . As the air in the troposphere warms and cools, the amount of water vapor that it holds changes dramatically. Here in Georgia, we have very hot and humid summers. The high summer humidity in this region is possible due to the increased capability warm air has to hold water vapor. Simply put, warmer air can hold more water than cooler air. As air cools, its ability to hold water vapor decreases, and any excess water will leave the air as liquid water. A great example of this is the formation of dew on surfaces overnight. During the day, the temperature is warmer than it is at night, and the air has a relatively high holding capacity for water vapor. When the sun sets, the air cools, decreasing its capacity to hold water vapor. That extra water must go somewhere, and it does that by accumulating on surfaces. Similarly, when warm and cool air fronts collide, the chances for rain and thunderstorms increase. Furthermore, an increase in temperature enhances evaporation occurring at the Earth’s surface. This increased evaporation leads to greater concentrations of water vapor in the atmosphere which can lead to increased precipitation.
The change in temperature that we have already seen in the Earth’s average atmospheric temperature is relatively small (about1.2 °C, according to Figure 7.5.1). However, as with many of the aspects of climate change, the potential for greater changes increases dramatically as time progresses in the future. This can be seen in Figure \(\PageIndex{4}\), which displays a model of the predicted temperature increase. Notice that these changes occur relatively rapidly, and are not uniform across the globe. What might be some of the reasons for this?
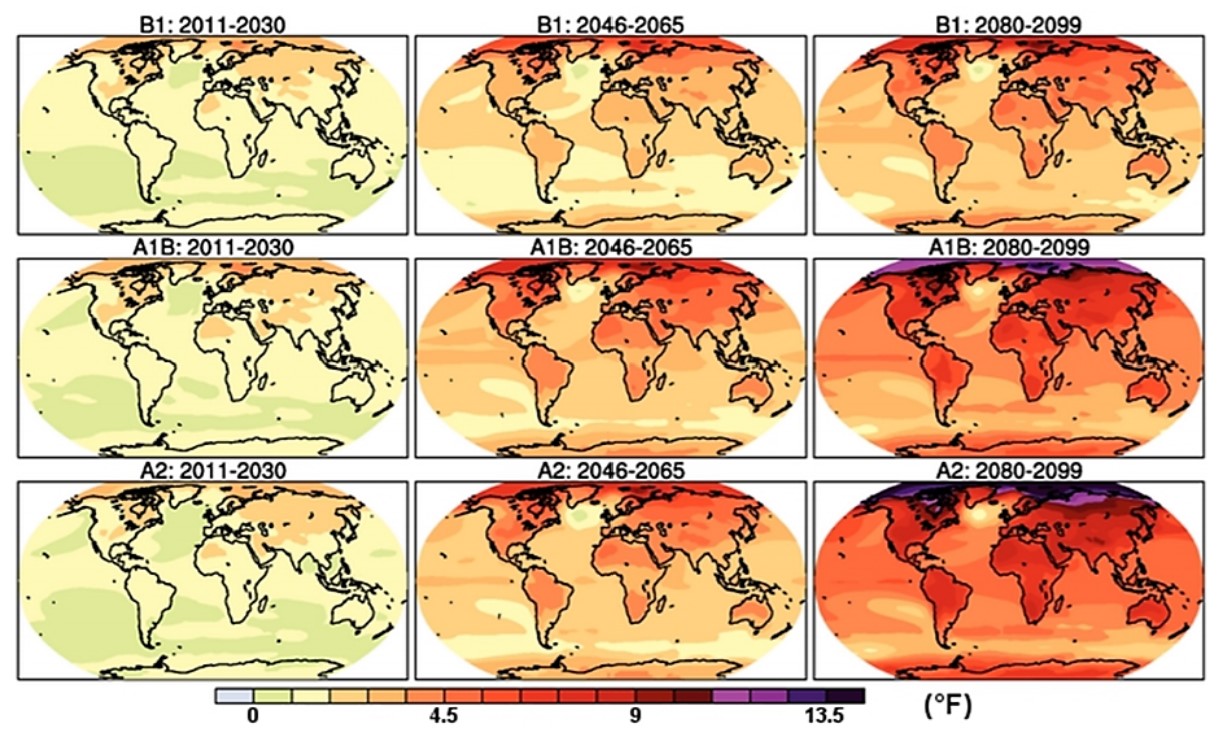
Changes in precipitation occur due to a variety of factors, including changes in atmospheric water vapor content due to changing temperature, as discussed above. Also at play is the heightened evaporation rate of water on Earth’s surface under warmer temperatures. More evaporation leads to more precipitation. Finally, shifts in wind patterns impact the distribution of precipitation events. As you can see in Figure \(\PageIndex{5}\), there are some areas of the globe that are expected to have an increase in precipitation, while others are expected to have a dramatic decrease. Some major population centers projected to have a moderate to severe precipitation increase include (population estimates of the metropolitan area given in parentheses): New York, United States (20.1 million); Bogotá, Colombia (12.1 m.); and Manila, Philippines (11.9 m.). What sort of challenges might these cities face in the future as they deal with this change in their climate?
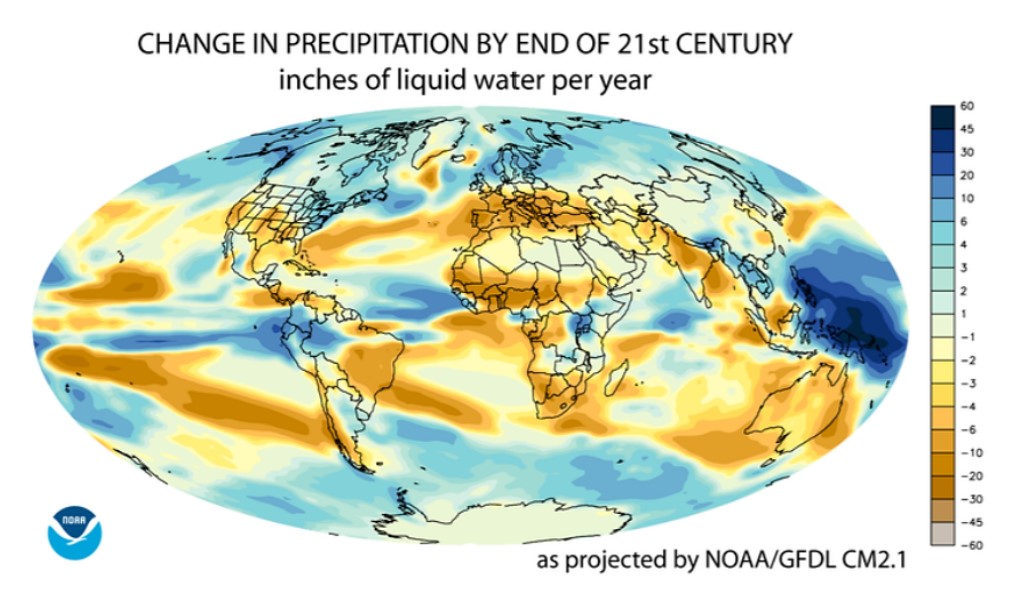
In contrast, many more major metropolitan areas are projected to have a moderate to severe precipitation decrease (droughts) by the end of the 21st century. These include Delhi, India (21.8 m.); Lagos, Nigeria (21 m.); São Paulo, Brazil (20.9 m.); Kolkata, India (14.6 m.); Istanbul, Turkey (14.4 m.); Los Angeles, United States (13.3 m.); Rio de Janeiro, Brazil (12 m.); Paris, France (12 m.); and Lahore, Pakistan (11.3 m.). The largest challenge that these areas are likely to face is a dwindling water supply for drinking and agriculture. See Chapter 8 for more detail on challenges faced by societies to supply clean, reliable water to their populations and farms.
Additional challenges may be felt by all areas of the world with regard to changes in the seasonality or timing of precipitation, as well the form in which precipitation falls (e.g., mist or downpour; rain, ice, or snow). All of these factors affect the availability of soil water for plants, the flow of rivers and streams, and the overall accessibility of water worldwide. Furthermore, scientists predict an increase in the number and severity of storms as climate change progresses. For a full discussion of the potential impacts of this, see the assigned article.
Sea level rise While we know that water continuously cycles around the world, and that the overall quantity of water on Earth will not change due to global climate change, the distribution of this water is changing. In particular, oceans are increasing in volume while land ice stores (such as glaciers) are decreasing. This contributes to an increase in sea level worldwide (Figure \(\PageIndex{6}\)).
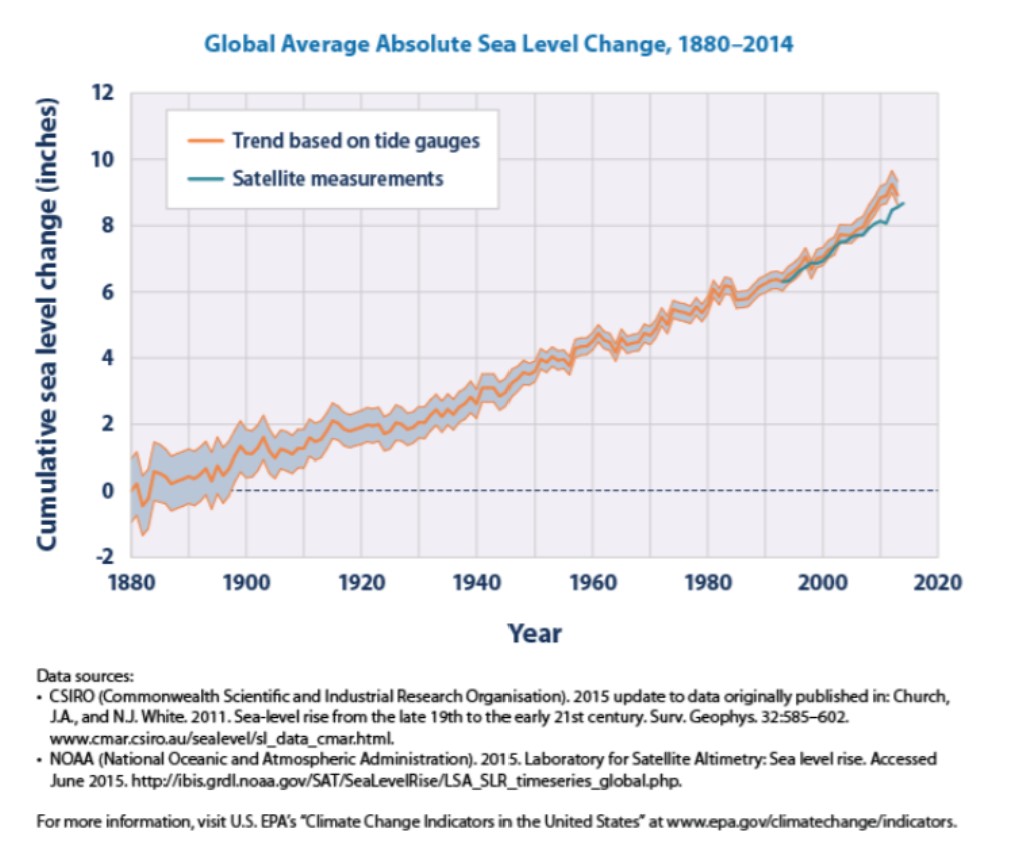
From the data in Figure \(\PageIndex{6}\), we see that sea level has increased at an average of 0.06 inches (0.15 cm) per year over the time period shown above. Most of this rise, however, has occurred within the most recent decades. The rate of increase has gone up to between 0.11 to 0.14 inches (0.28 to 0.36 cm) per year since 1993. There are two forces causing sea level to rise, both caused by climate change. First, the increased global temperature has caused increased ice melting in many regions of the globe. Melting land ice (such as the glacier shown in Figure \(\PageIndex{7}\)) contributes to sea level rise because water that used to be stored in ice sitting on top of land becomes running water which reaches the ocean through runoff. We also observe sea ice melting (see http://www.epa.gov/climatechange/sci ence/indicators/index.html for data and figures). Sea ice, such as the ice that covers the arctic regions of the Northern Hemisphere, has no land underneath it. When it melts, the water stays in the same locations, and the overall sea level does not change.
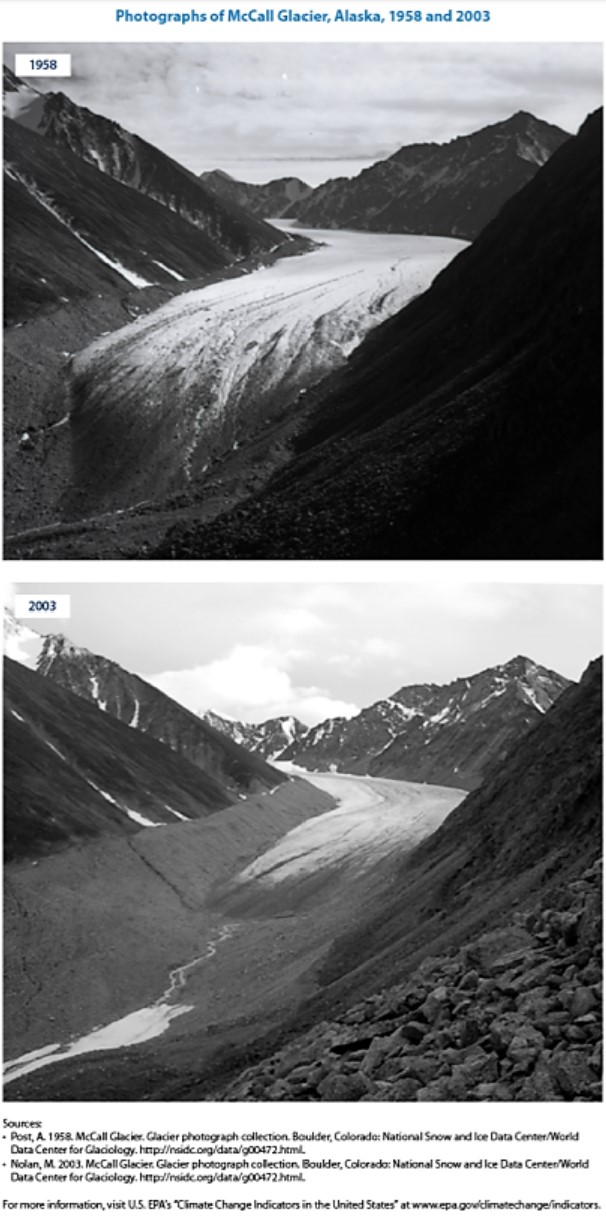
The second factor that influences sea level rise is a phenomenon called thermal expansion. Due to the physical properties of water, as water warms, its density decreases. A less dense substance will have fewer molecules in a given area than a more dense substance (see Chapter 1 supplemental material). This means that as the overall temperature of the oceans increases due to global climate change, the same amount of water molecules will now occupy a slightly larger volume. This may not seem significant, but considering the 1.3 billion trillion liters (264 billion gallons) of water in the ocean, even a small change in density can have large effects on sea level as a whole.
Scientists have already documented sea level rise in some areas of the world, including one familiar to most of us: the Southeastern United States. Figure \(\PageIndex{8}\) depicts the measured land area lost due to increasing sea level since 1996. Note that the Southeast (defined here as the Atlantic coast of North Carolina south to Florida) is particularly susceptible to land area loss due to the gently sloping nature of our coastline. Moving northward into the Mid-Atlantic States (defined here as Virginia north to Long Island, New York), coastal habitats tend to have a steeper geography, which protects against some losses.
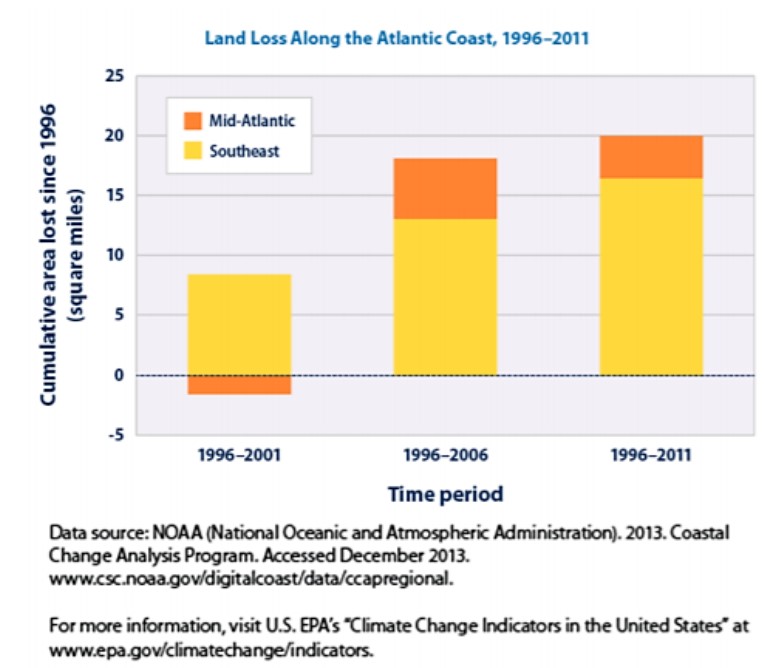
While the ecological effects of sea level rise remain in the United States, we don’t project any catastrophic loss of life, property, or livelihood for some time. This is, in part, due to large investments that we have made in infrastructure to protect our cities and farmlands. This is not the case in many areas of the world. For a discussion of the impacts of sea level rise on less industrialized nations of Bangladesh, Maldives, Kiribati, and Fiji, review the required article reading.
Ocean acidification
Dissolved CO2 is essential for many organisms, including shell-building animals and other organisms that form a hard coating on their exterior (e.g., shellfish, corals, Haptophyte algae). This hard coating is built out of aragonite, a mineral form of the molecule calcium carbonate, CaCO3. These organisms rely on the formation of carbonate ions (see Chapter 1 supplemental material for information on ions), CO3 2-, from dissolved CO2, through a natural, chemical reaction that occurs. This takes place through a chain-reaction equation, where bicarbonate (HCO3- ) is formed as an intermediate, and hydrogen ions (H+ ) are generated (equations \(\PageIndex{1}\) and \(\PageIndex{2}\)).
\[CO_{2} + H_{2}O \leftrightarrow H^{+} + HCO_{3}^{-} \nonumber \]
\[HCO_{3}^{-} \leftrightarrow H^{+} + CO_{3}^{2-} \nonumber \]
To have a better visualization of this process, follow along with the interactive graphic at: http://www.whoi.edu/home/oceanus_ima...ification.html.
As you can see, both equations \(\PageIndex{1}\) and \(\PageIndex{2}\) each produce one H+ . This is significant to water chemistry because an increase in H+ concentration means a decrease in the pH of the water. You can see in Figure \(\PageIndex{9}\) that a lower pH means that the liquid is more acidic. As shown in the interactive graphic, an increase in CO2 in the atmosphere causes additional CO2 to be dissolved in the ocean. This means that more CO2 in the atmosphere leads to more acidic ocean environments.

Unfortunately for shell-building animals, the buildup of H+ in the more acidic ocean environment blocks the absorption of calcium and CO32-, and makes the formation of aragonite more difficult. An aragonite deficit is already being documented in many of the world’s oceans, as shown in Figure \(\PageIndex{10}\).
The increasing acidity of the world’s oceans is resulting in habitat changes across the globe. This is only expected to worsen as atmospheric CO2 levels continue to increase. Many organisms, including the corals that are the foundation species of the beautiful coral reefs, are very sensitive to changes in ocean pH. Scientists have documented cases of ecosystem destruction through coral bleaching, caused by the effects of climate change including ocean acidification and increased temperature. For more information, visit the NOAA Coral Reef Conservation Program website: coralreef.noaa.gov/threats/climate/.

Climate Strategies
While the situation surrounding global climate change is in serious need of our attention, it is important to realize that many scientists, leaders, and concerned citizens are making solutions to climate change part of their life’s work. The two solutions to the problems caused by climate change are mitigation and adaptation, and we will likely need a combination of both in order to prosper in the future.
Adaptation strategies
We know that climate change is already occurring, as we can see and feel the effects of it. For this reason, it is essential to also adapt to our changing environment. This means that we must change our behaviors in response to the changing environment around us. Some adaptation strategies are discussed in the required article reading.
Adaption strategies will vary greatly by region, depending on the largest specific impacts in that area. For example, in the city of Delhi, India, a dramatic decrease in rainfall is projected over the next century (Figure 7.6.2). This city will likely need to implement policies and practices relating to conservation of water, for example: rainwater harvesting, water re-use, and increased irrigation efficiency. Rain-limited cities near oceans, such as Los Angeles, California may choose to use desalination to provide drinking water to their citizens. Desalination involves taking the salt out of seawater to make it potable (Chapter 14).
Cities with low elevations near oceans may need to implement adaptation strategies to rising sea levels, from seawalls and levees to relocation of citizens. One adaptations strategy gaining use is the creation or conservation of wetlands, which provide natural protection against storm surges and flooding.
Mitigation strategies
In general, a strategy to mitigate climate change is one that reduces the amount of greenhouse gases in the atmosphere or prevents additional emissions. Mitigations strategies attempt to “fix” the problems caused by climate change. Governmental regulations regarding fuel efficiency of vehicles is one example of an institutionalized mitigation strategy already in place in the United States and in many other countries around the world. Unlike some other countries, there are no carbon taxes or charges on burning fossil fuels in the United States. This is another governmental mitigation strategy that has been shown to be effective in many countries including India, Japan, France, Costa Rica, Canada, and the United Kingdom.
In addition to government measures and incentives, technology can also be harnessed to mitigate climate change. One strategy for this is the use of carbon capture and sequestration (CCS). Through CCS, 80-90% of the CO2 that would have been emitted to the atmosphere from sources such as a coal-fired power plant is instead captured and then stored deep beneath the Earth’s surface. The CO2 is often injected and sequestered hundreds of miles underground into porous rock formations sealed below an impermeable layer, where it is stored permanently (Figure \(\PageIndex{11}\)).
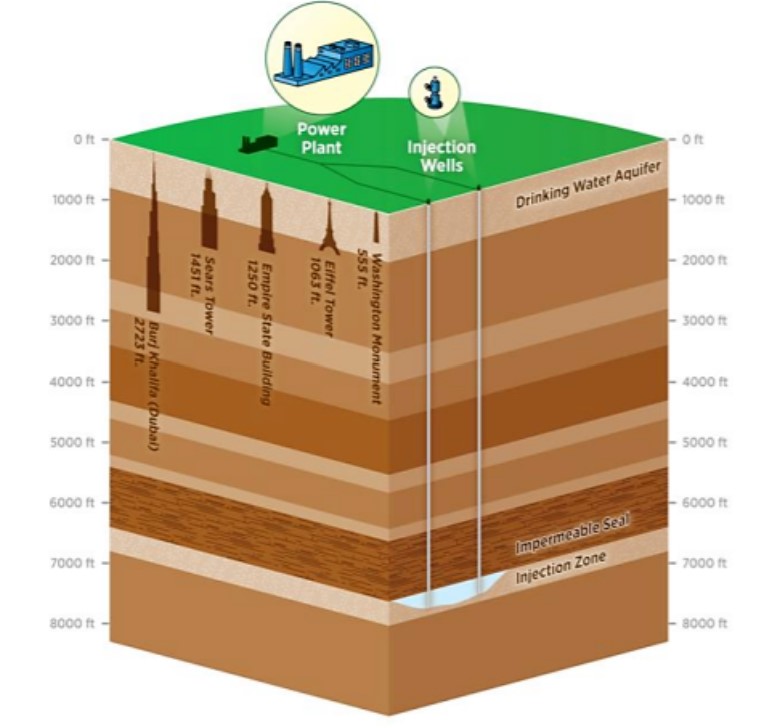
Scientists are also looking into the use of soils and vegetation for carbon storage potential. Proper management of soil and forest ecosystems has been shown to create additional carbon sinks for atmospheric carbon, reducing the overall atmospheric CO2 burden. Increasing soil carbon further benefits communities by providing better-quality soil for agriculture and cultivation.
Technologies related to alternative energy sources (Chapter 15) mitigate climate change by providing people with energy not derived from the combustion of fossil fuels. Finally, simple activities such as energy conservation, choosing to walk or bike instead of driving, and disposing of waste properly are activities that, when done by large numbers of people, actively mitigate climate change by preventing carbon emissions.
Take a moment to identify ways that you personally can be involved in the mitigation of or adaptation to climate change. What changes can you make in your own life to prevent excess carbon emissions? Similar to your ecological footprint, which you should have already calculated in lab, you can also calculate your carbon footprint. Use the EPA’s carbon footprint calculator to do so, and investigate the Reduce Your Emissions section to find ways to decrease your carbon footprint.
Figure \(\PageIndex{12}\) list various technologies and approaches that companies and individuals can adapt to reduce greenhouse gases. Technologies related to alternative energy sources mitigate climate change by providing people with energy not derived from the combustion of fossil fuels. Finally, simple activities such as energy conservation, choosing to walk or bike instead of driving, and disposing of waste properly are activities that, when done by large numbers of people, actively mitigate climate change by preventing carbon emissions.

Solutions for transportation air pollution, emission reductions, can lead to cleaner air and better health.
- Catalytic converters in conjunction with unleaded gasoline and low sulfur levels significantly reduce hydrocarbon and nitrogen oxide emissions.
- Fuel standards reduce exposure to pollutants like lead and benzene. Renewable fuels reduce CO2 emissions.
- Engine technologies like computer controls, variable valve timing, multi-valve engines, turbo charging and gasoline direct injection improve fuel economy and reduce CO2 emissions.
- Transmission technologies like 7+ speeds, dual clutch transmissions, (DCTs), and continuously variable transmissions (CVTs) improve fuel economy and reduce CO2 emissions.
- Diesel filters reduce particulate matter from on road and off road diesel engines. Alternative vehicle technologies like plug-in electric vehicles and fuel cells equals zero tailpipe emissions.
- Better transportation planning for passengers and freight reduce emissions and fuel use.
Summary
- Gases that trap heat in the atmosphere are called greenhouse gases.
- The greenhouse effect is the process by which radiation from a planet's atmosphere warms the planet's surface to a temperature above what it would be without this atmosphere.
- Global warming refers to the increase in the average temperature of the Earth’s atmosphere due to elevated greenhouse gas concentrations, heightening the greenhouse effect.
- Climate change includes both global warming driven by human-induced emissions of greenhouse gasesand the resulting large-scale shifts in weather patterns.
- Greenhouse gases differ in their ability to produce the greenhouse effect as measured by the global warming potential, or GWP. A molecule of CH4 is about 28 times more effective than one of CO2 at absorbing infrared radiation, while N2O is 298 times more effective . The synthetic fluorinated gases are evn much higher (in the thousands). This is largely due to their very long residence time in the atmosphere.
- Different technologies and approaches recommended by the US EPA to to reduce human greenhouse emmissions were provided.
Contributors and Attributions
- Libretext: Introduction to Environmental Science (Zendher et al.)
- US EPA
- Wikipedia