26.2: Alkanes
- Page ID
- 24380
\( \newcommand{\vecs}[1]{\overset { \scriptstyle \rightharpoonup} {\mathbf{#1}} } \)
\( \newcommand{\vecd}[1]{\overset{-\!-\!\rightharpoonup}{\vphantom{a}\smash {#1}}} \)
\( \newcommand{\id}{\mathrm{id}}\) \( \newcommand{\Span}{\mathrm{span}}\)
( \newcommand{\kernel}{\mathrm{null}\,}\) \( \newcommand{\range}{\mathrm{range}\,}\)
\( \newcommand{\RealPart}{\mathrm{Re}}\) \( \newcommand{\ImaginaryPart}{\mathrm{Im}}\)
\( \newcommand{\Argument}{\mathrm{Arg}}\) \( \newcommand{\norm}[1]{\| #1 \|}\)
\( \newcommand{\inner}[2]{\langle #1, #2 \rangle}\)
\( \newcommand{\Span}{\mathrm{span}}\)
\( \newcommand{\id}{\mathrm{id}}\)
\( \newcommand{\Span}{\mathrm{span}}\)
\( \newcommand{\kernel}{\mathrm{null}\,}\)
\( \newcommand{\range}{\mathrm{range}\,}\)
\( \newcommand{\RealPart}{\mathrm{Re}}\)
\( \newcommand{\ImaginaryPart}{\mathrm{Im}}\)
\( \newcommand{\Argument}{\mathrm{Arg}}\)
\( \newcommand{\norm}[1]{\| #1 \|}\)
\( \newcommand{\inner}[2]{\langle #1, #2 \rangle}\)
\( \newcommand{\Span}{\mathrm{span}}\) \( \newcommand{\AA}{\unicode[.8,0]{x212B}}\)
\( \newcommand{\vectorA}[1]{\vec{#1}} % arrow\)
\( \newcommand{\vectorAt}[1]{\vec{\text{#1}}} % arrow\)
\( \newcommand{\vectorB}[1]{\overset { \scriptstyle \rightharpoonup} {\mathbf{#1}} } \)
\( \newcommand{\vectorC}[1]{\textbf{#1}} \)
\( \newcommand{\vectorD}[1]{\overrightarrow{#1}} \)
\( \newcommand{\vectorDt}[1]{\overrightarrow{\text{#1}}} \)
\( \newcommand{\vectE}[1]{\overset{-\!-\!\rightharpoonup}{\vphantom{a}\smash{\mathbf {#1}}}} \)
\( \newcommand{\vecs}[1]{\overset { \scriptstyle \rightharpoonup} {\mathbf{#1}} } \)
\( \newcommand{\vecd}[1]{\overset{-\!-\!\rightharpoonup}{\vphantom{a}\smash {#1}}} \)
\(\newcommand{\avec}{\mathbf a}\) \(\newcommand{\bvec}{\mathbf b}\) \(\newcommand{\cvec}{\mathbf c}\) \(\newcommand{\dvec}{\mathbf d}\) \(\newcommand{\dtil}{\widetilde{\mathbf d}}\) \(\newcommand{\evec}{\mathbf e}\) \(\newcommand{\fvec}{\mathbf f}\) \(\newcommand{\nvec}{\mathbf n}\) \(\newcommand{\pvec}{\mathbf p}\) \(\newcommand{\qvec}{\mathbf q}\) \(\newcommand{\svec}{\mathbf s}\) \(\newcommand{\tvec}{\mathbf t}\) \(\newcommand{\uvec}{\mathbf u}\) \(\newcommand{\vvec}{\mathbf v}\) \(\newcommand{\wvec}{\mathbf w}\) \(\newcommand{\xvec}{\mathbf x}\) \(\newcommand{\yvec}{\mathbf y}\) \(\newcommand{\zvec}{\mathbf z}\) \(\newcommand{\rvec}{\mathbf r}\) \(\newcommand{\mvec}{\mathbf m}\) \(\newcommand{\zerovec}{\mathbf 0}\) \(\newcommand{\onevec}{\mathbf 1}\) \(\newcommand{\real}{\mathbb R}\) \(\newcommand{\twovec}[2]{\left[\begin{array}{r}#1 \\ #2 \end{array}\right]}\) \(\newcommand{\ctwovec}[2]{\left[\begin{array}{c}#1 \\ #2 \end{array}\right]}\) \(\newcommand{\threevec}[3]{\left[\begin{array}{r}#1 \\ #2 \\ #3 \end{array}\right]}\) \(\newcommand{\cthreevec}[3]{\left[\begin{array}{c}#1 \\ #2 \\ #3 \end{array}\right]}\) \(\newcommand{\fourvec}[4]{\left[\begin{array}{r}#1 \\ #2 \\ #3 \\ #4 \end{array}\right]}\) \(\newcommand{\cfourvec}[4]{\left[\begin{array}{c}#1 \\ #2 \\ #3 \\ #4 \end{array}\right]}\) \(\newcommand{\fivevec}[5]{\left[\begin{array}{r}#1 \\ #2 \\ #3 \\ #4 \\ #5 \\ \end{array}\right]}\) \(\newcommand{\cfivevec}[5]{\left[\begin{array}{c}#1 \\ #2 \\ #3 \\ #4 \\ #5 \\ \end{array}\right]}\) \(\newcommand{\mattwo}[4]{\left[\begin{array}{rr}#1 \amp #2 \\ #3 \amp #4 \\ \end{array}\right]}\) \(\newcommand{\laspan}[1]{\text{Span}\{#1\}}\) \(\newcommand{\bcal}{\cal B}\) \(\newcommand{\ccal}{\cal C}\) \(\newcommand{\scal}{\cal S}\) \(\newcommand{\wcal}{\cal W}\) \(\newcommand{\ecal}{\cal E}\) \(\newcommand{\coords}[2]{\left\{#1\right\}_{#2}}\) \(\newcommand{\gray}[1]{\color{gray}{#1}}\) \(\newcommand{\lgray}[1]{\color{lightgray}{#1}}\) \(\newcommand{\rank}{\operatorname{rank}}\) \(\newcommand{\row}{\text{Row}}\) \(\newcommand{\col}{\text{Col}}\) \(\renewcommand{\row}{\text{Row}}\) \(\newcommand{\nul}{\text{Nul}}\) \(\newcommand{\var}{\text{Var}}\) \(\newcommand{\corr}{\text{corr}}\) \(\newcommand{\len}[1]{\left|#1\right|}\) \(\newcommand{\bbar}{\overline{\bvec}}\) \(\newcommand{\bhat}{\widehat{\bvec}}\) \(\newcommand{\bperp}{\bvec^\perp}\) \(\newcommand{\xhat}{\widehat{\xvec}}\) \(\newcommand{\vhat}{\widehat{\vvec}}\) \(\newcommand{\uhat}{\widehat{\uvec}}\) \(\newcommand{\what}{\widehat{\wvec}}\) \(\newcommand{\Sighat}{\widehat{\Sigma}}\) \(\newcommand{\lt}{<}\) \(\newcommand{\gt}{>}\) \(\newcommand{\amp}{&}\) \(\definecolor{fillinmathshade}{gray}{0.9}\)Alkanes are organic compounds that consist entirely of single-bonded carbon and hydrogen atoms and lack any other functional groups. Alkanes have the general formula \(C_nH_{2n+2}\) and can be subdivided into the following three groups: the linear straight-chain alkanes, branched alkanes, and cycloalkanes. Alkanes are also saturated hydrocarbons.
Conformation
When we smell something, the information travels to us via molecules, and almost always these are organic molecules. These molecules can be detected by a variety of organs, including noses in dogs and antennae in crickets, but no matter what organ is sensing the smell, one of the crucial factors in determining how an organism reacts to a compound is the shape of the molecule. The sense of smell depends on thousands of different receptors in the organ, working conceptually on a lock-and-key basis: a molecule with a given shape can fit into a given receptor, and when it does, a signal is sent telling the nervous system that the organism has encountered that particular type of molecule, and the organism reacts appropriately.
What gives a molecule its shape? Given a structural formula, could you determine the shape of the corresponding molecule in three dimensions? Could you predict its biological activity, including not only its smell, but also a host of other behaviors linked to the shape of molecular messengers, such as anti-cancer activity or narcotic properties? These questions are at the cutting edge of biological chemistry. Although they are best answered through computer modeling, we can develop some of the qualitative ideas used in these models.
In the eclipsed conformation of ethane the smallest dihedral angle is 0º, whereas in the staggered conformation it is 60º, which can be seen more clearly in the respective Newman projection.
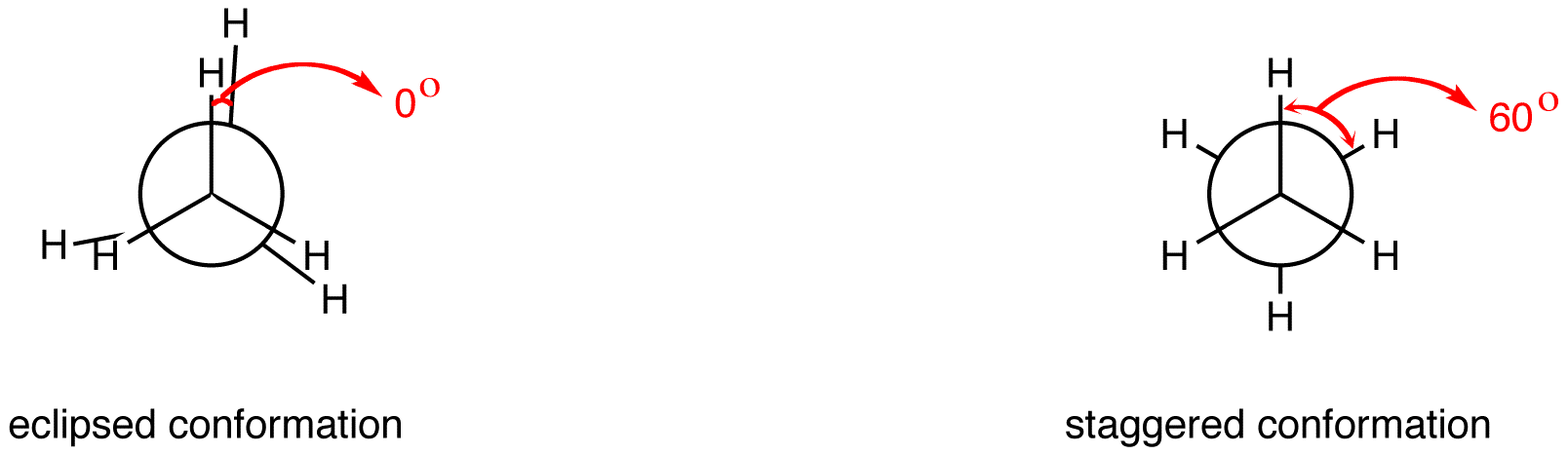
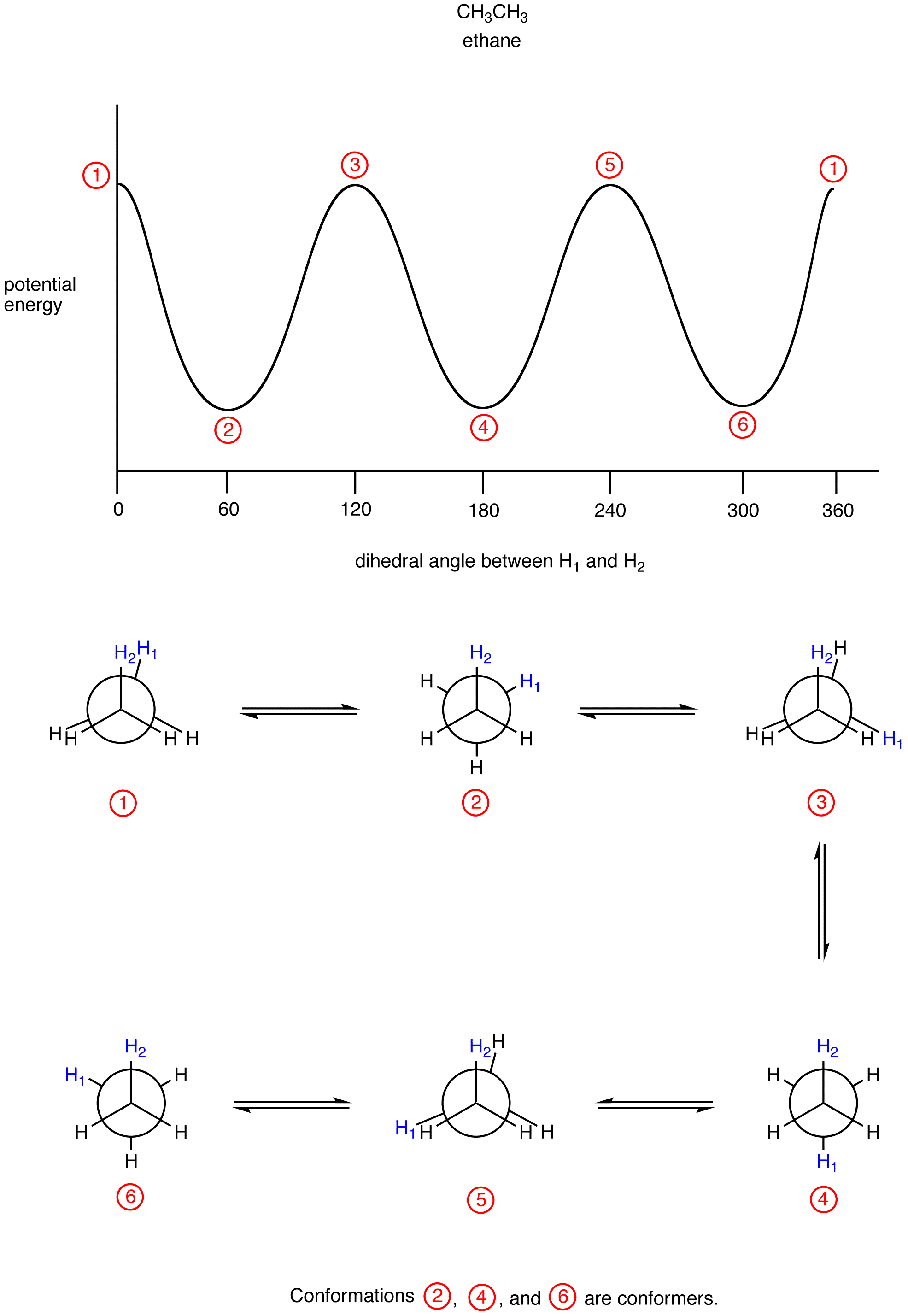
Conformations of Butane
In the case of ethane, conformational changes are very subtle, but in others they are more obvious. Butane (CH3CH2CH2CH3) has four tetrahedral carbons and three carbon-carbon bonds connecting them together. Let's number the carbons along the chain C1, C2, C3 and C4. Rotating around the C-C bonds connected to the terminal carbons -- C1-C2 and C3-C4 -- only subtle changes in shape would be apparent. However, rotating about C2-C3 produces some pretty obvious shape changes.
Pay attention to where the two methyl groups are with respect to each other. If we call C1-C2-C3-C4 the dihedral angle, then at 0 degrees the molecule is in an eclipsed conformation, apparent when looking at the Newman projection. Looked at from other vantage points, the molecule is curled up into the shape of a letter C (Figure \(\PageIndex{2}\)).
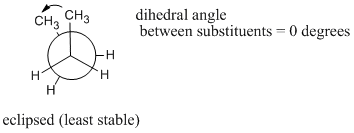
At 60 degrees, the molecule is no longer eclipsed, and just as in ethane the energy is a little bit lower, but the overall shape when viewed from the side is still a sort of twisted C (Figure \(\PageIndex{3}\)).
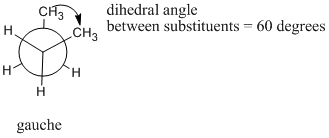
At 120 degrees the molecule is eclipsed again, but from the side it has now twisted almost into a shape like the letter Z ( Figure \(\PageIndex{4}\)).
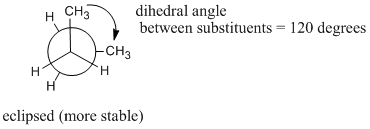
At 180 degrees, the molecule is staggered again and has settled into a regular, zig-zag, letter Z shape.
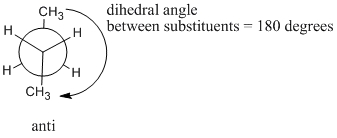
These conformations of butane are really pretty different. Which shape would the molecule prefer? From what we learned about ethane, we could probably rule out the eclipsed conformations. Each of those would have 3 kcal/mol of torsional strain.
However, there is another factor that destabilizes the initial conformation at 0 degrees, in favor of the Z-shaped one with the methyl groups 180 degrees apart from each other. This factor is called "sterics" and it refers to the idea that molecules, or parts of molecules, take up space, and so two parts of the butane can't occupy the same place at the same time. Put more simply, sterics refers to crowdedness. When the two methyl groups in butane are too close together, they are too crowded, and they are at higher energy. When they get farther apart, crowding subsides and the energy in the molecule goes down.
- Torsional strain forces bonds on neighboring carbons to be staggered.
- Steric strain forces groups away from each other to relieve crowding.
- Torsion and sterics both contribute to strain energy.
- Strain can be released by getting the molecule in a lower energy conformation.
For butane, that means getting those two methyl groups away from each other and keeping the bonds staggered. There is some additional jargon that is used to describe these butane conformations:
- In an anti conformer, the largest groups are 180 degrees from each other.
- In a gauche conformer, the bonds are staggered but the largest groups are 600 from each other.
Preparation of Alkanes
An important way to generate alkanes is the hydrogenation of an alkene (discussed later) The double bond of an alkene consists of a sigma (σ) bond and a pi (π) bond. Because the carbon-carbon π bond is relatively weak, it is quite reactive and can be easily broken and reagents can be added to carbon. Reagents are added through the formation of single bonds to carbon in an addition reaction.
An example of an alkene addition reaction is a process called hydrogenation.In a hydrogenation reaction, two hydrogen atoms are added across the double bond of an alkene, resulting in a saturated alkane. Hydrogenation of a double bond is a thermodynamically favorable reaction because it forms a more stable (lower energy) product. In other words, the energy of the product is lower than the energy of the reactant; thus it is exothermic (heat is released). The heat released is called the heat of hydrogenation, which is an indicator of a molecule’s stability.
Although the hydrogenation of an alkene is a thermodynamically favorable reaction, it will not proceed without the addition of a catalyst. Common catalysts used are insoluble metals such as palladium and platinum. Hydrogenation reactions are extensively used to create commercial goods.Hydrogenation is used in the food industry to make a large variety of manufactured goods, like spreads and shortenings, from liquid oils. This process also increases the chemical stability of products and yields semi-solid products like margarine. Hydrogenation is also used in coal processing. Solid coal is converted to a liquid through the addition of hydrogen. Liquefying coal makes it available to be used as fuel.
Alkanes from Petroleum
Cracking is the name given to breaking up large hydrocarbon molecules into smaller and more useful bits. This is achieved by using high pressures and temperatures without a catalyst, or lower temperatures and pressures in the presence of a catalyst. The source of the large hydrocarbon molecules is often the naphtha fraction or the gas oil fraction from the fractional distillation of crude oil (petroleum). There is not any single unique reaction happening in the cracker. The hydrocarbon molecules are broken up in a fairly random way to produce mixtures of smaller hydrocarbons, some of which have carbon-carbon double bonds. One possible reaction involving the hydrocarbon C15H32 might be: