22.11: Boron
- Last updated
- Save as PDF
- Page ID
- 21910
\( \newcommand{\vecs}[1]{\overset { \scriptstyle \rightharpoonup} {\mathbf{#1}} } \)
\( \newcommand{\vecd}[1]{\overset{-\!-\!\rightharpoonup}{\vphantom{a}\smash {#1}}} \)
\( \newcommand{\id}{\mathrm{id}}\) \( \newcommand{\Span}{\mathrm{span}}\)
( \newcommand{\kernel}{\mathrm{null}\,}\) \( \newcommand{\range}{\mathrm{range}\,}\)
\( \newcommand{\RealPart}{\mathrm{Re}}\) \( \newcommand{\ImaginaryPart}{\mathrm{Im}}\)
\( \newcommand{\Argument}{\mathrm{Arg}}\) \( \newcommand{\norm}[1]{\| #1 \|}\)
\( \newcommand{\inner}[2]{\langle #1, #2 \rangle}\)
\( \newcommand{\Span}{\mathrm{span}}\)
\( \newcommand{\id}{\mathrm{id}}\)
\( \newcommand{\Span}{\mathrm{span}}\)
\( \newcommand{\kernel}{\mathrm{null}\,}\)
\( \newcommand{\range}{\mathrm{range}\,}\)
\( \newcommand{\RealPart}{\mathrm{Re}}\)
\( \newcommand{\ImaginaryPart}{\mathrm{Im}}\)
\( \newcommand{\Argument}{\mathrm{Arg}}\)
\( \newcommand{\norm}[1]{\| #1 \|}\)
\( \newcommand{\inner}[2]{\langle #1, #2 \rangle}\)
\( \newcommand{\Span}{\mathrm{span}}\) \( \newcommand{\AA}{\unicode[.8,0]{x212B}}\)
\( \newcommand{\vectorA}[1]{\vec{#1}} % arrow\)
\( \newcommand{\vectorAt}[1]{\vec{\text{#1}}} % arrow\)
\( \newcommand{\vectorB}[1]{\overset { \scriptstyle \rightharpoonup} {\mathbf{#1}} } \)
\( \newcommand{\vectorC}[1]{\textbf{#1}} \)
\( \newcommand{\vectorD}[1]{\overrightarrow{#1}} \)
\( \newcommand{\vectorDt}[1]{\overrightarrow{\text{#1}}} \)
\( \newcommand{\vectE}[1]{\overset{-\!-\!\rightharpoonup}{\vphantom{a}\smash{\mathbf {#1}}}} \)
\( \newcommand{\vecs}[1]{\overset { \scriptstyle \rightharpoonup} {\mathbf{#1}} } \)
\( \newcommand{\vecd}[1]{\overset{-\!-\!\rightharpoonup}{\vphantom{a}\smash {#1}}} \)
\(\newcommand{\avec}{\mathbf a}\) \(\newcommand{\bvec}{\mathbf b}\) \(\newcommand{\cvec}{\mathbf c}\) \(\newcommand{\dvec}{\mathbf d}\) \(\newcommand{\dtil}{\widetilde{\mathbf d}}\) \(\newcommand{\evec}{\mathbf e}\) \(\newcommand{\fvec}{\mathbf f}\) \(\newcommand{\nvec}{\mathbf n}\) \(\newcommand{\pvec}{\mathbf p}\) \(\newcommand{\qvec}{\mathbf q}\) \(\newcommand{\svec}{\mathbf s}\) \(\newcommand{\tvec}{\mathbf t}\) \(\newcommand{\uvec}{\mathbf u}\) \(\newcommand{\vvec}{\mathbf v}\) \(\newcommand{\wvec}{\mathbf w}\) \(\newcommand{\xvec}{\mathbf x}\) \(\newcommand{\yvec}{\mathbf y}\) \(\newcommand{\zvec}{\mathbf z}\) \(\newcommand{\rvec}{\mathbf r}\) \(\newcommand{\mvec}{\mathbf m}\) \(\newcommand{\zerovec}{\mathbf 0}\) \(\newcommand{\onevec}{\mathbf 1}\) \(\newcommand{\real}{\mathbb R}\) \(\newcommand{\twovec}[2]{\left[\begin{array}{r}#1 \\ #2 \end{array}\right]}\) \(\newcommand{\ctwovec}[2]{\left[\begin{array}{c}#1 \\ #2 \end{array}\right]}\) \(\newcommand{\threevec}[3]{\left[\begin{array}{r}#1 \\ #2 \\ #3 \end{array}\right]}\) \(\newcommand{\cthreevec}[3]{\left[\begin{array}{c}#1 \\ #2 \\ #3 \end{array}\right]}\) \(\newcommand{\fourvec}[4]{\left[\begin{array}{r}#1 \\ #2 \\ #3 \\ #4 \end{array}\right]}\) \(\newcommand{\cfourvec}[4]{\left[\begin{array}{c}#1 \\ #2 \\ #3 \\ #4 \end{array}\right]}\) \(\newcommand{\fivevec}[5]{\left[\begin{array}{r}#1 \\ #2 \\ #3 \\ #4 \\ #5 \\ \end{array}\right]}\) \(\newcommand{\cfivevec}[5]{\left[\begin{array}{c}#1 \\ #2 \\ #3 \\ #4 \\ #5 \\ \end{array}\right]}\) \(\newcommand{\mattwo}[4]{\left[\begin{array}{rr}#1 \amp #2 \\ #3 \amp #4 \\ \end{array}\right]}\) \(\newcommand{\laspan}[1]{\text{Span}\{#1\}}\) \(\newcommand{\bcal}{\cal B}\) \(\newcommand{\ccal}{\cal C}\) \(\newcommand{\scal}{\cal S}\) \(\newcommand{\wcal}{\cal W}\) \(\newcommand{\ecal}{\cal E}\) \(\newcommand{\coords}[2]{\left\{#1\right\}_{#2}}\) \(\newcommand{\gray}[1]{\color{gray}{#1}}\) \(\newcommand{\lgray}[1]{\color{lightgray}{#1}}\) \(\newcommand{\rank}{\operatorname{rank}}\) \(\newcommand{\row}{\text{Row}}\) \(\newcommand{\col}{\text{Col}}\) \(\renewcommand{\row}{\text{Row}}\) \(\newcommand{\nul}{\text{Nul}}\) \(\newcommand{\var}{\text{Var}}\) \(\newcommand{\corr}{\text{corr}}\) \(\newcommand{\len}[1]{\left|#1\right|}\) \(\newcommand{\bbar}{\overline{\bvec}}\) \(\newcommand{\bhat}{\widehat{\bvec}}\) \(\newcommand{\bperp}{\bvec^\perp}\) \(\newcommand{\xhat}{\widehat{\xvec}}\) \(\newcommand{\vhat}{\widehat{\vvec}}\) \(\newcommand{\uhat}{\widehat{\uvec}}\) \(\newcommand{\what}{\widehat{\wvec}}\) \(\newcommand{\Sighat}{\widehat{\Sigma}}\) \(\newcommand{\lt}{<}\) \(\newcommand{\gt}{>}\) \(\newcommand{\amp}{&}\) \(\definecolor{fillinmathshade}{gray}{0.9}\)Learning Objectives
- To understand the trends in properties and the reactivity of the group 13 elements.
Group 13 is the first group to span the dividing line between metals and nonmetals, so its chemistry is more diverse than that of groups 1 and 2, which include only metallic elements. Except for the lightest element (boron), the group 13 elements are all relatively electropositive; that is, they tend to lose electrons in chemical reactions rather than gain them. Although group 13 includes aluminum, the most abundant metal on Earth, none of these elements was known until the early 19th century because they are never found in nature in their free state. Elemental boron and aluminum, which were first prepared by reducing B2O3 and AlCl3, respectively, with potassium, could not be prepared until potassium had been isolated and shown to be a potent reductant. Indium (In) and thallium (Tl) were discovered in the 1860s by using spectroscopic techniques, long before methods were available for isolating them. Indium, named for its indigo (deep blue-violet) emission line, was first observed in the spectrum of zinc ores, while thallium (from the Greek thallos, meaning “a young, green shoot of a plant”) was named for its brilliant green emission line. Gallium (Ga; Mendeleev’s eka-aluminum) was discovered in 1875 by the French chemist Paul Émile Lecoq de Boisbaudran during a systematic search for Mendeleev’s “missing” element in group 13.
Group 13 elements are never found in nature in their free state.
Preparation and General Properties of the Group 13 Elements
As reductants, the group 13 elements are less powerful than the alkali metals and alkaline earth metals. Nevertheless, their compounds with oxygen are thermodynamically stable, and large amounts of energy are needed to isolate even the two most accessible elements—boron and aluminum—from their oxide ores.

Although boron is relatively rare (it is about 10,000 times less abundant than aluminum), concentrated deposits of borax [Na2B4O5(OH)4·8H2O] are found in ancient lake beds (Figure \(\PageIndex{1}\)) and were used in ancient times for making glass and glazing pottery. Boron is produced on a large scale by reacting borax with acid to produce boric acid [B(OH)3], which is then dehydrated to the oxide (B2O3). Reduction of the oxide with magnesium or sodium gives amorphous boron that is only about 95% pure:
\[\mathrm{Na_2B_4O_5(OH)_4\cdot8H_2O(s)}\xrightarrow{\textrm{acid}}\mathrm{B(OH)_3(s)}\xrightarrow{\Delta}\mathrm{B_2O_3(s)} \label{Eq1}\]
\[\mathrm{B_2O_3(s)}+\mathrm{3Mg(s)}\xrightarrow{\Delta}\mathrm{2B(s)}+\mathrm{3MgO(s)} \label{Eq2}\]
Pure, crystalline boron, however, is extremely difficult to obtain because of its high melting point (2300°C) and the highly corrosive nature of liquid boron. It is usually prepared by reducing pure BCl3 with hydrogen gas at high temperatures or by the thermal decomposition of boron hydrides such as diborane (B2H6):
\[\mathrm{BCl_3(g)}+\frac{3}{2}\mathrm{H_2(g)}\rightarrow\mathrm{B(s)}+\mathrm{3HCl(g)} \label{Eq3}\]
\[B_2H_{6(g)} \rightarrow 2B_{(s)} + 3H_{2(g)} \label{Eq4}\]
The reaction shown in Equation \(\ref{Eq3}\) is used to prepare boron fibers, which are stiff and light. Hence they are used as structural reinforcing materials in objects as diverse as the US space shuttle and the frames of lightweight bicycles that are used in races such as the Tour de France. Boron is also an important component of many ceramics and heat-resistant borosilicate glasses, such as Pyrex, which is used for ovenware and laboratory glassware.
In contrast to boron, deposits of aluminum ores such as bauxite, a hydrated form of Al2O3, are abundant. With an electrical conductivity about twice that of copper on a weight for weight basis, aluminum is used in more than 90% of the overhead electric power lines in the United States. However, because aluminum–oxygen compounds are stable, obtaining aluminum metal from bauxite is an expensive process. Aluminum is extracted from oxide ores by treatment with a strong base, which produces the soluble hydroxide complex [Al(OH)4]−. Neutralization of the resulting solution with gaseous CO2 results in the precipitation of Al(OH)3:
\[2[Al(OH)_4]^−_{(aq)} + CO_{2(g)} \rightarrow 2Al(OH)_{3(s)} + CO^{2−}_{3(aq)} + H_2O_{(l)} \label{Eq5}\]
Thermal dehydration of Al(OH)3 produces Al2O3, and metallic aluminum is obtained by the electrolytic reduction of Al2O3 using the Hall–Heroult process. Of the group 13 elements, only aluminum is used on a large scale: for example, each Boeing 777 airplane is about 50% aluminum by mass.

The other members of group 13 are rather rare: gallium is approximately 5000 times less abundant than aluminum, and indium and thallium are even scarcer. Consequently, these metals are usually obtained as by-products in the processing of other metals. The extremely low melting point of gallium (29.6°C), however, makes it easy to separate from aluminum. Due to its low melting point and high boiling point, gallium is used as a liquid in thermometers that have a temperature range of almost 2200°C. Indium and thallium, the heavier group 13 elements, are found as trace impurities in sulfide ores of zinc and lead. Indium is used as a crushable seal for high-vacuum cryogenic devices, and its alloys are used as low-melting solders in electronic circuit boards. Thallium, on the other hand, is so toxic that the metal and its compounds have few uses. Both indium and thallium oxides are released in flue dust when sulfide ores are converted to metal oxides and SO2. Until relatively recently, these and other toxic elements were allowed to disperse in the air, creating large “dead zones” downwind of a smelter. The flue dusts are now trapped and serve as a relatively rich source of elements such as In and Tl (as well as Ge, Cd, Te, and As).
Table \(\PageIndex{1}\) summarizes some important properties of the group 13 elements. Notice the large differences between boron and aluminum in size, ionization energy, electronegativity, and standard reduction potential, which is consistent with the observation that boron behaves chemically like a nonmetal and aluminum like a metal. All group 13 elements have ns2np1 valence electron configurations, and all tend to lose their three valence electrons to form compounds in the +3 oxidation state. The heavier elements in the group can also form compounds in the +1 oxidation state formed by the formal loss of the single np valence electron. Because the group 13 elements generally contain only six valence electrons in their neutral compounds, these compounds are all moderately strong Lewis acids.
Property | Boron | Aluminum* | Gallium | Indium | Thallium |
---|---|---|---|---|---|
*This is the name used in the United States; the rest of the world inserts an extra i and calls it aluminium. | |||||
†The configuration shown does not include filled d and f subshells. | |||||
‡The values cited are for six-coordinate ions in the most common oxidation state, except for Al3+, for which the value for the four-coordinate ion is given. The B3+ ion is not a known species; the radius cited is an estimated four-coordinate value. | |||||
§X is Cl, Br, or I. Reaction with F2 gives the trifluorides (MF3) for all group 13 elements. | |||||
atomic symbol | B | Al | Ga | In | Tl |
atomic number | 5 | 13 | 31 | 49 | 81 |
atomic mass (amu) | 10.81 | 26.98 | 69.72 | 114.82 | 204.38 |
valence electron configuration† | 2s22p1 | 3s23p1 | 4s24p1 | 5s25p1 | 6s26p1 |
melting point/boiling point (°C) | 2075/4000 | 660/2519 | 29.7/2204 | 156.6/2072 | 304/1473 |
density (g/cm3) at 25°C | 2.34 | 2.70 | 5.91 | 7.31 | 11.8 |
atomic radius (pm) | 87 | 118 | 136 | 156 | 156 |
first ionization energy (kJ/mol) | 801 | 578 | 579 | 558 | 589 |
most common oxidation state | +3 | +3 | +3 | +3 | +1 |
ionic radius (pm)‡ | −25 | 54 | 62 | 80 | 162 |
electron affinity (kJ/mol) | −27 | −42 | −40 | −39 | −37 |
electronegativity | 2.0 | 1.6 | 1.8 | 1.8 | 1.8 |
standard reduction potential (E°, V) | −0.87 | −1.66 | −0.55 | −0.34 | +0.741 of M3+(aq) |
product of reaction with O2 | B2O3 | Al2O3 | Ga2O3 | In2O3 | Tl2O |
type of oxide | acidic | amphoteric | amphoteric | amphoteric | basic |
product of reaction with N2 | BN | AlN | GaN | InN | none |
product of reaction with X2§ | BX3 | Al2X6 | Ga2X6 | In2X6 | TlX |
Neutral compounds of the group 13 elements are electron deficient, so they are generally moderately strong Lewis acids.
In contrast to groups 1 and 2, the group 13 elements show no consistent trends in ionization energies, electron affinities, and reduction potentials, whereas electronegativities actually increase from aluminum to thallium. Some of these anomalies, especially for the series Ga, In, Tl, can be explained by the increase in the effective nuclear charge (Zeff) that results from poor shielding of the nuclear charge by the filled (n − 1)d10 and (n − 2)f14 subshells. Consequently, although the actual nuclear charge increases by 32 as we go from indium to thallium, screening by the filled 5d and 4f subshells is so poor that Zeff increases significantly from indium to thallium. Thus the first ionization energy of thallium is actually greater than that of indium.
Anomalies in periodic trends among Ga, In, and Tl can be explained by the increase in the effective nuclear charge due to poor shielding.
Reactions and Compounds of Boron
Elemental boron is a semimetal that is remarkably unreactive; in contrast, the other group 13 elements all exhibit metallic properties and reactivity. We therefore consider the reactions and compounds of boron separately from those of other elements in the group. All group 13 elements have fewer valence electrons than valence orbitals, which generally results in delocalized, metallic bonding. With its high ionization energy, low electron affinity, low electronegativity, and small size, however, boron does not form a metallic lattice with delocalized valence electrons. Instead, boron forms unique and intricate structures that contain multicenter bonds, in which a pair of electrons holds together three or more atoms.

Elemental boron forms multicenter bonds, whereas the other group 13 elements exhibit metallic bonding.
The basic building block of elemental boron is not the individual boron atom, as would be the case in a metal, but rather the B12 icosahedron. Because these icosahedra do not pack together very well, the structure of solid boron contains voids, resulting in its low density (Figure \(\PageIndex{3}\)). Elemental boron can be induced to react with many nonmetallic elements to give binary compounds that have a variety of applications. For example, plates of boron carbide (B4C) can stop a 30-caliber, armor-piercing bullet, yet they weigh 10%–30% less than conventional armor. Other important compounds of boron with nonmetals include boron nitride (BN), which is produced by heating boron with excess nitrogen (Equation \(\ref{Eq22.6}\)); boron oxide (B2O3), which is formed when boron is heated with excess oxygen (Equation \(\ref{Eq22.7}\)); and the boron trihalides (BX3), which are formed by heating boron with excess halogen (Equation \(\ref{Eq22.8}\)).
\[\mathrm{2B(s)}+\mathrm{N_2(g)}\xrightarrow{\Delta}\mathrm{2BN(s)} \label{Eq22.6}\]
\[\mathrm{4B(s)} + \mathrm{3O_2(g)}\xrightarrow{\Delta}\mathrm{2B_2O_3(s)}\label{Eq22.7}\]
\[\mathrm{2B(s)} +\mathrm{3X_2(g)}\xrightarrow{\Delta}\mathrm{2BX_3(g)}\label{Eq22.8}\]
As is typical of elements lying near the dividing line between metals and nonmetals, many compounds of boron are amphoteric, dissolving in either acid or base.Boron nitride is similar in many ways to elemental carbon. With eight electrons, the B–N unit is isoelectronic with the C–C unit, and B and N have the same average size and electronegativity as C. The most stable form of BN is similar to graphite, containing six-membered B3N3 rings arranged in layers. At high temperature and pressure, hexagonal BN converts to a cubic structure similar to diamond, which is one of the hardest substances known. Boron oxide (B2O3) contains layers of trigonal planar BO3 groups (analogous to BX3) in which the oxygen atoms bridge two boron atoms. It dissolves many metal and nonmetal oxides, including SiO2, to give a wide range of commercially important borosilicate glasses. A small amount of CoO gives the deep blue color characteristic of “cobalt blue” glass.
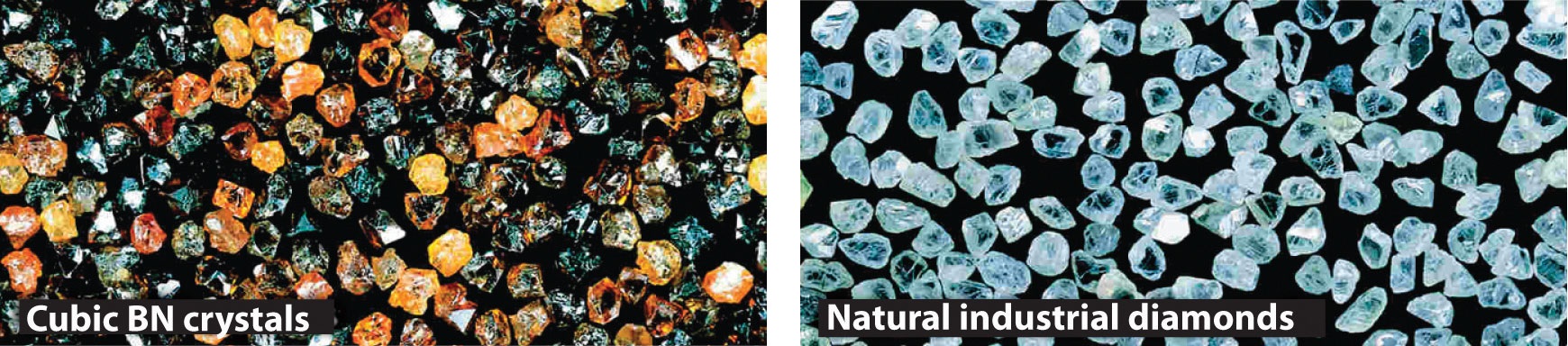
At high temperatures, boron also reacts with virtually all metals to give metal borides that contain regular three-dimensional networks, or clusters, of boron atoms. The structures of two metal borides—ScB12 and CaB6—are shown in Figure \(\PageIndex{4}\). Because metal-rich borides such as ZrB2 and TiB2 are hard and corrosion resistant even at high temperatures, they are used in applications such as turbine blades and rocket nozzles.
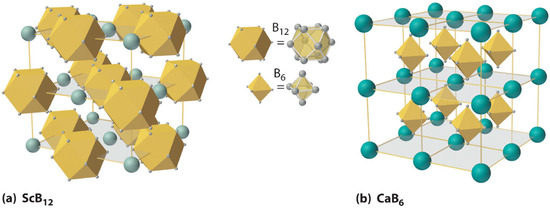
Boron hydrides were not discovered until the early 20th century, when the German chemist Alfred Stock undertook a systematic investigation of the binary compounds of boron and hydrogen, although binary hydrides of carbon, nitrogen, oxygen, and fluorine have been known since the 18th century. Between 1912 and 1936, Stock oversaw the preparation of a series of boron–hydrogen compounds with unprecedented structures that could not be explained with simple bonding theories. All these compounds contain multicenter bonds. The simplest example is diborane (B2H6), which contains two bridging hydrogen atoms (part (a) in Figure \(\PageIndex{5}\). An extraordinary variety of polyhedral boron–hydrogen clusters is now known; one example is the B12H122− ion, which has a polyhedral structure similar to the icosahedral B12 unit of elemental boron, with a single hydrogen atom bonded to each boron atom.
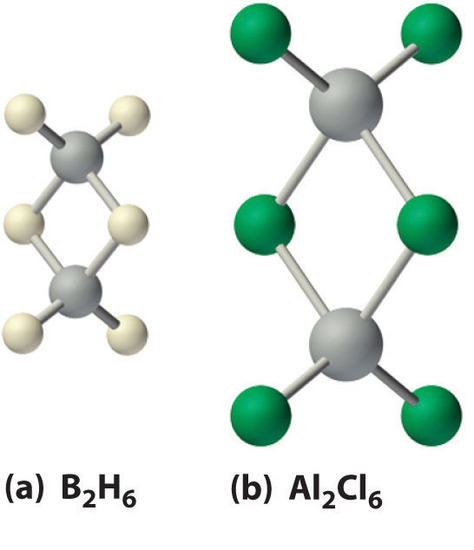
A related class of polyhedral clusters, the carboranes, contain both CH and BH units; an example is shown here. Replacing the hydrogen atoms bonded to carbon with organic groups produces substances with novel properties, some of which are currently being investigated for their use as liquid crystals and in cancer chemotherapy.
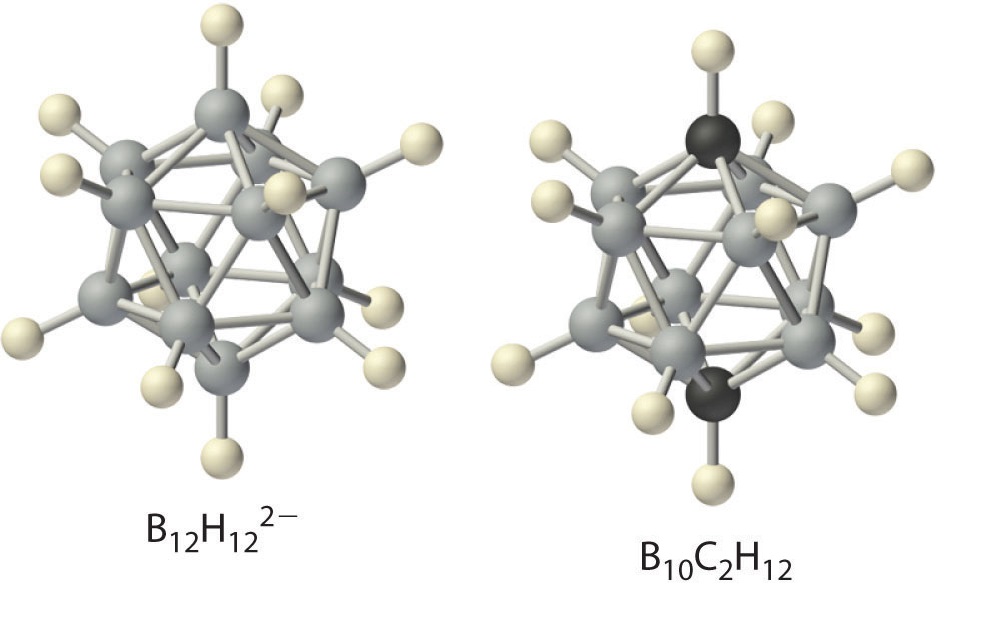
The enthalpy of combustion of diborane (B2H6) is −2165 kJ/mol, one of the highest values known:
\[B_2H_{6(g)} + 3O_{2(g)} \rightarrow B_2O_{3(s)} + 3H_2O(l)\;\;\; ΔH_{comb} = −2165\; kJ/mol \label{Eq 22.9}\]
Consequently, the US military explored using boron hydrides as rocket fuels in the 1950s and 1960s. This effort was eventually abandoned because boron hydrides are unstable, costly, and toxic, and, most important, B2O3 proved to be highly abrasive to rocket nozzles. Reactions carried out during this investigation, however, showed that boron hydrides exhibit unusual reactivity.
Because boron and hydrogen have almost identical electronegativities, the reactions of boron hydrides are dictated by minor differences in the distribution of electron density in a given compound. In general, two distinct types of reaction are observed: electron-rich species such as the BH4− ion are reductants, whereas electron-deficient species such as B2H6 act as oxidants.
Example \(\PageIndex{1}\)
For each reaction, explain why the given products form.
- B2H6(g) + 3O2(g) → B2O3(s) + 3H2O(l)
- BCl3(l) + 3H2O(l) → B(OH)3(aq) + 3HCl(aq)
- \(\mathrm{2BI_3(s)}+\mathrm{3H_2(g)}\xrightarrow{\Delta}\frac{1}{6}\mathrm{B_{12}(s)}+\mathrm{6HI(g)}\)
Given: balanced chemical equations
Asked for: why the given products form
Strategy:
Classify the type of reaction. Using periodic trends in atomic properties, thermodynamics, and kinetics, explain why the reaction products form.
Solution
- Molecular oxygen is an oxidant. If the other reactant is a potential reductant, we expect that a redox reaction will occur. Although B2H6 contains boron in its highest oxidation state (+3), it also contains hydrogen in the −1 oxidation state (the hydride ion). Because hydride is a strong reductant, a redox reaction will probably occur. We expect that H− will be oxidized to H+ and O2 will be reduced to O2−, but what are the actual products? A reasonable guess is B2O3 and H2O, both stable compounds.
- Neither BCl3 nor water is a powerful oxidant or reductant, so a redox reaction is unlikely; a hydrolysis reaction is more probable. Nonmetal halides are acidic and react with water to form a solution of the hydrohalic acid and a nonmetal oxide or hydroxide. In this case, the most probable boron-containing product is boric acid [B(OH)3].
- We normally expect a boron trihalide to behave like a Lewis acid. In this case, however, the other reactant is elemental hydrogen, which usually acts as a reductant. The iodine atoms in BI3 are in the lowest accessible oxidation state (−1), and boron is in the +3 oxidation state. Consequently, we can write a redox reaction in which hydrogen is oxidized and boron is reduced. Because compounds of boron in lower oxidation states are rare, we expect that boron will be reduced to elemental boron. The other product of the reaction must therefore be HI.
Exercise \(\PageIndex{1}\)
Predict the products of the reactions and write a balanced chemical equation for each reaction.
- \(\mathrm{B_2H_6(g)}+\mathrm{H_2O(l)}\xrightarrow{\Delta}\)
- \(\mathrm{BBr_3(l)}+\mathrm{O_2(g)}\rightarrow\)
- \(\mathrm{B_2O_3(s)}+\mathrm{Ca(s)}\xrightarrow{\Delta}\)
Answer
- \(\mathrm{B_2H_6(g)}+\mathrm{H_2O(l)}\xrightarrow{\Delta}\mathrm{2B(OH)_3(s)}+\mathrm{6H_2(g)}\)
- \(\mathrm{BBr_3(l)}+\mathrm{O_2(g)}\rightarrow\textrm{no reaction}\)
- \(\mathrm{6B_2O_3(s)}+18\mathrm{Ca(s)}\xrightarrow{\Delta}\mathrm{B_{12}(s)}+\mathrm{18CaO(s)}\)
Reactions and Compounds of the Heavier Group 13 Elements
All four of the heavier group 13 elements (Al, Ga, In, and Tl) react readily with the halogens to form compounds with a 1:3 stoichiometry:
\[ 2M_{(s)} + 3X_{2(s,l,g)} \rightarrow 2MX_{3(s)} \text{ or } M_2X_6 \label{Eq10}\]
The reaction of Tl with iodine is an exception: although the product has the stoichiometry TlI3, it is not thallium(III) iodide, but rather a thallium(I) compound, the Tl+ salt of the triiodide ion (I3−). This compound forms because iodine is not a powerful enough oxidant to oxidize thallium to the +3 oxidation state.
Of the halides, only the fluorides exhibit behavior typical of an ionic compound: they have high melting points (>950°C) and low solubility in nonpolar solvents. In contrast, the trichorides, tribromides, and triiodides of aluminum, gallium, and indium, as well as TlCl3 and TlBr3, are more covalent in character and form halogen-bridged dimers (part (b) in Figure \(\PageIndex{4}\)). Although the structure of these dimers is similar to that of diborane (B2H6), the bonding can be described in terms of electron-pair bonds rather than the delocalized electron-deficient bonding found in diborane. Bridging halides are poor electron-pair donors, so the group 13 trihalides are potent Lewis acids that react readily with Lewis bases, such as amines, to form a Lewis acid–base adduct:
\[Al_2Cl_{6(soln)} + 2(CH_3)_3N_{(soln)} \rightarrow 2(CH_3)_3N:AlCl_{3(soln)} \label{Eq11}\]
In water, the halides of the group 13 metals hydrolyze to produce the metal hydroxide (\[M(OH)_3\)):
\[MX_{3(s)} + 3H_2O_{(l)} \rightarrow M(OH)_{3(s)} + 3HX_{(aq)} \label{Eq12}\]
In a related reaction, Al2(SO4)3 is used to clarify drinking water by the precipitation of hydrated Al(OH)3, which traps particulates. The halides of the heavier metals (In and Tl) are less reactive with water because of their lower charge-to-radius ratio. Instead of forming hydroxides, they dissolve to form the hydrated metal complex ions: [M(H2O)6]3+.
Of the group 13 halides, only the fluorides behave as typical ionic compounds.
Like boron (Equation \(\ref{Eq22.7}\)), all the heavier group 13 elements react with excess oxygen at elevated temperatures to give the trivalent oxide (M2O3), although Tl2O3 is unstable:
\[\mathrm{4M(s)}+\mathrm{3O_2(g)}\xrightarrow{\Delta}\mathrm{2M_2O_3(s)} \label{Eq13}\]
Aluminum oxide (Al2O3), also known as alumina, is a hard, high-melting-point, chemically inert insulator used as a ceramic and as an abrasive in sandpaper and toothpaste. Replacing a small number of Al3+ ions in crystalline alumina with Cr3+ ions forms the gemstone ruby, whereas replacing Al3+ with a mixture of Fe2+, Fe3+, and Ti4+ produces blue sapphires. The gallium oxide compound MgGa2O4 gives the brilliant green light familiar to anyone who has ever operated a xerographic copy machine. All the oxides dissolve in dilute acid, but Al2O3 and Ga2O3 are amphoteric, which is consistent with their location along the diagonal line of the periodic table, also dissolving in concentrated aqueous base to form solutions that contain M(OH)4− ions.
Group 13 trihalides are potent Lewis acids that react with Lewis bases to form a Lewis acid–base adduct.
Aluminum, gallium, and indium also react with the other group 16 elements (chalcogens) to form chalcogenides with the stoichiometry M2Y3. However, because Tl(III) is too strong an oxidant to form a stable compound with electron-rich anions such as S2−, Se2−, and Te2−, thallium forms only the thallium(I) chalcogenides with the stoichiometry Tl2Y. Only aluminum, like boron, reacts directly with N2 (at very high temperatures) to give AlN, which is used in transistors and microwave devices as a nontoxic heat sink because of its thermal stability; GaN and InN can be prepared using other methods. All the metals, again except Tl, also react with the heavier group 15 elements (pnicogens) to form the so-called III–V compounds, such as GaAs. These are semiconductors, whose electronic properties, such as their band gaps, differ from those that can be achieved using either pure or doped group 14 elements. For example, nitrogen- and phosphorus-doped gallium arsenide (GaAs1−x−yPxNy) is used in the displays of calculators and digital watches.
All group 13 oxides dissolve in dilute acid, but Al2O3 and Ga2O3 are amphoteric.
Unlike boron, the heavier group 13 elements do not react directly with hydrogen. Only the aluminum and gallium hydrides are known, but they must be prepared indirectly; AlH3 is an insoluble, polymeric solid that is rapidly decomposed by water, whereas GaH3 is unstable at room temperature.
Complexes of Group 13 Elements
Boron has a relatively limited tendency to form complexes, but aluminum, gallium, indium, and, to some extent, thallium form many complexes. Some of the simplest are the hydrated metal ions [M(H2O)63+], which are relatively strong Brønsted–Lowry acids that can lose a proton to form the M(H2O)5(OH)2+ ion:
\[[M(H_2O)_6]^{3+}_{(aq)} \rightarrow M(H_2O)_5(OH)^{2+}_{(aq)} + H^+_{(aq)} \label{Eq14}\]

Group 13 metal ions also form stable complexes with species that contain two or more negatively charged groups, such as the oxalate ion. The stability of such complexes increases as the number of coordinating groups provided by the ligand increases.
Example \(\PageIndex{2}\)
For each reaction, explain why the given products form.
- \(\mathrm{2Al(s)} + \mathrm{Fe_2O_3(s)}\xrightarrow{\Delta}\mathrm{2Fe(l)} + \mathrm{Al_2O_3(s)}\)
- \(\mathrm{2Ga(s)} + \mathrm{6H_2O(l)}+ \mathrm{2OH^-(aq)}\xrightarrow{\Delta}\mathrm{3H_2(g)} + \mathrm{2Ga(OH)^-_4(aq)}\)
- \(\mathrm{In_2Cl_6(s)}\xrightarrow{\mathrm{H_2O(l)}}\mathrm{2In^{3+}(aq)}+\mathrm{6Cl^-(aq)}\)
Given: balanced chemical equations
Asked for: why the given products form
Strategy:
Classify the type of reaction. Using periodic trends in atomic properties, thermodynamics, and kinetics, explain why the reaction products form.
Solution
- Aluminum is an active metal and a powerful reductant, and Fe2O3 contains Fe(III), a potential oxidant. Hence a redox reaction is probable, producing metallic Fe and Al2O3. Because Al is a main group element that lies above Fe, which is a transition element, it should be a more active metal than Fe. Thus the reaction should proceed to the right. In fact, this is the thermite reaction, which is so vigorous that it produces molten Fe and can be used for welding.
- Gallium lies immediately below aluminum in the periodic table and is amphoteric, so it will dissolve in either acid or base to produce hydrogen gas. Because gallium is similar to aluminum in many of its properties, we predict that gallium will dissolve in the strong base.
- The metallic character of the group 13 elements increases with increasing atomic number. Indium trichloride should therefore behave like a typical metal halide, dissolving in water to form the hydrated cation.
Exercise \(\PageIndex{2}\)
Predict the products of the reactions and write a balanced chemical equation for each reaction.
- LiH(s) + Al2Cl6(soln)→
- Al2O3(s) + OH−(aq)→
- Al(s) + N2(g) \(\xrightarrow{\Delta}\)
- Ga2Cl6(soln) + Cl−(soln)→
Answer
- 8LiH(s) + Al2Cl6(soln)→2LiAlH4(soln) + 6LiCl(s)
- Al2O3(s) + 2OH−(aq) + 3H2O(l) → 2Al(OH)4−(aq)
- 2Al(s) + N2(g) \(\xrightarrow{\Delta}\) 2AlN(s)
- Ga2Cl6(soln) + 2Cl−(soln) → 2GaCl4−(soln)
Summary
Compounds of the group 13 elements with oxygen are thermodynamically stable. Many of the anomalous properties of the group 13 elements can be explained by the increase in Zeff moving down the group. Isolation of the group 13 elements requires a large amount of energy because compounds of the group 13 elements with oxygen are thermodynamically stable. Boron behaves chemically like a nonmetal, whereas its heavier congeners exhibit metallic behavior. Many of the inconsistencies observed in the properties of the group 13 elements can be explained by the increase in Zeff that arises from poor shielding of the nuclear charge by the filled (n − 1)d10 and (n − 2)f14 subshells. Instead of forming a metallic lattice with delocalized valence electrons, boron forms unique aggregates that contain multicenter bonds, including metal borides, in which boron is bonded to other boron atoms to form three-dimensional networks or clusters with regular geometric structures. All neutral compounds of the group 13 elements are electron deficient and behave like Lewis acids. The trivalent halides of the heavier elements form halogen-bridged dimers that contain electron-pair bonds, rather than the delocalized electron-deficient bonds characteristic of diborane. Their oxides dissolve in dilute acid, although the oxides of aluminum and gallium are amphoteric. None of the group 13 elements reacts directly with hydrogen, and the stability of the hydrides prepared by other routes decreases as we go down the group. In contrast to boron, the heavier group 13 elements form a large number of complexes in the +3 oxidation state.