4.1: Introduction
- Page ID
- 190024
\( \newcommand{\vecs}[1]{\overset { \scriptstyle \rightharpoonup} {\mathbf{#1}} } \)
\( \newcommand{\vecd}[1]{\overset{-\!-\!\rightharpoonup}{\vphantom{a}\smash {#1}}} \)
\( \newcommand{\id}{\mathrm{id}}\) \( \newcommand{\Span}{\mathrm{span}}\)
( \newcommand{\kernel}{\mathrm{null}\,}\) \( \newcommand{\range}{\mathrm{range}\,}\)
\( \newcommand{\RealPart}{\mathrm{Re}}\) \( \newcommand{\ImaginaryPart}{\mathrm{Im}}\)
\( \newcommand{\Argument}{\mathrm{Arg}}\) \( \newcommand{\norm}[1]{\| #1 \|}\)
\( \newcommand{\inner}[2]{\langle #1, #2 \rangle}\)
\( \newcommand{\Span}{\mathrm{span}}\)
\( \newcommand{\id}{\mathrm{id}}\)
\( \newcommand{\Span}{\mathrm{span}}\)
\( \newcommand{\kernel}{\mathrm{null}\,}\)
\( \newcommand{\range}{\mathrm{range}\,}\)
\( \newcommand{\RealPart}{\mathrm{Re}}\)
\( \newcommand{\ImaginaryPart}{\mathrm{Im}}\)
\( \newcommand{\Argument}{\mathrm{Arg}}\)
\( \newcommand{\norm}[1]{\| #1 \|}\)
\( \newcommand{\inner}[2]{\langle #1, #2 \rangle}\)
\( \newcommand{\Span}{\mathrm{span}}\) \( \newcommand{\AA}{\unicode[.8,0]{x212B}}\)
\( \newcommand{\vectorA}[1]{\vec{#1}} % arrow\)
\( \newcommand{\vectorAt}[1]{\vec{\text{#1}}} % arrow\)
\( \newcommand{\vectorB}[1]{\overset { \scriptstyle \rightharpoonup} {\mathbf{#1}} } \)
\( \newcommand{\vectorC}[1]{\textbf{#1}} \)
\( \newcommand{\vectorD}[1]{\overrightarrow{#1}} \)
\( \newcommand{\vectorDt}[1]{\overrightarrow{\text{#1}}} \)
\( \newcommand{\vectE}[1]{\overset{-\!-\!\rightharpoonup}{\vphantom{a}\smash{\mathbf {#1}}}} \)
\( \newcommand{\vecs}[1]{\overset { \scriptstyle \rightharpoonup} {\mathbf{#1}} } \)
\( \newcommand{\vecd}[1]{\overset{-\!-\!\rightharpoonup}{\vphantom{a}\smash {#1}}} \)
\(\newcommand{\avec}{\mathbf a}\) \(\newcommand{\bvec}{\mathbf b}\) \(\newcommand{\cvec}{\mathbf c}\) \(\newcommand{\dvec}{\mathbf d}\) \(\newcommand{\dtil}{\widetilde{\mathbf d}}\) \(\newcommand{\evec}{\mathbf e}\) \(\newcommand{\fvec}{\mathbf f}\) \(\newcommand{\nvec}{\mathbf n}\) \(\newcommand{\pvec}{\mathbf p}\) \(\newcommand{\qvec}{\mathbf q}\) \(\newcommand{\svec}{\mathbf s}\) \(\newcommand{\tvec}{\mathbf t}\) \(\newcommand{\uvec}{\mathbf u}\) \(\newcommand{\vvec}{\mathbf v}\) \(\newcommand{\wvec}{\mathbf w}\) \(\newcommand{\xvec}{\mathbf x}\) \(\newcommand{\yvec}{\mathbf y}\) \(\newcommand{\zvec}{\mathbf z}\) \(\newcommand{\rvec}{\mathbf r}\) \(\newcommand{\mvec}{\mathbf m}\) \(\newcommand{\zerovec}{\mathbf 0}\) \(\newcommand{\onevec}{\mathbf 1}\) \(\newcommand{\real}{\mathbb R}\) \(\newcommand{\twovec}[2]{\left[\begin{array}{r}#1 \\ #2 \end{array}\right]}\) \(\newcommand{\ctwovec}[2]{\left[\begin{array}{c}#1 \\ #2 \end{array}\right]}\) \(\newcommand{\threevec}[3]{\left[\begin{array}{r}#1 \\ #2 \\ #3 \end{array}\right]}\) \(\newcommand{\cthreevec}[3]{\left[\begin{array}{c}#1 \\ #2 \\ #3 \end{array}\right]}\) \(\newcommand{\fourvec}[4]{\left[\begin{array}{r}#1 \\ #2 \\ #3 \\ #4 \end{array}\right]}\) \(\newcommand{\cfourvec}[4]{\left[\begin{array}{c}#1 \\ #2 \\ #3 \\ #4 \end{array}\right]}\) \(\newcommand{\fivevec}[5]{\left[\begin{array}{r}#1 \\ #2 \\ #3 \\ #4 \\ #5 \\ \end{array}\right]}\) \(\newcommand{\cfivevec}[5]{\left[\begin{array}{c}#1 \\ #2 \\ #3 \\ #4 \\ #5 \\ \end{array}\right]}\) \(\newcommand{\mattwo}[4]{\left[\begin{array}{rr}#1 \amp #2 \\ #3 \amp #4 \\ \end{array}\right]}\) \(\newcommand{\laspan}[1]{\text{Span}\{#1\}}\) \(\newcommand{\bcal}{\cal B}\) \(\newcommand{\ccal}{\cal C}\) \(\newcommand{\scal}{\cal S}\) \(\newcommand{\wcal}{\cal W}\) \(\newcommand{\ecal}{\cal E}\) \(\newcommand{\coords}[2]{\left\{#1\right\}_{#2}}\) \(\newcommand{\gray}[1]{\color{gray}{#1}}\) \(\newcommand{\lgray}[1]{\color{lightgray}{#1}}\) \(\newcommand{\rank}{\operatorname{rank}}\) \(\newcommand{\row}{\text{Row}}\) \(\newcommand{\col}{\text{Col}}\) \(\renewcommand{\row}{\text{Row}}\) \(\newcommand{\nul}{\text{Nul}}\) \(\newcommand{\var}{\text{Var}}\) \(\newcommand{\corr}{\text{corr}}\) \(\newcommand{\len}[1]{\left|#1\right|}\) \(\newcommand{\bbar}{\overline{\bvec}}\) \(\newcommand{\bhat}{\widehat{\bvec}}\) \(\newcommand{\bperp}{\bvec^\perp}\) \(\newcommand{\xhat}{\widehat{\xvec}}\) \(\newcommand{\vhat}{\widehat{\vvec}}\) \(\newcommand{\uhat}{\widehat{\uvec}}\) \(\newcommand{\what}{\widehat{\wvec}}\) \(\newcommand{\Sighat}{\widehat{\Sigma}}\) \(\newcommand{\lt}{<}\) \(\newcommand{\gt}{>}\) \(\newcommand{\amp}{&}\) \(\definecolor{fillinmathshade}{gray}{0.9}\)The earth is an oxygen-rich place. Elemental oxygen, O2, makes up about 20% of the earth's atmosphere by weight. Oxygen atoms, bound in water, make up about 85% of the hydrosphere (the earth's oceans, rivers and lakes). Oxygen atoms also comprise about 45% of the lithosphere (the earth's crust), mostly bound in the form of silicates, aluminosilicates and carbonates.
In this chapter, we will focus on elemental oxygen and the ways nature has evolved to make use of O2 as a reactant. Dioxygen has a double nature, like Dr. Jeckyll and Mr. Hyde. It is necessary for life, providing a driving force for basic metabolic processes. That driving force ultimately comes from the exergonic conversion of O2 into water. However, nature has also had to evolve mechanisms to protect itself against reactive oxygen species, which would otherwise cause permanent damage to biomolecules. Those reactive oxygen species arise from the reduction of atmospheric oxygen. They also have dual roles, acting as important cell signalling molecules as well as dangers to the cell, and so their regulation is crucial in biochemistry.
Although da Vinci noted in the 1400's that air contained several components, one of which could support combustion, in a sense oxygen was not "discovered" until the turbulent 1770's. It was first isolated from air in experiments performed by Carl Wilhelm Scheele, a German pharmaceutical chemist (although his homeland of Pomerania was at that time part of Sweden, which extended to the south shores of the Baltic sea). This discovery was soon followed by an independent one by the English scientist and fire-and-brimstone preacher, Joseph Priestley. However, early research on oxygen is often associated with Antoine Lavoisier, whose experiments built upon those of Scheele and Priestley and are generally considered to mark the beginnings of modern chemistry.
These men did not lead long, peaceful lives. Priestley was forced to emigrate to Pennsylvania because of his troublemaking ways, Lavoisier was unfortunately executed during the reign of terror following the French Revolution, and Scheele's habit of tasting his experiments led to an early death from kidney failure. In contrast, a fourth scientist who is closely associated with oxygen, Henry Cavendish, died a wealthy (but remarkably reclusive) man of nearly eighty, having achieved some fame in England for his discoveries. Cavendish established the exact proportion of oxygen and nitrogen in the air, demonstrated in an impressively explosive way how oxygen and his other discovery, hydrogen, could be combined to make water, and even found time to accurately weigh the planet Earth.
Oxygen, present at the birth of modern chemistry, continued to make appearances in the development of the field. In the 1840's, Michael Faraday, the great English chemist and physicist, first demonstrated the O2 is paramagnetic, meaning it is attracted to a magnetic field. Sixty years later, American chemist and physicist Robert Mulliken explained this property through "molecular orbital theory" (sometimes called Hund-Mulliken theory after its developers). This explanation should be familiar to college-level chemistry students.
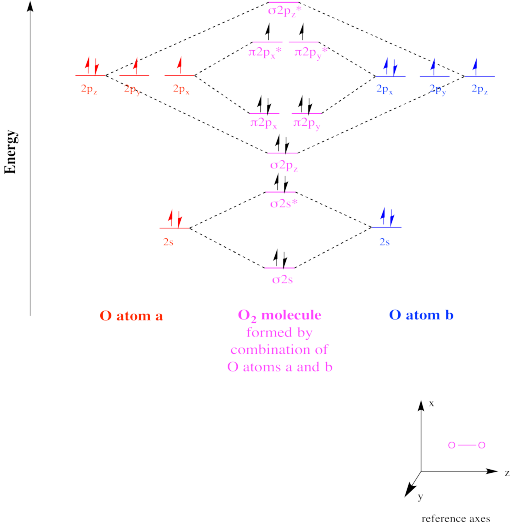
The molecular orbital interaction diagram for O2 shows how two oxygen atoms would combine under symmetry constraints to produce new orbitals in an O2 molecule. The net four electrons in bonding levels (i.e. at lower energy than they were in the atoms, as opposed to antibonding levels, which are at higher energy than they were in the separate atoms) suggests two bonds, since a bond between two atoms generally has two electrons. This prediction is also predicted by simple Lewis theory (i.e. that's what you would draw in a Lewis structure).

However, in contrast to the Lewis structure, in which all of the electrons in O2 are paired, Mulliken's MO picture suggests that there are two unpaired electrons. Because species with unpaired electrons are attracted to magnetic fields, this picture provides a compelling explanation for the paramagnetic behaviour of elemental oxygen.
This species is called a triplet state. A triplet is a state in which there are two net spins in the molecule, which has consequences in spectroscopy. If there were no net spins (i.e. they are all paired), the state would be described as a singlet. If there were one net spin (all other spins paired), it would be called a doublet.
If O2 accepts one electron, it forms a species called "superoxide" ion.
- Draw the MO diagram for the species formed when O2 accepts one electron.
- Is this new species a singlet, doublet or triplet?
- How would you describe the net number of bonds (the bond order) in this species?
- Draw a Lewis structure for this species.
- Answer
-
If O2 accepts two electrons, it forms a species called "peroxide" ion.
- Draw the MO diagram for the species formed when O2 accepts two electrons.
- Is this new species a singlet, doublet or triplet?
- How would you describe the net number of bonds (the bond order) in this species?
- Draw a Lewis structure for this species.
- Answer
-
In the 1930's, other forms of O2 were discovered by spectroscopists. By monitoring the interaction of light with oxygen, they detected evidence for different electronic states, meaning different arrangements of electrons. W. H. J. Childs and R. Mecke observed a singlet state in which two electrons appeared to be unpaired but with opposite spins. According to Hund's rule, this a higher energy state, because the electrons in singly-occupied orbitals should have the same spin. This state is described by spectroscopists as the 1Σg+ state. The usual form of O2 is described as the 3Σg- state. The 1Σg+ excited state is about 25 kcal/mol higher in energy than the 3Σg- ground state.

Gerhard Herzberg, the German-Canadian Nobel Laureate, observed a second singlet state. In this case, the electrons are spin-paired within the same orbital, also in violation of Hund's rule, since an empty orbital exists at the same energy level. This form of O2 is described as the 1Δg state. It is about 35 kcal/mol higher in energy than the ground state.
Although they are clearly higher in energy than the usual ground state, these two singlet states can arise when an oxygen molecule absorbs extra energy. Singlet oxygen undergoes reactions that are different from the usual triplet oxygen and it is considered a reactive oxygen species; it is linked to LDL cholesterol oxidation and cardiovascular damage, for example.
There is one last historical development in oxygen chemistry that we'll look at here. In the 1960's, Estonian-American chemist Lauri Vaska demonstrated the reversible binding of O2 to a coordination complex, trans-[Ir(CO)Cl(PPh3)2]. This observation was made when Vaska was a research scientist at the Mellon Insitute, now part of Carnegie-Mellon University in Pittsburgh.
Show a mechanism, with arrows, for the binding of oxygen to Vaska's complex.
This last example is particularly important in this chapter. The binding of oxygen by a transition metal is, of course, an essential step for the survival of every vertebrate. However, haemoglobin is a very complicated molecule. At the time that Vaska made his discovery, there was a need to demonstrate things like how an oxygen molecule could bind to a metal. How did it attach? Did it bind though a lone pair or a pi bond? In what geometry did it bind? Was the Fe-O-O bond linear? Was it angular or bent?
Frequently in the field of bioinorganic chemistry, simple coordination complexes serve as models for the complicated tasks carried out by transition metals in biology. "Model compounds", as they are called, provide the kind of precedent upon which researchers start to build a picture of what is happenning within the complex environment of the cell. That kind of precedent may take the form of structural studies, in which particular coordination environments and geometries may indicate what may be possible in a metalloenzyme. It may also involve reactivity studies, in which a small complex believed to resemble the active site of a metalloprotein is shown to undergo reactions that may be relevant to biological processes.
Visit an overview of oxygen from a biochemical perspective at Henry Jakubowski's Biochemistry Online.