Electrochemical Cell Conventions
- Page ID
- 291
\( \newcommand{\vecs}[1]{\overset { \scriptstyle \rightharpoonup} {\mathbf{#1}} } \)
\( \newcommand{\vecd}[1]{\overset{-\!-\!\rightharpoonup}{\vphantom{a}\smash {#1}}} \)
\( \newcommand{\id}{\mathrm{id}}\) \( \newcommand{\Span}{\mathrm{span}}\)
( \newcommand{\kernel}{\mathrm{null}\,}\) \( \newcommand{\range}{\mathrm{range}\,}\)
\( \newcommand{\RealPart}{\mathrm{Re}}\) \( \newcommand{\ImaginaryPart}{\mathrm{Im}}\)
\( \newcommand{\Argument}{\mathrm{Arg}}\) \( \newcommand{\norm}[1]{\| #1 \|}\)
\( \newcommand{\inner}[2]{\langle #1, #2 \rangle}\)
\( \newcommand{\Span}{\mathrm{span}}\)
\( \newcommand{\id}{\mathrm{id}}\)
\( \newcommand{\Span}{\mathrm{span}}\)
\( \newcommand{\kernel}{\mathrm{null}\,}\)
\( \newcommand{\range}{\mathrm{range}\,}\)
\( \newcommand{\RealPart}{\mathrm{Re}}\)
\( \newcommand{\ImaginaryPart}{\mathrm{Im}}\)
\( \newcommand{\Argument}{\mathrm{Arg}}\)
\( \newcommand{\norm}[1]{\| #1 \|}\)
\( \newcommand{\inner}[2]{\langle #1, #2 \rangle}\)
\( \newcommand{\Span}{\mathrm{span}}\) \( \newcommand{\AA}{\unicode[.8,0]{x212B}}\)
\( \newcommand{\vectorA}[1]{\vec{#1}} % arrow\)
\( \newcommand{\vectorAt}[1]{\vec{\text{#1}}} % arrow\)
\( \newcommand{\vectorB}[1]{\overset { \scriptstyle \rightharpoonup} {\mathbf{#1}} } \)
\( \newcommand{\vectorC}[1]{\textbf{#1}} \)
\( \newcommand{\vectorD}[1]{\overrightarrow{#1}} \)
\( \newcommand{\vectorDt}[1]{\overrightarrow{\text{#1}}} \)
\( \newcommand{\vectE}[1]{\overset{-\!-\!\rightharpoonup}{\vphantom{a}\smash{\mathbf {#1}}}} \)
\( \newcommand{\vecs}[1]{\overset { \scriptstyle \rightharpoonup} {\mathbf{#1}} } \)
\( \newcommand{\vecd}[1]{\overset{-\!-\!\rightharpoonup}{\vphantom{a}\smash {#1}}} \)
\(\newcommand{\avec}{\mathbf a}\) \(\newcommand{\bvec}{\mathbf b}\) \(\newcommand{\cvec}{\mathbf c}\) \(\newcommand{\dvec}{\mathbf d}\) \(\newcommand{\dtil}{\widetilde{\mathbf d}}\) \(\newcommand{\evec}{\mathbf e}\) \(\newcommand{\fvec}{\mathbf f}\) \(\newcommand{\nvec}{\mathbf n}\) \(\newcommand{\pvec}{\mathbf p}\) \(\newcommand{\qvec}{\mathbf q}\) \(\newcommand{\svec}{\mathbf s}\) \(\newcommand{\tvec}{\mathbf t}\) \(\newcommand{\uvec}{\mathbf u}\) \(\newcommand{\vvec}{\mathbf v}\) \(\newcommand{\wvec}{\mathbf w}\) \(\newcommand{\xvec}{\mathbf x}\) \(\newcommand{\yvec}{\mathbf y}\) \(\newcommand{\zvec}{\mathbf z}\) \(\newcommand{\rvec}{\mathbf r}\) \(\newcommand{\mvec}{\mathbf m}\) \(\newcommand{\zerovec}{\mathbf 0}\) \(\newcommand{\onevec}{\mathbf 1}\) \(\newcommand{\real}{\mathbb R}\) \(\newcommand{\twovec}[2]{\left[\begin{array}{r}#1 \\ #2 \end{array}\right]}\) \(\newcommand{\ctwovec}[2]{\left[\begin{array}{c}#1 \\ #2 \end{array}\right]}\) \(\newcommand{\threevec}[3]{\left[\begin{array}{r}#1 \\ #2 \\ #3 \end{array}\right]}\) \(\newcommand{\cthreevec}[3]{\left[\begin{array}{c}#1 \\ #2 \\ #3 \end{array}\right]}\) \(\newcommand{\fourvec}[4]{\left[\begin{array}{r}#1 \\ #2 \\ #3 \\ #4 \end{array}\right]}\) \(\newcommand{\cfourvec}[4]{\left[\begin{array}{c}#1 \\ #2 \\ #3 \\ #4 \end{array}\right]}\) \(\newcommand{\fivevec}[5]{\left[\begin{array}{r}#1 \\ #2 \\ #3 \\ #4 \\ #5 \\ \end{array}\right]}\) \(\newcommand{\cfivevec}[5]{\left[\begin{array}{c}#1 \\ #2 \\ #3 \\ #4 \\ #5 \\ \end{array}\right]}\) \(\newcommand{\mattwo}[4]{\left[\begin{array}{rr}#1 \amp #2 \\ #3 \amp #4 \\ \end{array}\right]}\) \(\newcommand{\laspan}[1]{\text{Span}\{#1\}}\) \(\newcommand{\bcal}{\cal B}\) \(\newcommand{\ccal}{\cal C}\) \(\newcommand{\scal}{\cal S}\) \(\newcommand{\wcal}{\cal W}\) \(\newcommand{\ecal}{\cal E}\) \(\newcommand{\coords}[2]{\left\{#1\right\}_{#2}}\) \(\newcommand{\gray}[1]{\color{gray}{#1}}\) \(\newcommand{\lgray}[1]{\color{lightgray}{#1}}\) \(\newcommand{\rank}{\operatorname{rank}}\) \(\newcommand{\row}{\text{Row}}\) \(\newcommand{\col}{\text{Col}}\) \(\renewcommand{\row}{\text{Row}}\) \(\newcommand{\nul}{\text{Nul}}\) \(\newcommand{\var}{\text{Var}}\) \(\newcommand{\corr}{\text{corr}}\) \(\newcommand{\len}[1]{\left|#1\right|}\) \(\newcommand{\bbar}{\overline{\bvec}}\) \(\newcommand{\bhat}{\widehat{\bvec}}\) \(\newcommand{\bperp}{\bvec^\perp}\) \(\newcommand{\xhat}{\widehat{\xvec}}\) \(\newcommand{\vhat}{\widehat{\vvec}}\) \(\newcommand{\uhat}{\widehat{\uvec}}\) \(\newcommand{\what}{\widehat{\wvec}}\) \(\newcommand{\Sighat}{\widehat{\Sigma}}\) \(\newcommand{\lt}{<}\) \(\newcommand{\gt}{>}\) \(\newcommand{\amp}{&}\) \(\definecolor{fillinmathshade}{gray}{0.9}\)Using chemical reactions to produce electricity is now a priority for many researchers. Being able to adequately use chemical reactions as a source of power would greatly help our environmental pollution problems. In this section of electrochemistry, we will be learning how to use chemical reactions to produce this clean electricity and even use electricity to generate chemical reactions. In order to induce a flow of electric charges, we place a strip of metal (the electrode) in a solution containing the same metal, which is in aqueous state. The combination of an electrode and its solution is called a half cell. Within the half cell, metals ions from the solution could gain electrons from the electrode and become metal atoms;or the metal atoms from the electrode could lose electrons and become metals ions in the solution.
Introduction
We use two different half cells to measure how readily electrons can flow from one electrode to another, and the device used for measurement is called a voltmeter. The voltmeter measures the cell potential, denoted by Ecell, (in units of Volts, 1 V=1 J/C), which is the potential difference between two half cells. The salt bridge allows the ions to flow from one half cell to another but prevents the flow of solutions.
As indicated in the diagram, the anode is the electrode where oxidation occurs; \(\ce{Cu}\) loses two electrons to form \(\ce{Cu^{+2}}\). The cathode is the electrode where reduction occurs; \(\ce{Ag^{+} (aq)}\) gains electron to become \(\ce{Ag(s)}\). As a convenient substitution for the drawing, we use a cell diagram to show the parts of an electrochemical cell. For example above, the cell notation is:
\[\underbrace{\ce{Cu(s) | Cu^{2+} (aq)}}_{\text{oxidation half-reaction}} \,||\, \underbrace{\ce{Ag^{+} (aq)| Ag(s)}}_{\text{reduction half-reaction}} \nonumber \]
Where we place the anode on the left and cathode on the right, "\(|\)" represents the boundary between the two phases, and "\(||\)" represents the salt bridge. There are two types of electrochemical cells:
A Galvanic Cell (aka Voltaic Cell) induces a spontaneous redox reaction to create a flow of electrical charges, or electricity. Non-rechargeable batteries are examples of Galvanic cells.
- A Reaction is spontaneous when the change in Gibb’s energy, \(∆G\) is negative.
- Electrons flow from the anode(negative since electrons are built up here) to the cathode (positive since it is gaining electrons).
An Electrolytic cell is one kind of battery that requires an outside electrical source to drive the non-spontaneous redox reaction. Rechargeable batteries act as Electrolytic cells when they are being recharged.
- A reaction is non-spontaneous when ∆G is > 0.
- Must supply electrons to the cathode to drive the reduction, so cathode is negative.
- Must remove electrons from the anode to drive the oxidation, so anode is positive.
Both Galvanic and Electrolytic cells contain:
- Two electrodes: the anode where oxidation occurs and the cathode where reduction occurs (note that Cathode does not mean +, and Anode does not mean -)
- Volt meter: measures the electric current. In galvanic cells, this shows how much voltage is produced and in electrolytic cells, this shows how much voltage is applied to the system.
- Electrolyte
- conducting medium
- has contact with electrodes
- usually in aqueous solution of ionic compounds
- Salt Bridge
- joins the two halves of the electrochemical cell
- filled with a salt solution or gel
- keeps the solution separate
- Completes the circuit
Basic Terminology
Electrochemical cells use a vast amount of terminology. Here is a brief definition of some of the more common terms:
- Voltage: The potential difference between two half cells, also the amount of energy that drives a reaction. Voltage is an intensive property (amount of voltage does matter).
- Current: The flow of electric charges (in units of electrons per second). It is an extensive property (amount of current does matter). NOTE: High voltage does not mean high current.
- Primary Battery: non-rechargeable batteries. AA, AAA, etc.
- Secondary Battery: Rechargeable batteries. Lithium, cell phone batteries, etc.
- Tertiary Battery also called Fuel cells. Although not always considered as batteries, these often require a constant flow of reactants.
Galvanic Cell (aka Voltaic Cells)
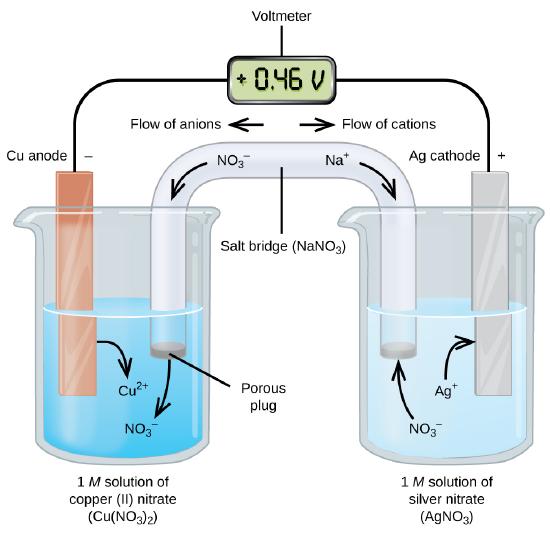
A galvanic cell produces an electrical charge from the flow of electrons. The electrons move due to the redox reaction. As we can see in Figure \(\PageIndex{1}\), \(\ce{Cu(s)}\) oxidizes to \(\ce{Cu^{2+}(aq)}\), while \(\ce{Ag^{+}(s)}\) reduces to \(\ce{Ag(s)}\). The cell notation for this cell is:
\[\underbrace{\ce{Cu (s) | Cu^{2+} (aq)}}_{\text{oxidation half-reaction}} \,||\, \underbrace{\ce{Ag^{+} (aq)| Ag(s)}}_{\text{reduction half-reaction}} \nonumber \]
To understand the cell, solve the redox equation.
First, split the reaction into two half reactions, with the same elements paired with one another.
\[\underbrace{\ce{Cu(s) -> Cu^{+2}(aq)}}_{\text{Oxidation reaction occurs at the Anode}} \nonumber \]
\[\underbrace{\ce{Ag^{+}(aq) -> Ag(s)}}_{\text{Reduction reaction occurs at the Cathode}} \nonumber \]
Next, we balance the two equations.
\[\text{Oxidation}: \ce{Cu(s) -> Cu^{2+}(aq) + 2e^{-} (Anode)} \nonumber \]
\[\text{Reduction}: \ce{e^{-} + Ag^{+}(aq) → Ag(s) (Cathode)} \nonumber \]
Finally, we recombine the two equations.
\[\ce{Cu(s) + 2Ag^{+}(aq) -> Cu^{2+}(aq) + 2Ag(s)} \nonumber \]
This is a spontaneous reaction that releases energy so this system does work on the surroundings.
Galvanic cells are quite common. A, AA, AAA, D, C, etc. batteries are all galvanic cells. Any non-rechargeable battery that does not depend on an outside electrical source is a Galvanic cell.
Electrolytic Cell
An electrolytic cell is a cell which requires an outside electrical source to initiate the redox reaction. The process of how electric energy drives the non-spontaneous reaction is called electrolysis. Whereas the galvanic cell used a redox reaction to make electrons flow, the electrolytic cell uses electron movement (in the source of electricity) to cause the redox reaction. In an electrolytic cell, electrons are forced to flow in the opposite direction. Since the direction is reversed of the voltaic cell, the E0cell for electrolytic cell is negative. Also, in order to force the electrons to flow in the opposite direction, the electromotive force that connects the two electrode-the battery must be larger than the magnitude of \(E^o_{cell}\). This additional requirement of voltage is called overpotential.
Reaction in Figure \(\PageIndex{1}\) can be switched by applying a voltage
\[\underbrace{\ce{Ag(s) -> Ag^{+}(aq) + e^{-}}}_{\text{Oxidation reaction occurs at the Anode}} \nonumber \]
\[\underbrace{\ce{Cu^{+2}(aq) + 2e^{-} -> Cu(s)}}_{\text{Reduction reaction occurs at the Cathode}} \nonumber \]
with the opposite total reaction
\[\ce{Cu^{2+}(aq) + 2Ag(s) ->[\text{applied voltage}] Cu(s) + 2Ag^{+}(aq)} \nonumber \]
- Galvanic: turns chemical energy into electrical energy
- Electrolytic Cell: turns electrical energy into chemical energy
The most common form of Electrolytic cell is the rechargeable battery (cell phones, mp3's, etc) or electroplating. While the battery is being used in the device it is a galvanic cell function (using the redox energy to produce electricity). While the battery is charging it is an electrolytic cell function (using outside electricity to reverse the completed redox reaction).
References
- Petrucci, Harwood, Herring, and Madura. General Chemistry: Principles and Modern Applications: Ninth Edition. New Jersey: Pearson, 2007.
- Professor Delmar Larsen. Lecture 2, 3, and 6. Spring 2010
- Rieger, Philip. Electrochemistry. 2, Illustrated. Springer Us, 1994. 112-113. Print.
- Hamann, Carl, Andrew Hamnett, and Wolf Vielstich. Electrochemistry. 2, Illustrated. Vch Verlagsgesellschaft Mbh, 2007. 82. Print.