1: Electrochemical Cells (Experiment)
- Page ID
- 431937
Pre-lab Questions
- Why is a tungsten electrode used instead of an iron electrode for the fourth half-cell in Table \(\PageIndex{1}\)?
- What is the purpose of the salt bridge?
- Why is each half-cell measured against the same copper half-cell?
Introduction
The use of electrochemical cells to convert the Gibbs energy stored in the constituent half-reactions into electrical work is of enormous industrial as well as fundamental significance. We all use batteries; these are simply galvanic cells similar to those you will construct in this experiment. In the laboratory, a typical electrochemical cell has the following general construction:
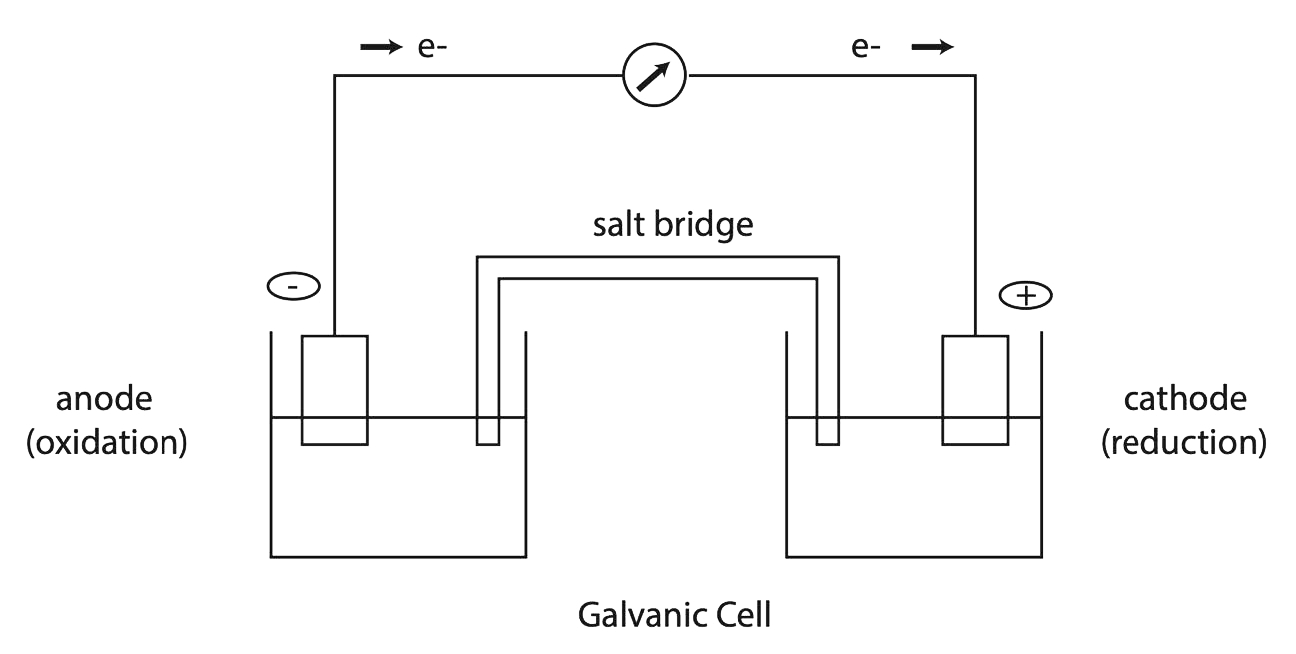
In Figure \(\PageIndex{1}\), there are two electrode-compartments, each of which contains an electrode and the constituents of the half-reaction. In many instances, the electrode is one of the chemical components of the half-reaction. For example, the half-reaction: \( \ce{Cu^{2+} + 2e^{-} \rightarrow Cu} \) involves the copper wire electrode directly. In other cases, however, the electrode does not participate in the chemistry of the half-reaction but merely provides an inert conducting surface on which the electron exchange occurs. For example, the half-reaction: \( \ce{Fe^{3+} + e^{-} \rightarrow Fe^{2+}} \) is studied with a \( \ce{Pt} \) or \( \ce{C} \) electrode rather than an iron electrode. This is because on an iron wire, both \( \ce{Fe^{3+} + 3e^{-} \rightarrow Fe} \) and \( \ce{Fe^{2+} + 2e^{-} \rightarrow Fe} \) could occur, rather than the \( \ce{Fe^{3+}/Fe^{2+}} \) reaction that is of interest.
A permeable barrier must separate the two electrode compartments. The barrier allows some ions to migrate between the two compartments to maintain electrical neutrality but does not allow the entire contents of the compartments to mix. A glass tube is often used, which is filled with a gel saturated with a strong electrolyte such as \( \ce{KNO3} \). In this case, the \( \ce{K+} \) and \( \ce{NO_3^{-}} \) ions in this "salt bridge" carry the current between the cell compartments.
Electrochemical cells have both a magnitude for the measured voltage and a polarity. The polarity is determined by the flow of electrons from the red to the black lead and, therefore, is dependent on the reaction that occurs at each electrode. By convention, the red electrode is designated the positive electrode. The positive electrode is deficient in electrons; hence, a reduction must be taking place at that electrode. Conversely, a negative electrode has a surplus of electrons, and electrons are being released into it by an oxidative half-reaction. By definition, oxidation occurs at an anode. Hence, the (-) pole of an electrochemical cell is necessarily its anode, and the (+) pole is its cathode (where the reduction occurs).
Methodology
In this experiment, you will construct and measure the voltage of electrochemical cells that involve the half-reactions (in alphabetical order): \( \ce{Cu^{2+} + 2e^{-} \rightarrow Cu} \) and \( \ce{Fe^{3+} + e^{-} \rightarrow Fe^{2+}} \). You will be able to determine the relative positions of these half-reactions in a Table of Standard Potentials from these results. You will also confirm that the potentials of half-reactions are concentration dependent. As you know, to calculate the voltages of non-standard cells (i.e. not 1 M and 1 atm), you need to use the Nernst equation, which can be found in your textbook. You will assume the temperature is room temperature (25°C). Read the chapter on Electrochemistry in your textbook as pre-laboratory preparation before beginning this experiment. One last but important note: the experiment is quite easy and short in terms of the actual collection of data; however, the write-up is difficult and requires a good understanding of electrochemistry. Students in the past have found it very helpful if they take the time to do each step slowly and try to understand what is happening as the experiment is performed. They also have said that it is critical to begin the write-up while they are in the laboratory in order to repeat steps that have become confusing or to get assistance from the TA or fellow students. You are strongly encouraged to follow this advice!
Safety
Solutions containing \( \ce{Cu} \) and \( \ce{Fe} \) must be disposed of in the proper waste container. The other solutions can go down the drain. Treat the voltmeters with care as they are very expensive. WEAR YOUR GOGGLES.
Experimental Procedures
Part A
-
Your class will be provided with a LabQuest Mini and a Voltage Probe with a red and black lead. There is an alligator clip at the end of each lead that can be used to hold the electrode. Note that in this setting, both a sign and a magnitude appear in the display. The sign is the relative polarity of the wire connected to the large plug. Also, obtain a salt bridge from the supply bench. Make sure the salt bridge is stored in deionized water when not in use so that it will not dry out. Using 50 mL beakers, prepare the half-cells shown below in test tubes. Measure out ~20 mL of each solution. The construction of these half-cells is as follows:
Beaker Solution Electrode 1 0.1 M \( \ce{Cu(NO3)2} \) \( \ce{Cu} \) 2 0.1 M \( \ce{Pb(NO3)2} \) \( \ce{Pb} \) 3 0.1 M \( \ce{Zn(NO3)2} \) \( \ce{Zn} \) 4* see below \( \ce{W} \) *The fourth beaker has, as the electrolyte, a solution that is made by mixing small equal volumes of 0.2 M FeSO4 and 0.2 M Fe(NO3)3 solutions. An inert tungsten plate will be used as the electrode for the half-cell.
- Measure the voltages of the 3 possible combinations of the four half-cells such that \( \ce{Cu} \) is always one of the half-cells. Make sure that the leads do not touch the solutions or faulty measurements will be made. The general apparatus is shown in Figure \(\PageIndex{2}\), but yours will look somewhat different. Be sure to record the polarity as well as the magnitude of each voltage and keep close track of which wire goes where. You should make an accurate drawing to keep track of all of this information. Remember to carefully rinse the salt bridge after each measurement to avoid contamination. Repeat this part of the experiment at least two times to be sure you are obtaining reasonably consistent values for the voltages of the six cells. It is important that you simply "play" with these cells and leads until they start to make sense. You can mix and match any other possible combination of cells (such as zinc vs lead or iron vs lead) to see the various potential differences.
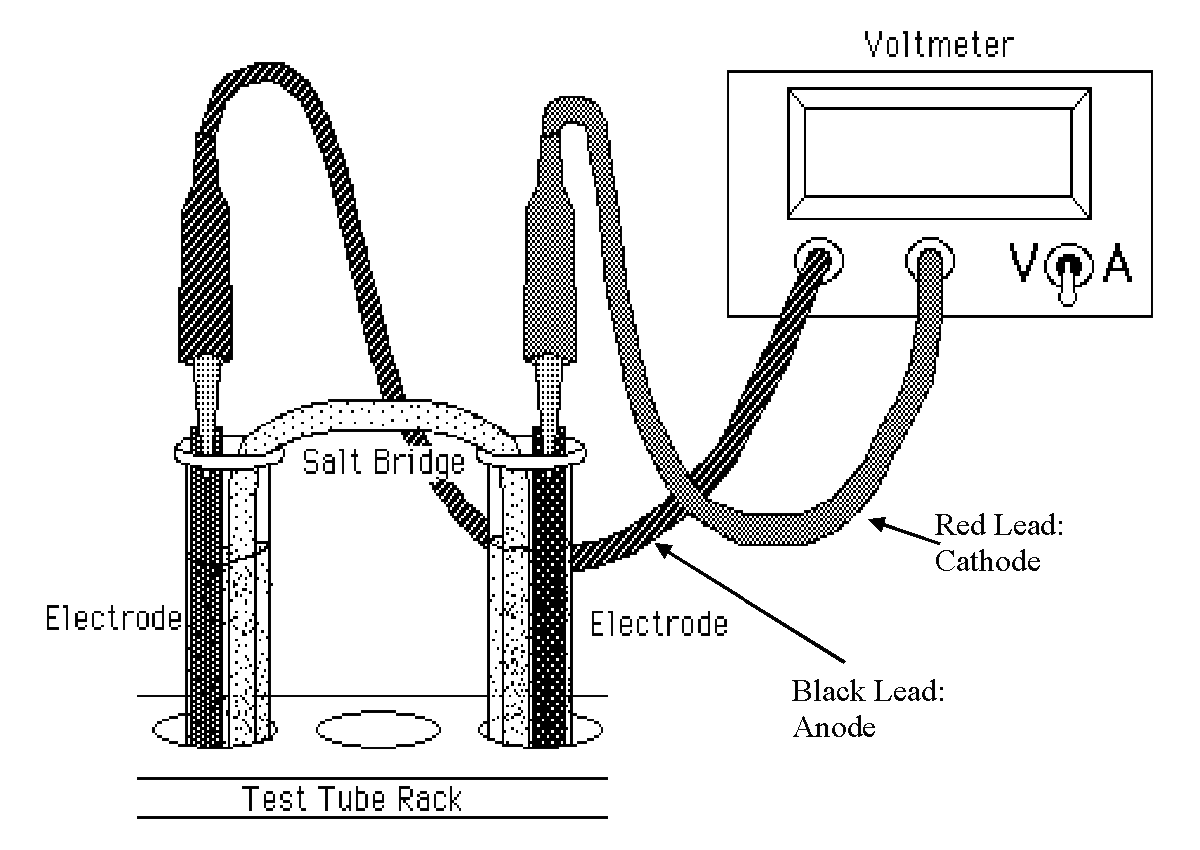
Part B
- Prepare a 0.01 M \( \ce{Cu(NO3)2} \) solution from the 0.1 M stock solution by dilution. This will be used for one half-cell. You will need to prepare a 0.02 M \( \ce{Fe(NO3)3} \) solution from the 0.2 M stock solution and a 0.02 M \( \ce{FeSO4} \) solution from the 0.2 M stock solution. Then by mixing equal volumes of the 0.2 M stock solutions with the 0.02 M solutions, you should make a solution that is 0.1 M \( \ce{Fe^{3+}} \) /0.01 M \( \ce{Fe^{2+}} \) and one that is 0.01 M \( \ce{Fe^{3+}} \) /0.1 M \( \ce{Fe^{2+}} \). These solutions should be placed into separate beakers. (The iron solution reacts with the oxygen in the air, so use the solutions immediately after mixing.)
- Using the following half-cells, measure the voltage of each against the \( \ce{Cu /Cu^{2+}} \) (0.1 M) half-cell. The half-cells to be used are: \( \ce{Cu / Cu^{2+}} \) (0.01 M); \( \ce{W / Fe^{3+}} \) (0.1 M), \( \ce{Fe^{2+}} \) (0.01 M); and \( \ce{W / Fe^{3+}} \) (0.01 M), \( \ce{Fe^{2+}} \) (0.1 M). Repeat this set of measurements until you have reasonably consistent voltages for the three cells. Again, simply "play" with the leads and half-cells until the voltages (magnitude and sign) make sense. (Reminder: the black lead is, by convention, the anode).
- Return all electrodes to the proper container. Be especially sure to return the tungsten plates, as they are the hardest to replace. Place all \( \ce{Cu} \) and \( \ce{Fe} \) containing solutions in the waste container labeled as "Cation Metal Waste" in the fume hood.
- Return the voltmeter to the front of the room and be sure that it is turned off.
Calculation
- Write the line diagram for each cell you measured, and write down the experimental voltage to the right of the diagram. Use the convention of the "anode on the left", and thus all voltages should be positive if the black lead was connected to the anode.
- Using your data, you will now construct a Standard Reduction Table. To do this, you will need a comparison cell, called a "standard." The usual "standard" is the hydrogen electrode, but this is very difficult to construct. The "standard" you have used is the \( \ce{Cu/Cu^{2+}} \) (0.1 M) half-cell. It is well known that the reduction potential of a \( \ce{Cu/Cu^{2+}} \) (1 M) half-cell has a value of 0.340 V (notice that this is at standard conditions, 1M solution). Using the reduction potential of copper at standard conditions (0.340 V), first, calculate the voltage of the \( \ce{Cu/Cu^{2+}} \) (0.1 M) half-cell using the Nernst equation.
-
Next, using your data determine the non-standard voltage of the other half-cells that you linked to the "standard" copper half-cell in the experiment in Step 2. This can be done by writing the half-reactions and simply adding or subtracting the voltage of your "standard" copper half-cell from the measured voltage of the complete cell. Remember that \[ E_{cell}=E_{cathode}-E_{anode} \] assuming that you have written the potentials as reduction potentials.
Finally, use the Nernst equation to calculate the reduction potential for the other half cells at standard conditions (1 M) you used in Step 2.
-
Now that you have calculated the voltage of each half-cell at standard conditions, create a conventional Standard Reduction Table for the four half-reactions you used in Step 2. Your table should include the 4 half reactions (written as reductions), your experimental reduction potential, and the accepted values listed in Appendix E of your textbook.
-
Step 4 of the procedure explores the concentration dependence of the half-cells. When you linked the two copper half-cells together, you measured the voltage for a copper-ion concentration cell. Using your newly prepared standard reduction table and the Nernst equation, calculate the theoretical value for the \( \ce{Cu} \) concentration cell. Compare this calculated value to the experimental value. The numerical agreement will not be perfect; however, the qualitative behavior should be correct.
-
You have measured a total of three \( \ce{Fe^{3+}/Fe^{2+}} \) cells with different concentrations of the oxidized and reduced forms of iron against the \( \ce{Cu /Cu^{2+}} \) (0.1 M) half-cell. Using your newly prepared standard reduction table and the Nernst equation, calculate the theoretical value for the various iron cells and compare these calculated values to the experimental values. Again, the agreement should be semi-quantitative.
Clean Up
Rinse all glassware with deionized water. Return all chemicals and equipment to the proper location. Rinse the bench with your sponge and water. All metal-containing solutions must be disposed of in the proper waste bottles.