Key Terms
Make certain that you can define, and use in context, the key terms below.
- anti periplanar
- deuterium isotope effect
- E2 reaction
- periplanar
- syn periplanar
Study Notes
An E2 reaction is a bimolecular elimination reaction; thus, two molecules are involved in the rate-limiting step. In this section, we are concerned with E2 reactions involving an alkyl halide and a base.
Use molecular models to assist you to understand the difference between syn periplanar and anti periplanar, and to appreciate why E2 eliminations are stereospecific.
Note that when deuterium is used the kinetic isotope effect (KIE) is referred to as the deuterium isotope effect. A C–H bond is about 5 kJ/mol weaker than a C–D bond. So if the rate-limiting step involves a breaking of this bond as it does at the E2 transition state there will be a substantial difference in reaction rates when comparing deuterated and non-deuterated analogues. Indeed, the reaction of 2-bromopropane with sodium ethoxide (NaOEt) is 6.7 times faster than its deuterated counterpart, providing evidence consistent with an E2 mechanism.
Introduction
E2, bimolecular elimination, was proposed in the 1920s by British chemist Christopher Kelk Ingold. E2 reactions are typically seen with secondary and tertiary alkyl halides, in the presence of a base (OH-, RO-, R2N-). For a primary halide to undergo an E2 reaction a strong sterically hindered base is usually required. The products of an E2 reaction follow Zaitsev's rule, the most substituted alkene is usually the major product.
E2 Mechanism
The E2 mechanism takes place in a single concerted step. The rate at which this mechanism occurs follows second order kinetics, and depends on the concentration of both the base and alkyl halide. The base removes a hydrogen from a carbon adjacent to the carbon with the leaving group. The electrons of the broken H-C bond move to form the pi bond of the neutral product alkene. In doing this, the C-X bond is broken causing the removal of the leaving group. Overall during this reaction an electron pair is transferred from the base to the leaving group.

E2 Reaction Transition State
Because the E2 reaction is predicted to follow a concerted mechanism only one transition state is expected in its energy coordinate diagram.
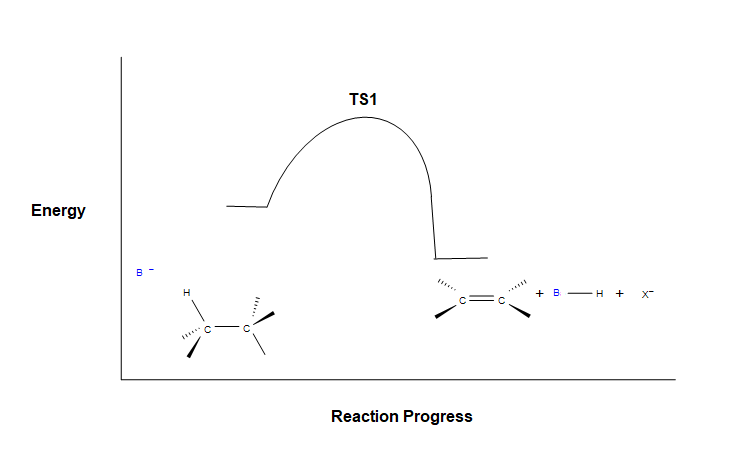
As the base begins to remove a hydrogen adjacent to the leaving group an H-B is starting to form and an H-C bond is in the process of breaking. Simultaneously, a C=C pi bond forms and the C-X bond breaks. During this reaction a set of electrons and a negative charge are transferred from the base to the leaving group. This is represented by a partial negative charge being present on both the base and the leaving group in the transition state.
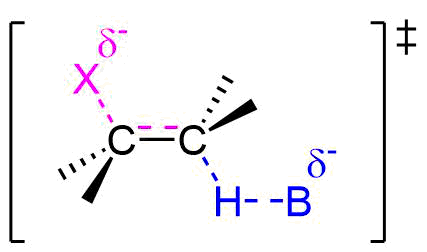
Evidence for the E2 Mechanism and Transition State
Kinetic studies of E2 reactions show that they are second order overall and follow the rate law: rate = k[RX][Base]. This data is consistent with the predicted bimolecular E2 mechanism that includes both the alkyl halide and the base participating in the mechanism's rate-determining step.
Further evidence for the predicted mechanism for the E2 reaction was provided by experiments involving the deuterium isotope effect (DIE). The deuterium isotope effect (DIE) is a phenomenon associated with molecules which have had hydrogen (H) atoms isotopically substituted with deuterium (D) atoms, exhibiting different reaction rates. The bond dissociation energy for C-D bonds is about 5 kJ/mol stronger than the bond dissociation energy of C-H bonds. This difference in energy results in a reduction in the rate of reaction, if the deuterium replacement occurs at a site of bond breaking in the rate determining step of a reaction. So if the rate-limiting step involves a breaking of this bond, as it does at the E2 transition state, there will be a substantial decrease in reaction rates when comparing deuterated and non-deuterated analogues. Indeed, the reaction of 2-bromopropane with sodium ethoxide (NaOEt) is 6.7 times faster than its deuterated counterpart, providing evidence that the C-H bond is broken during the rate determining step of the E2 mechanism. This is consistent with the predicted mechanism of the E2 reaction consisting of a single concerted step. If the rate-determining step was the leaving group bond breaking first (as in an E1 reaction) the deuterium substitution would not have caused an observable change in reaction rate.
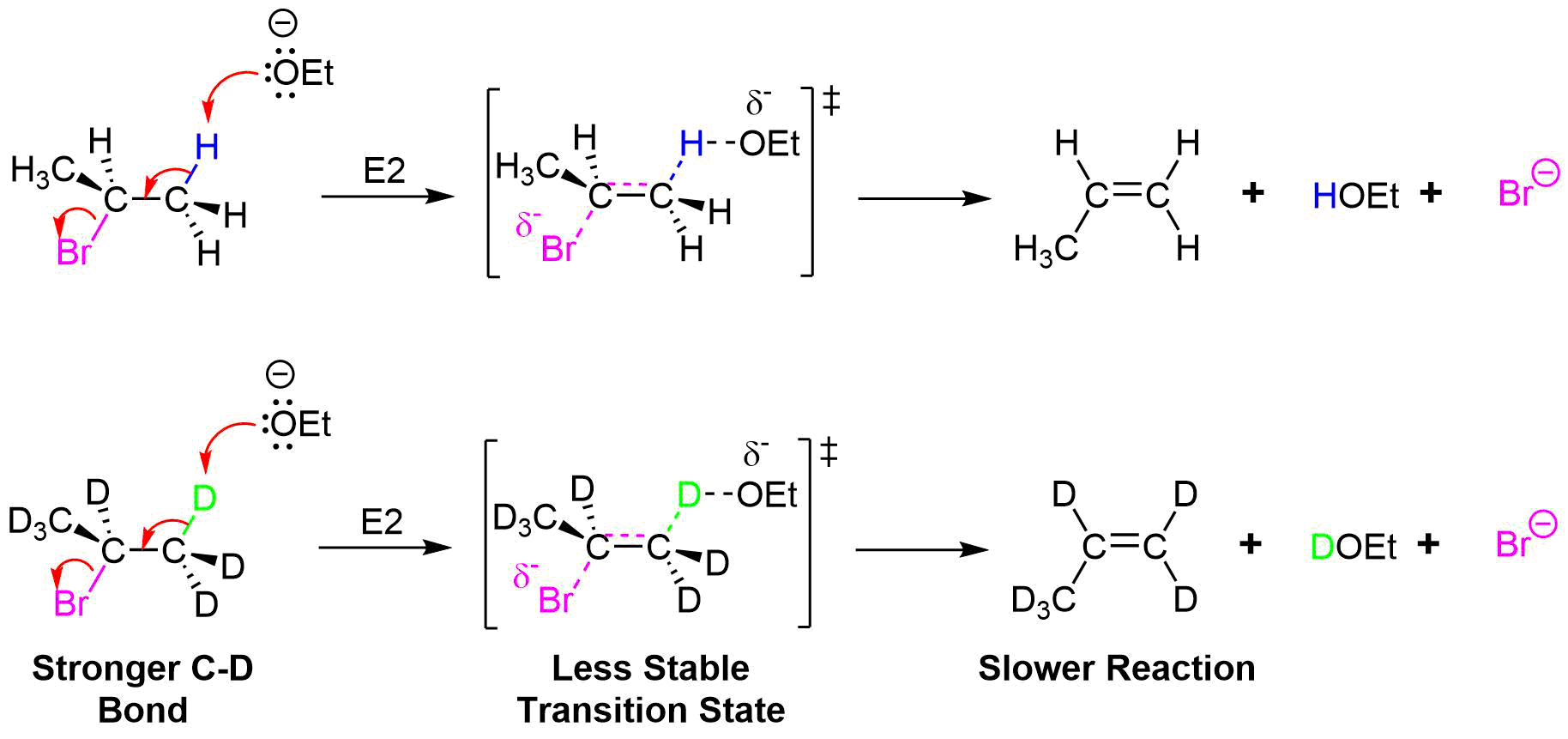
Yet another piece of evidence which supports the E2 mechanism comes when considering the stereochemistry of the elimination products. In the transition state of the E2 mechanism there are two carbons rehybridization from sp3 to sp2 while forming a π-bond. For good orbital overlap to occur during this change all the atoms involved in the reaction, the two carbons, the hydrogen, and the leaving group need to all lie in the same plane or periplanar. There are two possible ways this geometry can be achieved: syn-periplanar where the hydrogen and the leaving group are on the same side of the C-C bond and anti-periplanar where the hydrogen and the leaving group are on opposite sides of the C-C bond. To obtain a syn-periplanar geometry the substituents attached to the C-C bond must adopt an energetically unfavorable eclipsed conformation. The energy barrier to syn-orientation is such that syn-elimination is rarely observed in E2 reactions. E2 eliminations typically take place from the anti-periplanar conformation, as this is the most stable conformation due to the substituents attached to the C-C bond being staggered.

When viewing the transition state of the E2 mechanism when in the anti-periplanar conformation, the two sp3 hybridized orbitals making up the C-H and C-X sigma bonds are in the same plane. When these two carbons rehybridization from sp3 to sp2, the p orbitals forming the π-bond will also have good overlap.

The stereochemical consequences of reactant molecules obtaining anti-periplanar geometry prior to E2 reactions has been observed in numerous experiments and provides further evidence of the proposed mechanism. For example, (2S, 3R)-2-bromo-2,3-diphenylbutane only forms (Z)-2,3-diphenyl-2-butene as a product of E2 elimination. To form the Z alkene isomer, the starting material must obtain the anti-periplanar geometry preferred for E2 reactions.
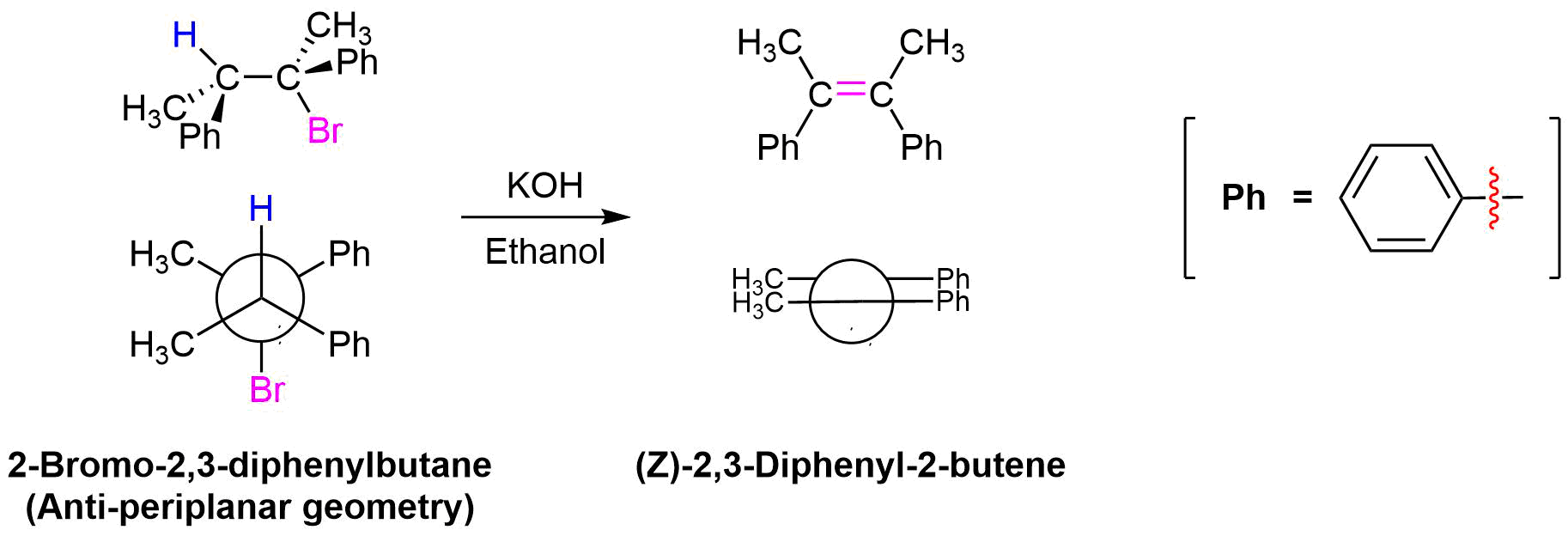
No isomeric E alkene product is formed because the starting materials would need to obtain a staggard, syn-periplanar geometry. The syn-periplanar geometry would be transferred to the transition state making it higher in energy and harder to form. The fact that only the Z alkene isomer forms provides further evidence that the E2 mechanism takes place by a single bimolecular step.
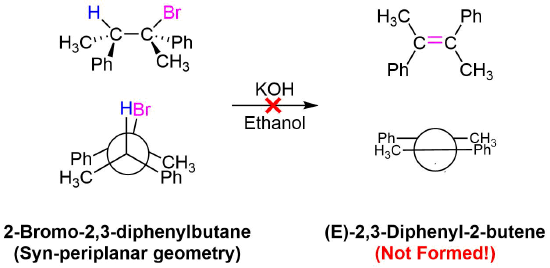
Predicting the Stereochemical Product of E2 Reactions
E2 reactions have the requirements of a leaving group and a hydrogen on a carbon adjacent to the leaving group carbon. For non-ringed compounds, the C-C can usually undergo free rotation to place the C-X and C-H bonds anti-periplaner to each other. Stereochemical considerations usually come into play when both of the carbons in the C-C bond are chiral. When these carbons are achiral but contain the other requirements for an E2 reaction, products lacking stereochemistry are usually produced.
When both of the carbons in the C-C bond are chiral, one carbon will have a leaving group and the other carbon will have a single hydrogen. To consider the stereochemistry of an E2 product, start by creating a Newman projection looking down the C-C bond. Then rotate the C-C bond until the C-X and C-H bonds are in the anti position. The Newman projection now shows the relative positioning of the substituents on the C-C bond as they will appear in the double bond formed. It should be noted that if syn elimination was possible, a positional isomer of the double bond will be created. Consider the elimination products for (2R, 3R)-2-bromo-3-methylpentane.

First, locate hydrogens on carbons adjacent to the leaving group. The compound used in this example has two unique sets of hydrogens.

Because the adjacent hydrogen colored fuchsia is attached to a chiral carbon it require special consideration. Convert the structure into a Newman projection along the C-C bond that will for the alkene elimination product. The C-C bond can be view down either direction and still product the correct product.

Then rotate such the the adjacent H and the leaving group are in the anti position.

This Newman projection shows the relative positioning of the substituents on the double bond formed. Note, none of the Z isomer is formed because it would require syn orientation of the H and Br.

Lastly, consider the products made by other adjacent hydrogens and apply Zaitsev's rule to predict the preferred product.

Determining Cis and Trans Products in Elimination Reaction
Understanding that E2 reactions require an anti-periplanar geometry can explain cis and trans alkene isomers can form as products. This occurs as an important exception to the rule that stereoisomers only form as elimination products when both of the carbons in the C-C bond forming the alkene are chiral. When considering the E2 reaction products of (R)-2-butane, there are two groups of hydrogens on carbons adjacent to the leaving group a CH3 and a CH2.

The carbon in the CH2 group is achiral so both the H's appear to be equivalent. However, when the anti-periplanar geometry requirement is applied each hydrogen will produce its own unique product. Once the reactant is converted into a Newman projection it can be seen that the Br leaving group and the hydrogen colored blue are in the anti orientation and are ready to be eliminated. It should also be noted that the two CH3 groups are also in the anti position which gives them the least amount of steric interaction possible. Because the CH3 groups are on opposite sides of the Newman projection the CH3 groups will be on opposite sides of the alkene thus creating the product trans-2-butene. When the Newman projection is rotated such that the fuchsia colored hydrogen is anti to the Br leaving group, the two CH3 groups are now on the same. This orientation creates the isomer cis-2-butene. However, to obtain this orientation the two CH3 groups must overcome the steric strain associated with a gauche conformation. This makes the transition state leading the cis isomer higher in energy and more difficult to form. Because of this, the trans isomer will be the major product. It is clear that the two hydrogens of the CH2 group are not equivalent despite their not being attached to a chiral carbon.

After considering all the products made during this E2 reaction the preferred product can be determined. The cis and trans isomer both have two alkene substituents while the third product only has one. Of the cis and trans isomer, the trans isomer is expected to be easier to form. Zaitsev's rule says that trans-2-butene will be the major product of this reaction.

Exercise \(\PageIndex{1}\)
1) What is the product of the following molecule in an E2 reaction? What is the stereochemistry?

- Answer
-
The stereochemistry is (Z) for the reaction.

Contributors and Attributions