17.2: Electrolysis
- Page ID
- 49549
\( \newcommand{\vecs}[1]{\overset { \scriptstyle \rightharpoonup} {\mathbf{#1}} } \)
\( \newcommand{\vecd}[1]{\overset{-\!-\!\rightharpoonup}{\vphantom{a}\smash {#1}}} \)
\( \newcommand{\id}{\mathrm{id}}\) \( \newcommand{\Span}{\mathrm{span}}\)
( \newcommand{\kernel}{\mathrm{null}\,}\) \( \newcommand{\range}{\mathrm{range}\,}\)
\( \newcommand{\RealPart}{\mathrm{Re}}\) \( \newcommand{\ImaginaryPart}{\mathrm{Im}}\)
\( \newcommand{\Argument}{\mathrm{Arg}}\) \( \newcommand{\norm}[1]{\| #1 \|}\)
\( \newcommand{\inner}[2]{\langle #1, #2 \rangle}\)
\( \newcommand{\Span}{\mathrm{span}}\)
\( \newcommand{\id}{\mathrm{id}}\)
\( \newcommand{\Span}{\mathrm{span}}\)
\( \newcommand{\kernel}{\mathrm{null}\,}\)
\( \newcommand{\range}{\mathrm{range}\,}\)
\( \newcommand{\RealPart}{\mathrm{Re}}\)
\( \newcommand{\ImaginaryPart}{\mathrm{Im}}\)
\( \newcommand{\Argument}{\mathrm{Arg}}\)
\( \newcommand{\norm}[1]{\| #1 \|}\)
\( \newcommand{\inner}[2]{\langle #1, #2 \rangle}\)
\( \newcommand{\Span}{\mathrm{span}}\) \( \newcommand{\AA}{\unicode[.8,0]{x212B}}\)
\( \newcommand{\vectorA}[1]{\vec{#1}} % arrow\)
\( \newcommand{\vectorAt}[1]{\vec{\text{#1}}} % arrow\)
\( \newcommand{\vectorB}[1]{\overset { \scriptstyle \rightharpoonup} {\mathbf{#1}} } \)
\( \newcommand{\vectorC}[1]{\textbf{#1}} \)
\( \newcommand{\vectorD}[1]{\overrightarrow{#1}} \)
\( \newcommand{\vectorDt}[1]{\overrightarrow{\text{#1}}} \)
\( \newcommand{\vectE}[1]{\overset{-\!-\!\rightharpoonup}{\vphantom{a}\smash{\mathbf {#1}}}} \)
\( \newcommand{\vecs}[1]{\overset { \scriptstyle \rightharpoonup} {\mathbf{#1}} } \)
\( \newcommand{\vecd}[1]{\overset{-\!-\!\rightharpoonup}{\vphantom{a}\smash {#1}}} \)
\(\newcommand{\avec}{\mathbf a}\) \(\newcommand{\bvec}{\mathbf b}\) \(\newcommand{\cvec}{\mathbf c}\) \(\newcommand{\dvec}{\mathbf d}\) \(\newcommand{\dtil}{\widetilde{\mathbf d}}\) \(\newcommand{\evec}{\mathbf e}\) \(\newcommand{\fvec}{\mathbf f}\) \(\newcommand{\nvec}{\mathbf n}\) \(\newcommand{\pvec}{\mathbf p}\) \(\newcommand{\qvec}{\mathbf q}\) \(\newcommand{\svec}{\mathbf s}\) \(\newcommand{\tvec}{\mathbf t}\) \(\newcommand{\uvec}{\mathbf u}\) \(\newcommand{\vvec}{\mathbf v}\) \(\newcommand{\wvec}{\mathbf w}\) \(\newcommand{\xvec}{\mathbf x}\) \(\newcommand{\yvec}{\mathbf y}\) \(\newcommand{\zvec}{\mathbf z}\) \(\newcommand{\rvec}{\mathbf r}\) \(\newcommand{\mvec}{\mathbf m}\) \(\newcommand{\zerovec}{\mathbf 0}\) \(\newcommand{\onevec}{\mathbf 1}\) \(\newcommand{\real}{\mathbb R}\) \(\newcommand{\twovec}[2]{\left[\begin{array}{r}#1 \\ #2 \end{array}\right]}\) \(\newcommand{\ctwovec}[2]{\left[\begin{array}{c}#1 \\ #2 \end{array}\right]}\) \(\newcommand{\threevec}[3]{\left[\begin{array}{r}#1 \\ #2 \\ #3 \end{array}\right]}\) \(\newcommand{\cthreevec}[3]{\left[\begin{array}{c}#1 \\ #2 \\ #3 \end{array}\right]}\) \(\newcommand{\fourvec}[4]{\left[\begin{array}{r}#1 \\ #2 \\ #3 \\ #4 \end{array}\right]}\) \(\newcommand{\cfourvec}[4]{\left[\begin{array}{c}#1 \\ #2 \\ #3 \\ #4 \end{array}\right]}\) \(\newcommand{\fivevec}[5]{\left[\begin{array}{r}#1 \\ #2 \\ #3 \\ #4 \\ #5 \\ \end{array}\right]}\) \(\newcommand{\cfivevec}[5]{\left[\begin{array}{c}#1 \\ #2 \\ #3 \\ #4 \\ #5 \\ \end{array}\right]}\) \(\newcommand{\mattwo}[4]{\left[\begin{array}{rr}#1 \amp #2 \\ #3 \amp #4 \\ \end{array}\right]}\) \(\newcommand{\laspan}[1]{\text{Span}\{#1\}}\) \(\newcommand{\bcal}{\cal B}\) \(\newcommand{\ccal}{\cal C}\) \(\newcommand{\scal}{\cal S}\) \(\newcommand{\wcal}{\cal W}\) \(\newcommand{\ecal}{\cal E}\) \(\newcommand{\coords}[2]{\left\{#1\right\}_{#2}}\) \(\newcommand{\gray}[1]{\color{gray}{#1}}\) \(\newcommand{\lgray}[1]{\color{lightgray}{#1}}\) \(\newcommand{\rank}{\operatorname{rank}}\) \(\newcommand{\row}{\text{Row}}\) \(\newcommand{\col}{\text{Col}}\) \(\renewcommand{\row}{\text{Row}}\) \(\newcommand{\nul}{\text{Nul}}\) \(\newcommand{\var}{\text{Var}}\) \(\newcommand{\corr}{\text{corr}}\) \(\newcommand{\len}[1]{\left|#1\right|}\) \(\newcommand{\bbar}{\overline{\bvec}}\) \(\newcommand{\bhat}{\widehat{\bvec}}\) \(\newcommand{\bperp}{\bvec^\perp}\) \(\newcommand{\xhat}{\widehat{\xvec}}\) \(\newcommand{\vhat}{\widehat{\vvec}}\) \(\newcommand{\uhat}{\widehat{\uvec}}\) \(\newcommand{\what}{\widehat{\wvec}}\) \(\newcommand{\Sighat}{\widehat{\Sigma}}\) \(\newcommand{\lt}{<}\) \(\newcommand{\gt}{>}\) \(\newcommand{\amp}{&}\) \(\definecolor{fillinmathshade}{gray}{0.9}\)A typical electrolytic cell can be made as shown in Figure \(\PageIndex{1}\). Two electrical conductors (electrodes) are immersed in the liquid to be electrolyzed. These electrodes are often made of an inert material such as stainless steel, platinum, or graphite. The liquid to be electrolyzed must be able to conduct electricity, and so it is usually an aqueous solution of an electrolyte or a molten ionic compound. The electrodes are connected by wires to a battery or other source of direct current. This current source may be thought of as an “electron pump” which takes in electrons from one electrode and forces them out into the other electrode. The electrode from which electrons are removed becomes positively charged, while the electrode to which they are supplied has an excess of electrons and a negative charge.
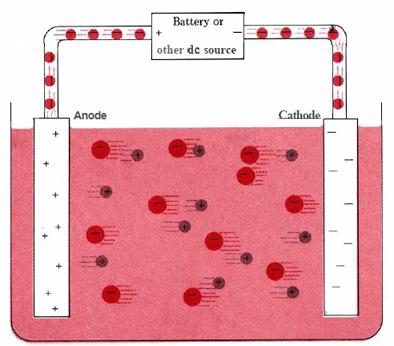
The negatively charged electrode will attract positive ions (cations) toward it from the solution. It can donate some of its excess electrons to such cations or to other species in the liquid being electrolyzed. Hence this electrode is in effect a reducing agent. In any electrochemical cell (electrolytic or galvanic) the electrode at which reduction occurs is called the cathode.
The positive electrode, on the other hand, will attract negative ions (anions) toward itself. This electrode can accept electrons from those negative ions or other species in the solution and hence behaves as an oxidizing agent. In any electrochemical cell the anode is the electrode at which oxidation occurs. An easy way to remember which electrode is which is that anode and oxidation begin with vowels while cathode and reduction begin with consonants.
The following video shows this process taking place in a neutral solution of water with some electrolytes present.
As an example of how electrolysis can cause a chemical reaction to occur, suppose we pass a direct electrical current through 1 M HCl. The H3O+ ions in this solution will be attracted to the cathode, and the \(\ce{Cl^{–}}\) ions will migrate toward the anode. At the cathode, H3O+ will be reduced to H2 gas according to the half-equation
\[\text{2H}^{+} + \text{2e}^{-} \rightarrow \text{H}_2\label{1} \]
(As seen in other sections, we shall write H+ instead of H3O+ in half-equations to save time.) At the anode, electrons will be accepted from \(\ce{Cl^{–}}\) ions, oxidizing them to Cl2:
\[\text{2Cl}^{-} \rightarrow \text{Cl}_2 + \text{2e}^{-} \label{2} \]
During electrolysis \(\ce{H2(g)}\) and \(\ce{Cl2(g)}\) bubble from the cathode and anode, respectively. The overall equation for the electrolysis is the sum of Eqsuations \ref{1} and \(\ref{2}\) :
\[\text{2H}^{+}(aq) + \text{2Cl}^{-}(aq) \rightarrow \text{H}_2(g) + \text{Cl}_2(g)\label{3} \]
or
\[\text{2H}_3\text{O}^{+}(aq) + \text{2Cl}^{-}(aq) \rightarrow \text{H}_2(g) + \text{Cl}_2(g) + \text{2H}_2\text{O}(l) \nonumber \]
The net reaction in Equation \ref{3} is the reverse of the spontaneous combination of \(\ce{H2(g)}\) with C\(\ce{Cl2(g)}\) to form \(\ce{HCl(aq)}\). Such a result is true of electrolysis in general: electrical current supplied from outside the system causes a non-spontaneous chemical reaction to occur.
Although electrolysis always reverses a spontaneous redox reaction, the result of a given electrolysis may not always be the reaction we want. In an aqueous solution, for example, there are always a great many water molecules in the vicinity of both the anode and cathode. These water molecules can donate electrons to the anode or accept electrons from the cathode just as anions or cations can. Consequently the electrolysis may oxidize and/or reduce water instead of causing the dissolved electrolyte to react. An example of this problem is electrolysis of lithium fluoride, \(\ce{LiF}\). We might expect reduction of \(\ce{Li^{+}}\) at the cathode and oxidation of \(\ce{F^{–}}\) at the anode, according to the half-equations
\[\text{Li}^{+}(aq) + \text{e}^{-} \rightarrow \text{Li}(s)\label{5} \]
\[\text{2F}^{-}(aq) \rightarrow \text{F}_2(g) + \text{2e}^{-} \nonumber \]
However, \(\ce{Li^{+}}\) is a very poor electron acceptor, and so it is very difficult to force Equation \ref{5} to occur. Consequently, excess electrons from the cathode are accepted by water molecules instead:
\[\text{2H}_2\text{O}(l) + \text{2e}^{-} \rightarrow \text{2OH}^{-}(aq) + \text{H}_2(g)\label{7} \]
A similar situation arises at the anode. F– ions are extremely weak reducing agents—much weaker than H2O molecules—so the half-equation is
\[\text{2H}_2\text{O}(l) \rightarrow \text{O}_2(g) + \text{4H}^{+}(aq) + \text{4e}^{-}\label{8} \]
The overall equation can be obtained by multiplying Equation \(\ref{7}\) by 2, adding it to Equation \(\ref{8}\) and combining H+ with OH– to form H2O:
\[\text{2H}_2\text{O}(l) \rightarrow \text{2H}_2(g) + \text{O}_2(g) \nonumber \]
The following video shows the electrolysis of water taking place, using sulfuric acid as a bridge to allow for the transfer of charge. After the electrolysis is complete, the identities of the gases formed are verified using burning splint tests.
Thus this electrolysis reverses the spontaneous combination of H2 and O2 to form H2O. In discussing redox reactions we mention several oxidizing agents, such as which are strong enough to oxidize H2O. At the same time we describe reducing agents which are strong enough to reduce H2O such as the alkali metals and the heavier alkaline earths. As a general rule such substances cannot be produced by electrolysis of aqueous solutions because H2O is oxidized or reduced instead. Substances which undergo spontaneous redox reaction with H2O are usually produced by electrolysis of molten salts or in some other solvent. There are some exceptions to this rule, however, because some electrode reactions are slower than others. Using Table 11.5, for example, we would predict that H2O is a better reducing agent than \(\ce{Cl^{–}}\).
Hence we would expect O2, not Cl2, to be produced by electrolysis of 1 M HCl, in contradiction of Equation \(\ref{1}\). It turns out that O2 is produced more slowly than Cl2, and the latter bubbles out of solution before the H2O can be oxidized. For this reason Table 1 found in the Redox Couples section cannot always be used to predict what will happen in an electrolysis.