Introduction To Electrochemical Sensors
- Page ID
- 283196
What’s a sensor?
The most basic definition of a sensor is an object that detects signals from its surrounding environment and converts it to meaningful or quantifiable information. Your eyes, ears, and nose are all different types of sensors that help you navigate your surroundings on a day-to-day basis by detecting and processing light, sound, and smell/taste. In chemistry, sensors serve a similar purpose: their job is to translate the reactions and conditions in a given environment into useful data for analysis—and just like your nose, eyes, and ears, different chemical sensors gather distinct sets of information that may be combined for a more complete picture of a given chemical environment. In this module, we cover the basics of electrochemical sensors, gas sensors, and biosensors.
ELECTROCHEMICAL SENSORS
Electrochemical sensors are made up of three essential components: a receptor that binds the sample, the sample or analyte, and a transducer to convert the reaction into a measurable electrical signal. In the case of electrochemical sensors, the electrode acts as the transducer.6
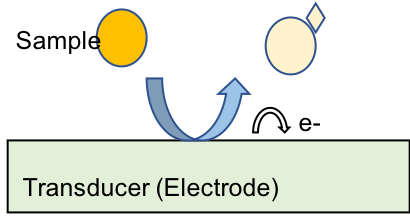
Figure 1: Schematic illustrating the basic components of an electrochemical sensor.
In most electrochemical sensors, an electrode surface is used as the site of the reaction.6 The electrode will either oxidize or reduce the analyte of interest. The current that is produced from the reaction is monitored and used to calculate important data such as concentrations from the sample.6
For instance, the nitric oxide (NO) sensor below, is a common electrochemical sensor. NO is an important vasodilator, and monitoring its levels becomes crucial in the diagnostics of cardiovascular complications. The sensor monitors the oxidation of NO as it occurs on the electrode surface. In many instances of electrochemical sensors, the electrode surface can be modified with catalysts, membranes, or other metals to make the electrode more sensitive and/or more selective toward the analyte (in the instance below, the Glassy Carbon (GC) electrode is modified with Cobalt (IV) Oxide and Platinum).

The direct electrooxidation of NO in solutions follows a 3 step reaction. The Δ E for the overall process is +0.5V vs. a Ag/AgCl reference electrode.
\[\ce{NO \rightarrow NO+ + e-} \tag{1}\]
\[\ce{NO+ + OH- \rightarrow HNO2} \tag{2}\]
\[\ce{HNO2 + H2O \rightarrow NO3- + 2e- + 3H+} \tag{3}\]
The ΔE for the overall process is +0.5V vs. a Ag/AgCl reference electrode.
The reaction for the Ag/AgCl reference electrode is the following:
\[\ce{AgCl + e- ↔ Ag + Cl-} \hspace{30px} \mathrm{E^\circ+ 0.222V}\nonumber\]
GAS SENSORS
What is it?
Gas sensors detect chemicals in the gas phase. Gas chromatography and electrochemical gas sensors are common examples of this technique. Like other sensors, gas sensors also require a sample and receptor to acquire and analyze measurements1; a good example of this are semiconductor gas sensors, where a gas interacts with the metal oxide surface and the subsequent analysis is made on the basis of whether that gas is reduced or oxidized.1
How is it made and how does it work?
Depending on the sample being analyzed, different methods of detection using gas sensors may be implemented. Electrochemical gas sensors work in a way similar to other electrochemical sensors, except in this case gases diffuse through a membrane to be reduced or oxidized at an electrode.7 The oxidation/reduction measurements allow us to measure the samples of interest.7
As an example the Figaro company’s CO gas electrochemical sensors works on this simple premises of monitoring the oxidation or reduction of a gas on an electrode surface. It works as an amperometric (detections current/time) cell with two electrodes, the working (sensing) electrode and the counter (pseudo reference) electrode which are placed in between a layer of electrolyte (H+).
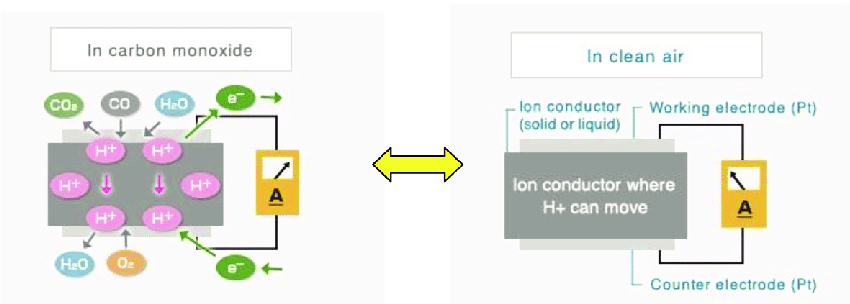
In this instance when CO gas reaches the working electrode, it is oxidized with the participation of a water molecules from the surrounding air (eq. 1).
\[\ce{CO + H2O \rightarrow CO2 + 2H+ + 2e-} \tag{eq. 1}\]
Since the working electrode is connected to the counter electrode, the protons (H+) formed by reaction (eq.1) will flow toward the counter electrode through the ion conductor and the electrons will move to the counter electrode through the external wiring. The protons and electrons will then react with oxygen from the environmental air (eq.2).
\[\ce{\frac{1}{2}O2 + 2H+ + 2e- \rightarrow H2O} \tag{eq. 2}\]
Thus the overall reactions holds:
\[\ce{CO + \frac{1}{2}O2 \rightarrow CO2} \tag{eq. 3}\]
In Table 1 below are the standard electrode potential for the above reaction (in reference to Reduced Hydrogen Electrode (RHE).
Reaction | Standard electrode potentials (V versus RHE*) |
---|---|
CO2 (g) + 2H+ + 2e- ⇌ CO (g) + H2O (l) | -0.11 |
H2O ⇌ ½O2 (g) + 2H+ + 2e- | +1.23 |
CO2 (g) ⇌ CO (g) + ½O2 (g) | ΔE = 1.34 V |
*RHE, reversible hydrogen electrode |
BIOSENSORS
Biosensors require the same elements as electrochemical sensors in order to function, with a slight modification. For biosensors, the elements required are a receptor that binds the sample, an interface for the sample to react, and a separate transducer. There are a number of different biosensors available, including amperometric biosensors, thermometric biosensors, optical biosensors, and immuno-biosensors, to list a few.
While there are a number of different biosensors available, at its core, a biosensor is a sensor that observes a specific reaction and quantifies it using a biological marker. For instance, enzymes, antibodies, and cell surface receptors are most often used to measure the amount of analyte in a given sample.5 To be effective, biosensors must meet certain criteria. They must be stable with little variation for a specific experiment, in addition to being both accurate and precise in the data they provide. It is also important that biosensors are specific, so that the experimenter can be sure of the parameters he or she measures.5 These are all important considerations when designing a biosensor.
Below are couple of schematics of the basics of Electrochemical biosensors.
How is it made and how does it work?
There are a variety of biosensors that are used to detect biological entities in different settings. As mentioned previously, biosensors require receptors, a surface for reaction, and a transducer; there are a number of ways to achieve this conformation. Electrochemical biosensors, for instance, have an electrode surface that acts as the reaction site and as the transducer. In this case, electrodes, an enzyme to catalyze a reaction, and the sample would make up the biosensor. For example, the electrode is placed in a solution containing an enzyme (the bioreceptor) specific to the analyte—let’s say in this case, glucose—and the change in potential is measured as the enzyme reacts with the glucose on the surface of the electrode, also known as the transducer. Depending on what electrochemical method is used, the current measured by the electrode or the change in potential caused by the redox reaction occurring on the electrode, is directly proportional to the concentration of a given sample.
Why is it important?
Biosensors are especially important in detecting the levels of different substances in our bodies. For instance, biosensors can detect nutrients or oxygen levels, which can then be compared to normal. Sensors can also be used to check for hormone levels.4 In the medical field, patients’ glucose and oxygen levels are often measured to help doctors diagnose and treat them. For instance, an individual might present with frequent urination, high levels of hunger, and fatigue. Without sensors and further testing, a number of diagnoses might be applicable to said patient (type these symptoms into WebMD and see for yourselves!). Glucose sensors, in particular, are important for confirming whether a patient is hyperglycemic (has high blood glucose), which may often be a result of diabetes.
Recently, glucose monitoring has seen the development of different methods of glucose detection. Today, glucose monitoring is possible through finger-pricking, which involves using the blood from the patient’s pricked finger to measure blood glucose via test strips and glucometers.4 First generation sensors, discussed below, used the glucose oxidase enzyme in conjunction with a platinum electrode to detect the amount of oxygen and hydrogen peroxide as the means to finding blood glucose concentrations.4
Example: Using Glucose Oxidase in Electrochemical Glucose sensors
You may be familiar with the process of oxidative phosphorylation, in which the electron carriers NADH and FADH2, generated in the Krebs cycle, are oxidized and their electrons are shuffled through complexes in order to produce water and ATP. The electron carriers NADH and FADH2 are also often seen acting as enzyme cofactors. A cofactor can be thought of as a part of an enzyme that is not made up of protein but is necessary for its function.
Glucose oxidase is an example of an enzyme that uses FAD as a cofactor to catalyze the oxidation of glucose into hydrodgen peroxide and gluconic acid.5 This enzyme falls into the category of oxidoreductase enzymes because it catalyzes a redox reaction, shown below:
Reaction 1:
\[\mathrm{GluOx(FAD) + Glucose \rightarrow GluOx(FADH_2)} + \textrm{Glucono-d-lactone}\nonumber\]
\[\ce{GluOx(FADH2) + O2 \rightarrow GluOx(FAD) + H2O2}\nonumber\]
In this reaction, FAD is reduced to FADH2 while glucose is oxidized to glucono-d-lactone and hydrogen peroxide. Glucose content in a given sample may be detected through an indirect (1) or direct (2) method.
- The enzyme can be placed on the surface of the electrode, and the amount of H2O2 produced can be monitored find the amount of glucose present. This method works in the presence of oxygen, because, as shown in Reaction 1, the FADH2 cofactor is oxidized by oxygen, which is reduced to H2O2.5 This method is considered to be indirect because as opposed to measuring the amount of glucose remaining or glucono-d-lactone produced by the reaction, we are measuring the by-product, H2O2.5
- Alternatively, in the absence of oxygen, an electron mediator can be used to quantify the reaction. In this method, the mediator is oxidized by the electrode and then reduced by FADH2. Since this reaction takes place in the absence of oxygen, H2O2 is not produced.5 This also gives a more direct method of measuring glucose in that only the oxidation of glucose, as opposed to the reduction of O2 to H2O2, is measured.5 In any case where a biological entity is used to facilitate the reaction, an adhesion method is applied to adhere the biological entity to the electrode material.
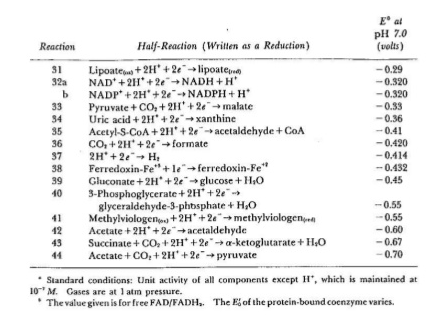
THE MARKET: WHAT’S A SENSOR WORTH?
Pat yourself on the back—you are a miracle that not only senses and processes information, but also understands it. Scientists around the world would give (almost) anything to develop a machine that can do even half of what you do every day, as accurately and precisely as you do it, without even having to think about it. That’s because sensing and processing information isn’t just important in passing your chemistry courses; in the medical field, it saves lives. It’s no surprise, then, that the biosensing market happens to be fast-growing and highly profitable.
Point of care (POC) testing comprises the largest share of the biosensor market as of 2016.2 POC tests are a set of diagnostic tests that are performed at the patient’s bedside. POC tests detect glucose, oxygen saturation, and antigens, among other species. These tests are particularly important in that they are both simple and quick, allowing physicians to streamline the treatment process. Each of these tests uses a different sensor or instrument to analyze a given sample. The market for glucose monitoring is also expected to see growth and potentially beat out POC by 2022.2 As diabetes continues to become more prevalent, the market for glucose sensors will continue to increase, as they play a crucial role in managing blood glucose levels.
Market analysis shows by 2020, the biosensor market is expected to be worth $21.2 billion USD, and $27.06 billion by 2022.3 In 2016, the industry was valued at $15.06 USD.3 Aside from POC testing and self-monitoring methods, sensors are important in research concerning genomics and proteomics. Both governments and private enterprises invest in this type invest in this research.3 The agricultural industry also contributes to the fast growth of the biosensor market, as biosensors have helped regulate pesticides.3
References
- Bárzana, E. (1995, January 01). Gas phase biosensors. Retrieved July 14, 2018, from https://link.springer.com/chapter/10.1007/BFb0102323
- Biosensors Market Size, Share & Trends Analysis Report By Application (Medical, Agriculture) By Technology (Thermal, Electrochemical, Optical) And Segment Forecasts, 2012 - 2020. (2017, March). Retrieved July 14, 2018, from https://www.grandviewresearch.com/industry-analysis/biosensors-market
- Biosensors Market worth 27.06 Billion USD by 2022. (n.d.). Retrieved July 14, 2018, from https://www.marketsandmarkets.com/PressReleases/biosensors.asp
- Bruen, D., Delaney, C., Florea, L., & Diamond, D. (2017). Glucose Sensing for Diabetes Monitoring: Recent Developments. Sensors,17(8), 1866. doi:10.3390/s17081866
- Grieshaber, D., Mackenzie, R., Vörös, J., & Reimhult, E. (2008). Electrochemical Biosensors - Sensor Principles and Architectures. Sensors,8(3), 1400-1458. doi:10.3390/s8031400
- Harper, A., & Anderson, M. R. (2010). Electrochemical Glucose Sensors—Developments Using Electrostatic Assembly and Carbon Nanotubes for Biosensor Construction. Sensors,10(9), 8248-8274. doi:10.3390/s100908248
- Kuretake, T., Kawahara, S., Motooka, M., & Uno, S. (2017). An Electrochemical Gas Biosensor Based on Enzymes Immobilized on Chromatography Paper for Ethanol Vapor Detection. Sensors (Basel, Switzerland), 17(2), 281. http://doi.org/10.3390/s17020281
Contributors and Attributions
- Asmira Alagic (admire.alagic@slu.edu) and Tara Tabibi, Saint Louis University
- Sourced from the Analytical Sciences Digital Library